DOI:
10.1039/C9QM00358D
(Research Article)
Mater. Chem. Front., 2019,
3, 2066-2071
Tunable aggregation-induced circularly polarized luminescence of chiral AIEgens via the regulation of mono-/di-substituents of molecules or nanostructures of self-assemblies†
Received
31st May 2019
, Accepted 24th July 2019
First published on 24th July 2019
Abstract
Four chiral AIE-active luminogens (AIEgens) containing tetraphenylethene (TPE) and one or two chiral glutamic moieties connected by thiourea were designed and synthesized. All AIEgens showed tunable aggregation-induced circularly polarized luminescence (AICPL) by adjusting the mono-/di-substituents of molecules or nanostructures of self-assemblies. The enantiotopic mono-substituted compounds (TPE-Glu) exhibited mirror-imaged AICPL signals with the maximal |glum| value of up to 0.02. Interestingly, the di-substituted cis- and trans-isomers showed reverse AICPL signals compared with those of mono-substituted ones. cis- and trans-isomers (TPE-DGlu) showed similar AICPL properties, whose signs of AICPL signals reversed while the water fraction (fw) was more than 80%. The SEM images of their self-assemblies indicated that the AICPL properties should have an intrinsic relation with their molecular structures or self-assembled nanostructures.
Introduction
Circularly polarized luminescence (CPL) refers to the phenomenon that chiral luminescent substances emit different ratios of left and right circularly polarized light, which has been attracting increasing attention due to its special properties and potential applications in various fields such as the investigation of the excited chiral molecular structures, 3D optical displays, information storage and processing, molecular switches, biological detection and probing.1 Up to now, different CPL systems have been reported, such as chiral organic conjugated molecules, polymers, organogels, and lanthanide complexes.2 However, most conventional organic conjugated materials suffer from aggregation-caused quenching (ACQ) effect in the solid state, which greatly limits their applications in optics.3 In 2001, Tang et al. first proposed aggregation-induced emission (AIE), which is used to describe the phenomenon that substances exhibit non-emissive behavior in a solution but have strong emission in the aggregated state.4 Therefore, the AIE property provides an approach to prepare CPL materials with high emission intensity in the solid state. Tang et al. originally combined AIE and CPL and prepared a compound by introducing chiral D-mannose moiety into an AIE-active silole in 2012, which exhibited no CPL signal in solution but high AICPL signal in the aggregated state.5 Since then, some other AIE-active CPL materials have been reported in different research fields.6 Among them, supramolecular assemblies formed through non-covalent interactions have received considerable attention because the chiral assemblies usually possess better CPL properties than those of chiral molecules and could transfer CPL-silence molecular chirality to CPL-on chiral nanostructures.7
It is well-known that molecular chirality has great influence on the optical activity of chiral compounds.8 However, the impact of cis- and trans-isomers on the optical activity of chiral molecules, particularly for the CPL property has rarely been studied.9 Although several studies have reported the influence of nanostructures on the AICPL property, it is still valuable to design chiral supramolecular systems that could be effectively regulated to obtain chiral materials with a tunable AICPL property.10
Herein, we designed and synthesized four compounds, which contained AIE-active tetraphenylethene (TPE) and one or two chiral glutamic moieties connected by thiourea. The enantiotopic mono-substituent compounds are labelled as TPE-L-Glu and TPE-D-Glu, while the di-substituent cis- and trans-isomers are marked as cis-TPE-L-DGlu and trans-TPE-L-DGlu, respectively. Both mono- and di-substituted compounds exhibited very weak emission and optical activity in THF, but showed obviously increasing fluorescence and tunable aggregation-induced circular dichroism (CD) and circularly polarized luminescence (CPL) signals with the addition of water, which demonstrated typical AIE and AICPL properties. The enantiotopic mono-substituted compounds (TPE-Glu) exhibited mirror-imaged AICPL signals, and the |glum| value could reach 0.02 when water fraction (fw) was at 50%. However, the di-substituted cis- and trans-isomers showed reverse AICPL signals compared with mono-substituted ones even when the same chiral glutamic moiety was used. cis-TPE-L-DGlu and trans-TPE-L-DGlu displayed similar optically active properties, whose aggregation-induced CD and CPL signals in more than 80% fw mixed solvents was very different from those in less than 80% fw mixed solvents. The SEM images of their self-assemblies indicated that AICPL properties could be related to both molecular structures and microstructures of the formed nanostructures (Scheme 1).
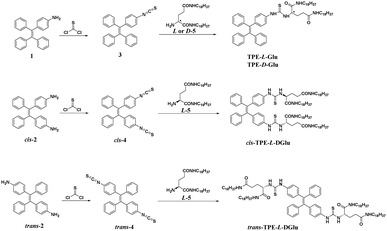 |
| Scheme 1 Synthetic procedures for the preparation of TPE-L-Glu, TPE-D-Glu, cis-TPE-L-DGlu and trans-TPE-L-DGlu. | |
Experimental
Preparation of compound 3
A mixture of compound 1 (0.50 g, 1.44 mmol) and triethylamine (0.6 mL, 4.32 mmol) was dissolved in 15 mL dichloromethane, followed by the addition of thiophosgene (0.15 mL, 1.96 mmol) in an ice bath. The reaction mixture was stirred for 4 h at ambient temperature. Then, 30 mL dichloromethane was added, and the mixture was washed with water and saturated brine, and then dried over anhydrous Na2SO4. After the solvent was removed under reduced pressure to obtain the residue, which was purified by silica gel column chromatography (hexane/ethyl acetate, 20/1, v/v) compound 3 was obtained as a yellow oil (0.49 g, 87%). 1H NMR (400 MHz, CDCl3) δ (ppm): 6.95 (d, J = 7.1 Hz, 2H), 7.00 (d, J = 4.2 Hz, 7H), 7.13–7.10 (m, 10H). HRMS (ESI+): m/z calcd [M+] 389.1238; found 389.1316.
Preparation of cis-4 and trans-4
The same procedure as that of compound 3 was followed for cis-4 and trans-4. The characterization of cis-4: 1H NMR (400 MHz, CDCl3) δ (ppm): 6.93 (d, J = 8.7 Hz, 6H), 6.96 (d, J = 9.4 Hz, 8H), 7.14 (s, 4H). HRMS (MALDI-TOF): m/z calcd [M+] 446.0911; found 446.0945. The characterization of trans-4: 1H NMR (400 MHz, CDCl3) δ (ppm): 6.95 (d, J = 7.1 Hz, 2H), 7.00 (d, J = 4.2 Hz, 6H), 7.11 (dd, J = 8.2, 2.5 Hz, 10H). HRMS (MALDI-TOF): m/z calcd [M+] 446.0911; found 446.0952.
Preparation of TPE-L-Glu and TPE-D-Glu
A mixture of compound 3 (0.50 g, 1.28 mmol) and compound L-5 (1.67 g, 2.57 mmol) was dissolved in 30 mL of anhydrous THF. The reaction mixture was stirred for 4 h at 80 °C. After cooling to room temperature, the reaction mixture was concentrated to afford the residue, which was purified via silica gel column chromatography (CH2Cl2/MeOH, 20/1, v/v) to afford TPE-L-Glu as a pale-white powder (1.05 g, 77%). 1H NMR (400 MHz, CDCl3) δ (ppm): 0.86 (t, J = 6.6 Hz, 6H), 1.24 (s, 60H), 1.48–1.49 (m, 4H), 3.17–3.26 (m, 7H), 6.98–7.09 (m, 19H). HRMS (MALDI-TOF): m/z calcd [M + Na+] 1061.7621; found 1061.7787. The prepared procedure of TPE-D-Glu was same as that for TPE-L-Glu. 1H NMR (400 MHz, CDCl3) δ (ppm): 0.81 (t, J = 6.0 Hz, 6H), 1.18 (s, 60H), 1.44 (d, J = 5.9 Hz, 4H), 3.12–3.19 (m, 5H), 4.86 (s, 1H), 5.75 (s, 1H), 6.90–7.04 (m, 19H). HRMS (MALDI-TOF): m/z calcd [M + Na+] 1061.7621; found 1061.7802.
Preparation of cis-TPE-L-DGlu and trans-TPE-L-DGlu
The same procedure as that of TPE-L-Glu was used for cis-TPE-L-DGlu and trans-TPE-L-DGlu. The characterization of cis-TPE-L-DGlu: 1H NMR (400 MHz, CDCl3) δ (ppm): 0.86 (t, J = 6.2 Hz, 12H), 1.23 (s, 120H), 1.35(t, J = 7.3 Hz, 8H), 1.42 (s, 8H), 3.08–3.21 (m, 12H), 6.82 (d, J = 8.5 Hz, 4H), 6.97 (d, J = 3.7 Hz, 4H), 7.00–7.05 (m, 10H). HRMS (MALDI-TOF): m/z calcd [M + Na+] 1768.3780; found 1768.3894. trans-TPE-L-DGlu: 1H NMR (400 MHz, CDCl3) δ (ppm): 0.86 (t, J = 6.6 Hz, 12H), 1.24 (s, 120H), 1.37–1.45 (m, 16H), 1.68 (d, J = 10.3 Hz, 4H), 3.11–3.17 (m, 4H), 6.86 (d, J = 8.3 Hz, 4H), 6.98 (d, J = 3.6 Hz, 4H), 7.01–7.10 (m, 10H). HRMS (MALDI-TOF): m/z calcd [M + Na+] 1768.3780; found 1768.3960.
Preparation of samples for optical measurements
Several stock solutions of the compounds were first prepared in THF (0.20 mM). Then, 10 μL of the stock solution was transferred to 2 mL THF/water mixture to afford the test solutions at the concentration of 10 μM. The optical measurements were then performed after 2 h.
Preparation of samples for SEM
Different THF/water mixtures of TPE-L-Glu, TPE-D-Glu, cis-TPE-L-DGlu and trans-TPE-L-DGlu with concentrations of 10 μM were prepared. Then, 10 μL of the solutions was dropped onto the surface of Si wafers, which were dried in vacuum after 2 h. The as-prepared samples were characterized by SEM.
Results and discussions
Synthesis of chiral AIEgens
Compounds 1, cis- and trans-2, L- and D-5 were prepared according to previously reported procedures.11 The initial compounds first reacted with thiophosgene to obtain mono- or di-isothiocyanato compounds 3 and cis- and trans-4. These intermediates then reacted with chiral glutamic derivatives (compound L- or D-5) to afford the four desired compounds. All the compounds were confirmed by NMR and HRMS (see ESI†).
Optical properties
The UV-vis spectra of these compounds were first obtained in a THF/water mixture, in which THF acted as a good solvent and water acted as a poor solvent. As shown in Fig. 1, the four compounds, TPE-L-Glu, TPE-D-Glu, cis-TPE-L-DGlu and trans-TPE-L-DGlu, exhibited similar absorption spectra with three absorption peaks located at about 250, 285 and 340 nm, respectively, which could result from the π–π* conjugation of the TPE cores. When fw was increased in the mixture, the decreased absorbance and spectral tails in the long wavelength region were clear probably due to the formation of aggregates,12 and the observed red-shift indicated that J-aggregate could be formed in the mixture.13 Interestingly, the absorption spectra of TPE-D-Glu displayed a small red-shift of 7 nm compared to TPE-L-Glu, which might be ascribed to the different sizes of the formed nanoparticles.
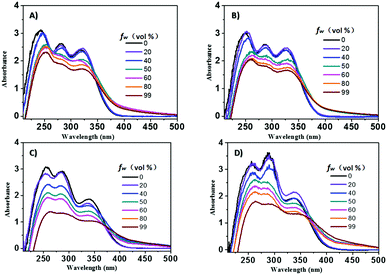 |
| Fig. 1 UV-vis absorption spectra of (A) TPE-L-Glu, (B) TPE-D-Glu, (C) cis-TPE-L-DGlu and (D) trans-TPE-L-DGlu in THF/water mixture with different fw. Concentration: 1 × 10−5 M. | |
Next, we investigated their CD spectra. As shown in Fig. 2A, the CD data of the mono-substituted compounds demonstrated that both compounds were CD silent in THF. However, the aggregation-induced CD (AICD) signals could be observed with an increase in fw of the mixed solvents. The two enantiomers exhibited slightly deflected mirror-imaged AICD signals, whose signals were consistent with their absorption data. Moreover, we found that the AICD signals apparently enhanced with higher fw, and could reach the maximum when fw was at 99%.
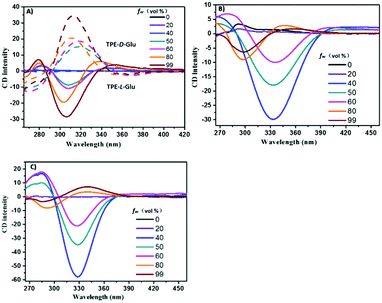 |
| Fig. 2 CD spectra of (A) TPE-D-Glu and TPE-L-Glu, (B) cis-TPE-L-DGlu, (C) trans-TPE-L-DGlu in THF/water mixtures with different fw. Solution concentration = 1 × 10−5 M. | |
As depicted in Fig. 2B and C, cis-TPE-L-DGlu presented a very weak signal at 290 nm in THF, while trans-TPE-L-DGlu had almost no signal at the same condition. The different AICD signals in THF between two isomers could result from the formation of the intramolecular hydrogen bond of the cis-isomer. Moreover, obvious aggregation-induced Cotton effects were observed with increased fw. The negative signals at 330 nm of the isomers were highest when fw was at 40%, i.e., the CD signal of trans-isomer was about twice than that of cis-isomer probably due to the different hydrogen bonds of the inter- and intra-forms. However, the negative signals decreased and displayed a slight red-shift when water was present in the mixture until fw was up to 60%. Furthermore, the negative signals at about 296 nm and positive signals at about 350 nm of cis-TPE-L-DGlu were detected when more water was added. The trans-isomer had similar negative and positive signals, which were related to those of the cis-isomer. All the above data indicated that the point chirality of the glutamic moiety could transfer to the TPE part in aggregates.
For the two di-substituted isomers, trans–cis isomerization could theoretically occur in the excited state. However, when the size of substituents or the interactions between the substituents increase, the trans–cis isomerization becomes much more difficult, according to the previous reported works.11b,14 The fluorescence spectra of mono- and di-substituted compounds are shown in Fig. 3. As predicted, the four TPE-containing compounds were AIE-active. Their fluorescence intensities were relatively weak when fw was less than 40% in the mixed solvents and enhanced rapidly with the addition of more water due to the formation of aggregates. The highest fluorescent intensities could increase to 164, 176 and 138 times for TPE-L-Glu, cis-TPE-L-DGlu and trans-TPE-L-DGlu, respectively. Both the aggregates in 99% fw mixtures and their solids displayed high green emission. Moreover, the emission peaks shifted to longer wavelengths with increase in fw, which further indicated the formation of J aggregates.
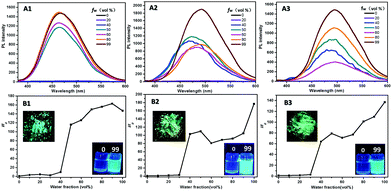 |
| Fig. 3 Fluorescent spectra of TPE-L-Glu, cis-TPE-L-DGlu and trans-TPE-L-DGlu in THF/water mixed solvents with different fw (A1–A3); plot of I/I0 values versus fw (B1–B3), where I0 represents the emission intensity in THF. Concentration: 1 × 10−5 M; excitation wavelength: 340 nm. Inset: Photographs of TPE-L-Glu, cis-TPE-L-DGlu and trans-TPE-L-DGlu in solid and THF/water mixtures with 0 and 99% fw under 365 nm UV lamp. | |
The aggregates exhibited strong fluorescence and obvious AICD signals, which inspired us to further investigate their CPL properties. As shown in Fig. 4A, TPE-L-Glu and TPE-D-Glu exhibited mirrored AICPL spectra in the region from 400 to 600 nm when fw was higher than 50%. The highest AICPL signals decreased and red-shifted when more water was added to the mixed solvents. The CPL dissymmetry factor, glum, could reach 0.021 and −0.019 for TPE-L-Glu and TPE-D-Glu, respectively. Interestingly, the original AICPL signals of cis-TPE-L-DGlu and trans-TPE-L-DGlu exhibited opposite signs compared with TPE-L-Glu although they had the same chiral L-glutamic moiety, which could be attributed to the different intermolecular noncovalent interactions.6a,15 The two di-substituted compounds possessed similar AICPL spectra with the highest signal at 60% fw, and their AICPL signals could reverse when fw was higher than 80%, whose glum values changed from −0.007 to 0.003 for cis-isomer, and from −0.008 to 0.002 for the trans-isomer, respectively. All the data indicated that the AICPL properties of these TPE derivatives could be adjusted by both the chirality of chiral source (L or D-stereoisomers) and the number of introduced substituents (mono- or di-substituted). The strength of the CPL signals could be tuned by changing fw in the mixed solvents.
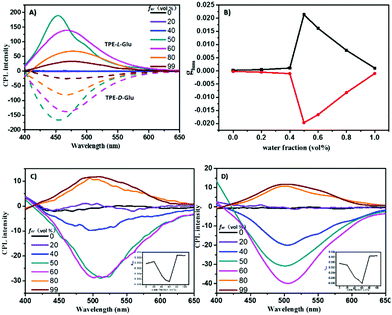 |
| Fig. 4 CPL spectra of (A) TPE-L-Glu and TPE-D-Glu, (C) cis-TPE-L-DGlu, (D) trans-TPE-L-DGlu in THF/water mixed solvents. Concentration: 1 × 10−5 M; excitation wavelength: 340 nm. (B) glumversus fw of TPE-L-Glu and TPE-D-Glu. Inset: glumversus fw of cis-TPE-L-DGlu and trans-TPE-L-DGlu. | |
The morphologies of the aggregates
According to the above optical data, all the compounds displayed tunable aggregation-induced optically active properties by changing fw of the mixed solvents, which stimulated us to investigate the relation between optical activities and supramolecular morphologies of the self-assembled aggregates. Moreover, to demonstrate the intermolecular interactions of the compounds even in THF, we obtained the SEM images of formed nanostructures after THF was volatilized. As shown in Fig. 5, the morphologies of formed self-assemblies were obviously different when the fw of the mixed solvents was changed. For the enantiotopic mono-substituted compounds, the SEM images of the compounds in THF (10 μM) showed the formation of similar nanofibers, which further showed intermolecular interactions even in the molecular state. Thicker nanofibers were detected when fw was increased to 40% due to the formation of aggregates. Moreover, helical nanofibers formed when 50% fw was in the mixed solvents, which L-enantiomer produced left-handed twist nanofibers and D-enantiomer gave the right-handed ones. However, irregular nanoparticles formed after more water was added. The different morphologies of formed nanostructures could lead to the changes in the AICPL intensities. For cis- and trans-isomeric compounds, different irregular nanoparticles were detected in the mixed solvents probably due to the different intra- or inter-molecular interactions. cis-TPE-L-DGlu with two L-glutamic moieties located at the same side experience intramolecular interactions that could lead to form nanoparticles to reduce energy.16 However, trans-TPE-L-DGlu with two substituents on the opposite sides could undergo intermolecular weak interactions and self-assembled in side-by-side formation to generate nanoribbons.17 The different noncovalent interactions resulted in the formation of diverse nanostructures and further affected the intensities of the AICPL signals of the two isomers. Note that the trans-one gave higher signals compared to the cis-one. The SEM images of the self-assemblies indicate that AICPL signals could be affected by the morphologies of formed microstructures, which the distinguishing formed nanostructures should originally ascribe to the different intermolecular interactions between the mono- and di-substituted AIEgens.
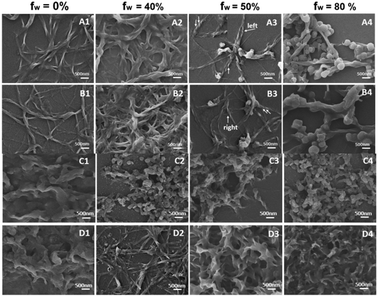 |
| Fig. 5 SEM images of TPE-L-Glu (A1–A4), TPE-D-Glu (B1–B4), cis-TPE-L-DGlu (C1–C4), trans-TPE-L-DGlu (D1–D4) obtained from THF/water mixed solvents at different fw. (A1–D1) 10/0; (A2–D2) 6/4; (A3–D3) 5/5; (A4–D4) 1/9. Concentration: 1 × 10−5 M. | |
Conclusions
In conclusion, we designed and synthesized four chiral AIEgens containing TPE and one or two chiral glutamic moieties that were connected by thiourea. All the compounds exhibited aggregation-induced optical activities in a THF/water mixture. The enantiotopic mono-substituted compounds gave mirror-imaged AICPL signals, whose |glum| value could reach 0.02. The di-substituted isomers showed opposed original AICPL signals compared with mono-substituted isomers although they had the same chiral glutamic moiety. cis- and trans-isomers displayed similar AICPL properties, whose signs of AICPL signals reversed when fw was more than 80%. The SEM images of the self-assemblies indicated that AICPL properties could be related to the number of substituents in molecules and the formed self-assembled microstructures.
Conflicts of interest
There are no conflicts to declare.
Acknowledgements
We gratefully acknowledge for financial support from the National Natural Science Foundation of China (No. 21702180), Jiangsu Provincial Nature Science Foundation (SBK2016021885), the Top-notch Academic Programs Project of Jiangsu Higher Education Institutions, and the Priority Academic Program Development of Jiangsu Higher Education Institutions.
Notes and references
-
(a) Y. Ying, R. C. da Costa, D.-M. Smilgies, A. J. Campbell and M. J. Fuchter, Adv. Mater., 2013, 25, 2624 CrossRef PubMed;
(b) M. Li, S.-H. Li, D. Zhang, M. Cai, L. Duan, M.-K. Fung and C.-F. Chen, Angew. Chem., Int. Ed., 2018, 57, 2889 CrossRef CAS PubMed;
(c) J. R. Brandt, X. Wang, Y. Yang, A. J. Campbell and M. J. Fuchter, J. Am. Chem. Soc., 2016, 138, 9743 CrossRef CAS PubMed;
(d) W. Li, Z. J. Coppens, L. V. Besteiro, W. Wang, A. O. Govorov and J. Valentine, Nat. Commun., 2015, 6, 8379 CrossRef CAS PubMed;
(e) J. Kumar, T. Nakashima, H. Tsumatori and T. Kawai, J. Phys. Chem. Lett., 2014, 5, 316 CrossRef CAS PubMed;
(f) H. Ruthanne, E. J. Swain, N. I. Hammer, D. Venkataraman and M. D. Barnes, Science, 2006, 314, 1437 CrossRef PubMed;
(g) C. Chen, J. Chen, T. Wang and M. Liu, ACS Appl. Mater. Interfaces, 2016, 8, 30608 CrossRef CAS PubMed;
(h) M. Shimada, Y. Yamanoi, T. Ohto, S.-T. Pham, R. Yamada, H. Tada, K. Omoto, S. Tashiro, M. Shionoya, M. Hattori, K. Jimura, S. Hayashi, H. Koike, M. Iwamura, K. Nozaki and H. Nishihara, J. Am. Chem. Soc., 2017, 139, 11214 CrossRef CAS PubMed;
(i) F. Song, Z. Xu, Q. Zhang, Z. Zhao, H. Zhang, W. Zhao, Z. Qiu, C. Qi, H. Zhang, H. H. Y. Sung, I. D. Williams, J. W. Y. Lam, Z. Zhao, A. Qin, D. Ma and B. Z. Tang, Adv. Funct. Mater., 2018, 28, 1800051 CrossRef.
-
(a) K. Nakamura, S. Furumi, M. Takeuchi, T. Shibuya and K. Tanaka, J. Am. Chem. Soc., 2014, 136, 5555 CrossRef CAS PubMed;
(b) T. Wu and P. Bouř, Chem. Commun., 2018, 54, 1790 RSC;
(c) Y. Wang, X. Li, L. Yang, W. Y. Sun, C. Zhu and Y. Cheng, Mater. Chem. Front., 2018, 2, 554 RSC;
(d) Y. Nagata, M. Uno and M. Suginome, Angew. Chem., Int. Ed., 2016, 55, 7126 CrossRef CAS PubMed;
(e) J. Zhang, Q. Liu, W. Wu, J. Peng, H. Zhang, F. Song, B. He, X. Wang, H. H.-Y. Sung, M. Chen, B. S. Li, S. H. Liu, J. W. Y. Lam and B. Z. Tang, ACS Nano, 2019, 13, 3618 CrossRef CAS PubMed;
(f) B. Zhao, K. Pan and J. Deng, Macromolecules, 2018, 51, 7104 CrossRef CAS;
(g) Y. Chen, X. Li, N. Li, Y. Quan, Y. Cheng and Y. Tang, Mater. Chem. Front., 2019, 3, 867 RSC;
(h) N. Zhao, W. Gao, M. Zhang, J. Yang, X. Zheng, Y. Li, R. Cui, W. Yin and N. Li, Mater. Chem. Front., 2019, 3, 1613 RSC.
-
(a) W. Z. Yuan, P. Lu, S. Chen, J. W. Y. Lam, Z. Wang, Y. Liu, H. S. Kwok, Y. Ma and B. Z. Tang, Adv. Mater., 2010, 22, 2159 CrossRef CAS PubMed;
(b) S. W. Thomas, G. D. Joly and T. M. Swager, Chem. Rev., 2007, 107, 1339 CrossRef CAS PubMed;
(c) J. Liang, B. Z. Tang and B. Liu, Chem. Soc. Rev., 2015, 44, 2798 RSC;
(d) A. S. Klymchenko, Acc. Chem. Res., 2017, 50, 366 CrossRef CAS PubMed.
-
(a) J. D. Luo, Z. L. Xie and B. Z. Tang, Chem. Commun., 2001, 1740 RSC;
(b) J. Mei, N. L. C. Leung, R. T. K. Kwok, J. W. Y. Lam and B. Z. Tang, Chem. Rev., 2015, 115, 11718 CrossRef CAS PubMed.
- J. Liu, H. Su, L. Meng, Y. Zhao, C. Deng, J. C. Y. Ng, P. Lu, M. Faisal, J. W. Y. Lam, X. Huang, H. Wu, K. S. Wong and B. Z. Tang, Chem. Sci., 2012, 3, 2737 RSC.
-
(a) H. Li, J. Cheng, Y. Zhao, J. W. Y. Lam, K. S. Wong, H. Wu, B. S. Li and B. Z. Tang, Mater. Horiz., 2014, 1, 518 RSC;
(b) X. Liu, J. Jiao, X. Jiang, J. Li, Y. Cheng and C. Zhu, J. Mater. Chem. C, 2013, 1, 4713 RSC;
(c) S. Zhang, Y. Wang, F. Meng, C. Dai, Y. Cheng and C. Zhu, Chem. Commun., 2015, 51, 9014 RSC;
(d) Q. Liu, Q. Xia, S. Wang, B. S. Li and B. Z. Tang, J. Mater. Chem. C, 2018, 6, 4807 RSC;
(e) J. Roose, B. Z. Tang and K. S. Wong, Small, 2016, 12, 6495 CrossRef CAS PubMed;
(f) G. Huang, R. Wen, Z. Wang, B. S. Li and B. Z. Tang, Mater. Chem. Front., 2018, 2, 1884 RSC.
-
(a) E. M. Sánchez-Carnerero, A. R. Agarrabeitia, F. Moreno, B. L. Maroto, G. Muller, M. J. Ortiz and S. de la Moya, Chem. – Eur. J., 2015, 21, 13488 CrossRef PubMed;
(b) Y. Zhang, D. Yang, J. Han, J. Zhou, Q. Jin, M. Liu and P. Duan, Langmuir, 2018, 34, 5821 CrossRef CAS PubMed;
(c) T. Goto, Y. Okazaki, M. Ueki, Y. Kuwahara, M. Takafuji, R. Oda and H. Ihara, Angew. Chem., Int. Ed., 2017, 56, 2989 CrossRef CAS PubMed;
(d) E. Yashima, N. Ousaka, D. Taura, K. Shimomura, T. Ikai and K. Maeda, Chem. Rev., 2016, 116, 13752 CrossRef CAS PubMed;
(e) D. Niu, Y. Jiang, L. Ji, G. Ouyang and M. Liu, Angew. Chem., Int. Ed., 2019, 58, 5946 CrossRef CAS PubMed.
-
(a) H. Rhee, I. Eom, S.-H. Ahn and M. Cho, Chem. Soc. Rev., 2012, 41, 4457 RSC;
(b) F. S. Richardson, Chem. Rev., 1979, 79, 17 CrossRef CAS.
-
(a) J. Wang, J. Mei, R. R. Hu, J. Z. Sun, A. J. Qin and B. Z. Tang, J. Am. Chem. Soc., 2012, 134, 9956 CrossRef CAS PubMed;
(b) C.-J. Zhang, G. Feng, S. Xu, Z. Zhu, X. Lu, J. Wu and B. Liu, Angew. Chem., Int. Ed., 2016, 55, 6192 CrossRef CAS PubMed;
(c) X. F. Fang, Y.-M. Zhang, K. Chang, Z. Liu, X. Su, H. Chen, S. X.-A. Zhang, Y. Liu and C. Wu, Chem. Mater., 2016, 28, 6628 CrossRef CAS.
-
(a) Q. Ye, D. Zhu, H. Zhang, X. Lu and Q. Lu, J. Mater. Chem. C, 2015, 3, 6997 RSC;
(b) B. S. Li, R. Wen, S. Xue, L. Shi, Z. Tang, Z. Wang and B. Z. Tang, Mater. Chem. Front., 2017, 1, 646 RSC;
(c) H. Li, B. S. Li and B. Z. Tang, Chem. – Asian J., 2019, 14, 674 CrossRef CAS PubMed;
(d) J. Han, J. You, X. Li, P. Duan and M. Liu, Adv. Mater., 2017, 29, 1606503 CrossRef PubMed;
(e) S. Zhang, Y. Sheng, G. Wei, Y. Quan, Y. Cheng and C. Zhu, Polym. Chem., 2015, 6, 2416 RSC;
(f) J. Li, C. Yang, C. Huang, Y. Wan and W.-Y. Lai, Tetrahedron Lett., 2016, 57, 1256 CrossRef CAS.
-
(a) M. Luo, X. Zhou, Z. Chi, S. Liu, Y. Zhang and J. Xu, Dyes Pigm., 2014, 101, 74 CrossRef CAS;
(b) H.-Q. Peng, X. Zheng, T. Han, R. T. K. Kwok, J. W. Y. Lam, X. Huang and B. Z. Tang, J. Am. Chem. Soc., 2017, 139, 10150 CrossRef CAS PubMed;
(c) T. Matsumoto, F. Yamada and T. Kurosaki, Macromolecules, 1997, 12, 3547 CrossRef;
(d) Y. Li, T. Wang and M. Liu, Soft Matter, 2007, 3, 1312 RSC.
-
(a) N. J. Hestand and F. C. Spano, Acc. Chem. Res., 2017, 50, 341 CrossRef CAS PubMed;
(b) C. Dai, D. Yang, W. Zhang, B. Bao, Y. Cheng and L. Wang, Polym. Chem., 2015, 6, 3962 RSC.
-
(a) M. E. Ziffer, S. B. Jo, Y. Liu, H. Zhong, J. C. Mohammed, J. S. Harrison, A. K.-Y. Jen and D. S. Ginger, J. Phys. Chem. C, 2018, 122, 18860 CrossRef CAS;
(b) V. Grande, B. Soberats, S. Herbst, V. Stepanenko and F. Würthner, Chem. Sci., 2018, 9, 6904 RSC;
(c) S. B. Anantharaman, T. Stöferle, F. A. Nüesch, R. F. Mahrt and J. Heier, Adv. Funct. Mater., 2019, 29, 1806997 CrossRef.
-
(a) J. Li, X. Peng, C. Huang, Q. Qi, W.-Y. Lai and W. Huang, Polym. Chem., 2018, 9, 5278 RSC;
(b) J. Li, C. Yang, X. Peng, Y. Chen, Q. Qi, X. Luo, W.-Y. Lai and W. Huang, J. Mater. Chem. C, 2018, 6, 19 RSC;
(c) X. Fang, Y.-M. Zhang, K. Chang, Z. Liu, X. Su, H. Chen, S. X.-A. Zhang, Y. Liu and C. Wu, Chem. Mater., 2016, 28, 6628 CrossRef CAS.
-
(a) H. Li, J. Cheng, H. Deng, E. Zhao, B. Shen, J. W. Y. Lam, K. S. Wong, H. Wu, B. S. Li and B. Z. Tang, J. Mater. Chem. C, 2015, 3, 2399 RSC;
(b) H. Li, X. Zheng, H. Su, J. W. Y. Lam, K. S. Wong, S. Xue, X. Huang, X. Huang, B. S. Li and B. Z. Tang, Sci. Rep., 2016, 6, 19277 CrossRef PubMed.
-
(a) I. H. Nayyar, E. R. Batista, S. Tretiak, A. Saxena, D. L. Smith and R. L. Martin, J. Polym. Sci., Part B: Polym. Phys., 2013, 51, 935 CrossRef CAS;
(b) A. Declemy, C. Rulliere and P. Kottis, Chem. Phys. Lett., 2017, 101, 401 CrossRef;
(c) Y.-Z. Zheng, Y. Zhou, Q. Liang, D.-F. Chen, R. Guo, C.-L. Xiong, X.-J. Xu, Z.-N. Zhang and Z.-J. Huang, Dyes Pigm., 2017, 141, 179 CrossRef CAS.
-
(a) H. Zhang, C. Guo, X. Wang, J. Xu, X. He, Y. Liu, X. Liu, H. Huang and J. Sun, Cryst. Growth Des., 2013, 13, 679 CrossRef CAS;
(b) Z. Wang, Y. Gong, L. Zhang, C. Jing, F. Gao, S. Zhang and H. Li, Chem. Eng. J., 2018, 342, 238 CrossRef CAS;
(c) C. Chen, T. Wang, Y. Fu and M. Liu, Chem. Commun., 2016, 52, 1381 RSC.
Footnote |
† Electronic supplementary information (ESI) available. See DOI: 10.1039/c9qm00358d |
|
This journal is © the Partner Organisations 2019 |
Click here to see how this site uses Cookies. View our privacy policy here.