DOI:
10.1039/C7OB02727C
(Communication)
Org. Biomol. Chem., 2018,
16, 34-37
Narcissistic chiral self-sorting of molecular face-rotating polyhedra†
Received
7th November 2017
, Accepted 20th November 2017
First published on 27th November 2017
Abstract
Narcissistic chiral self-sorting prevailed in the assembly of molecular face-rotating polyhedra from a C3h building block 5,5,10,10,15,15-hexabutyl-truxene-2,7,12-tricarbaldehyde and racemic mixtures of 1,2-diamines. Out of 124 possible stereoisomers, a pair of racemic polyhedra dominated, wherein (1R,2R)-diamines were segregated in AAAA polyhedra and (1S,2S)-diamines in CCCC polyhedra. This chiral self-sorting process is regulated by facial non-covalent interactions in the polyhedra. In contrast, D3h facial building blocks 1,3,5-tris-(4-formyl-phenyl)triazine and racemic mixtures of 1,2-diamines assembled into polyhedra without facial interactions, and their assembly process did not undergo apparent chiral self-sorting.
Introduction
Self-sorting is efficient in creating sophisticated supramolecular structures through simple steps,1 and has been widely applied to the synthesis of supramolecular polymers,2 gels,3 cages,4 macrocycles,5 topological molecules,6 capsules,7 and so on.8 Within complex mixtures, building blocks can differentiate between self and non-self-sorting based on their structural differences (e.g. size and shape).1c,d Chiral self-sorting, however, is particularly challenging because the only difference between a pair of enantiomers is the spatial arrangement of atoms.1f Molecular assemblies through chiral self-sorting have been mainly connected by non-covalent bonds, e.g. hydrogen bonding7b,9 and metal–ligand interactions,10 but these assemblies are difficult to separate. As a result, detailed investigation on stereoisomers of chiral self-sorting is rare.11 Dynamic covalent chemistry (DCC) provides reversible yet sufficiently strong bonds for the construction of robust supramolecular architectures.12 Recently, elegant examples of chiral self-sorting through DCC have been reported.5,11,13 Molecular design of building blocks and control over assembly processes (reaction temperature5 or solubility of the assemblies13b) can modulate the DCC-based chiral self-sorting assembly. If the enthalpy difference between stereoisomers is relatively small compared to the entropy difference, the assembly process is dominated by entropy.5 On the other hand, enthalpy-driven chiral self-sorting can be realized by introducing non-covalent bonds.13a In the area of DCC-based organic cages, however, there has been only one detailed investigation on chiral self-sorting reported by Mastalerz.13b Small enthalpy differences between the stereoisomers of salicylimine cage compounds allowed them to tune the assembly processes from narcissistic chiral self-sorting to social chiral self-sorting.
Recently our group reported a strategy to construct [4 + 6] face-rotating polyhedra (FRP) using 5,5,10,10,15,15-hexabutyl-truxene-2,7,12-tricarbaldehyde (TR) as the facial building block.14TR is a C3h-symmetric planar molecule, exhibiting either a clockwise (C) or an anti-clockwise (A) direction in a polyhedron (Fig. 1a). The directionality of faces leads to five possible stereoisomers for a molecular octahedron (Fig. 1).14,15 Interestingly, under thermodynamic equilibrium, (1R,2R)-cyclo-hexanediamine (CHDA) produces only AAAA polyhedra and (1S,2S)-CHDA produces only CCCC polyhedra. Due to its special form of chirality, our molecular FRP system could be used to investigate the assembly behaviour of chiral polyhedra.
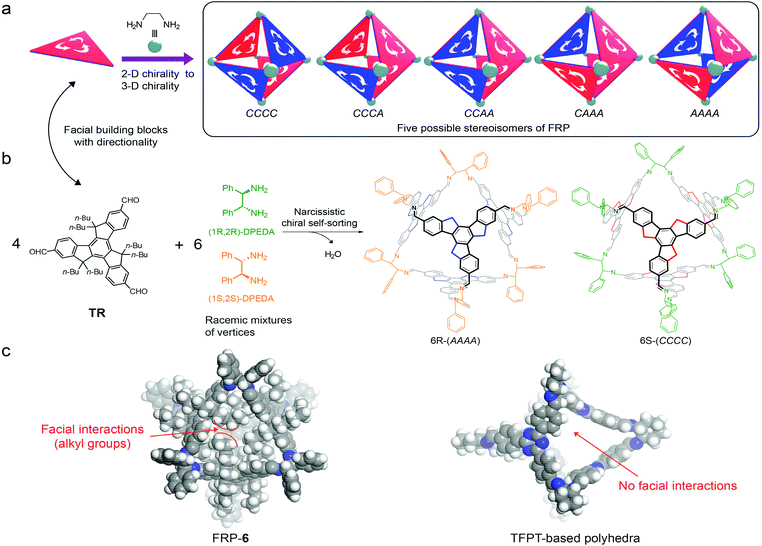 |
| Fig. 1 (a) Molecular face-rotating building block (TR) and ethylenediamine assembled into octahedra, which have five possible stereoisomers. (b) Assembly of TR with rac-DPEDA underwent narcissistic chiral self-sorting and gave rise to 6R-(AAAA) and 6S-(CCCC) out of 124 possible stereoisomers, butyl groups are omitted for clarity. (c) Crystal structures of polyhedra assembled by TR and TPFT. | |
Herein, we utilize our FRP systems to investigate the regulation of non-covalent interactions on the chiral self-sorting process. We assemble racemic mixtures of 1,2-diamines (rac-1,2-diamine) with two facial building blocks: TR or 1,3,5-tris-(4-formyl-phenyl)triazine (TFPT). Both TR and TFPT can form [4 + 6] polyhedra with the same geometry and size. The TR-based polyhedron has van der Waals forces between faces, whereas the TFPT based polyhedron does not have (Fig. 1c).
When rac-1,2-diamines are assembled into FRP as vertices, the number of possible stereoisomers increases exponentially due to three factors: chirality of vertices, directionality of faces and their relative arrangements. First, permutation of different chiral vertices within an octahedron gives rise to eleven possible stereoisomers (Fig. S25†). In addition, taking facial directionality into consideration, each of the possible stereoisomers have their own face-rotating patterns and relative arrangements of rotational faces and chiral vertices. The total number of possible stereoisomers is 124 (Fig. S26–S29†).
We assembled TR with two kinds of racemic mixtures of vertices, i.e. diphenylethylenediamine (DPEDA) and CHDA. The reversible imine formation between the aldehydes of TR and the amines of vertices ensured the ‘error checking’ and ‘proof-reading’ function and hence high yields of molecular polyhedra (Fig. 1). Since DPEDA has not been used as vertices of FRP yet, we first assembled TR with enantiopure DPEDA. The reaction at room temperature produced two stereoisomers. For instance, the assembly of TR and (1S,2S)-DPEDA resulted in FRP-5, which includes two stereoisomers as kinetic products, i.e., (CCCC)-5 and (CCCA)-5 (detailed experimental information is provided in the ESI†). In comparison, TR and enantiopure CHDA gave three stereoisomers as kinetic products according to our previous report.14 Different numbers of stereoisomers in the kinetic products of DPEDA and CHDA can be attributed to their different flexibilities in amine groups. Amine groups in DPEDA can rotate more freely than those of CHDA because of the ring strain of cyclohexane in CHDA.
TR was then assembled with rac-DPEDA and resulted in FRP-6. Stereoisomers in FRP-6 were investigated by nuclear magnetic resonance (NMR), chiral high performance liquid chromatography (HPLC), circular dichroism (CD) spectroscopy and single crystal X-ray analysis. In NMR spectra, the imine protons of FRP-6 exhibited only one set of singlets (Fig. 2b), indicating that all stereoisomers are T-symmetric. An analysis of the symmetry of 124 stereoisomers (Fig. S27–35†) suggested 6R-(AAAA) and 6S-(CCCC) as the only possible stereoisomers in FRP-6 (6R-(CCCC) and 6S-(AAAA) are thermodynamically unfavoured). Single crystals grown from FRP-6 confirmed the existence of only 6R-(AAAA) and 6S-(CCCC) as a pair of enantiomers. The two stereoisomers co-crystallized in the centrosymmetric space group R
. Furthermore, 6R-(AAAA) and 6S-(CCCC) were packed in segregated layers in the crystal, indicating chiral recognition during the crystallization process (Fig. 2c). To further confirm 6R-(AAAA) and 6S-(CCCC) as the only two stereoisomers, we separated FRP-6 using a chiral column, which showed two peaks eluting at 17.9 minutes and 20.3 minutes in a 1
:
1 ratio (Fig. 2a). We then measured their CD spectra and compared their normalized CD intensities with enantiopure ones. Our previous report14 found that the CD intensity of FRP is closely related to their rotational patterns. Based on this result, normalized CD intensities of the first and the second peaks are identical to those of enantiopure CCCC and AAAA polyhedra, respectively. Thus, the first peak had only CCCC and the second peak had only AAAA. These results confirmed that TR and rac-DPEDA underwent narcissistic chiral self-sorting and produced only two homo-chiral polyhedra as dominating stereoisomers. The degree of self-sorting (M) was calculated to be 62 (M = 124/2),16 indicating highly efficient chiral recognition during the assembly process.
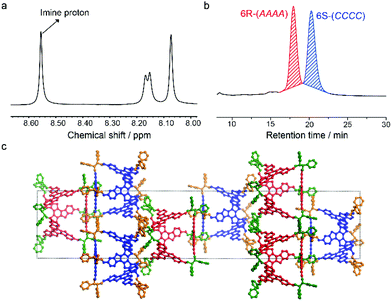 |
| Fig. 2 Characterisation of FRP-6: (a) 1H NMR spectrum of aromatic protons showed only one set of singlets, (b) chiral separation spectra showed two peaks, (c) single crystals of FRP-6 contain two types of stereoisomers in space group R , i.e. 6R-(AAAA) and 6R-(CCCC) (red: A faces, blue: C faces). | |
To extend the scope of chiral self-sorting systems, we then investigated the assembly of polyhedra using alternative faces and vertices. The influence of vertices was investigated by replacing DPEDA with 1,2-cyclohexanediamine (CHDA). Under thermodynamic equilibrium, the assembly of TR and rac-CHDA also produced only two homo-chiral polyhedra (6R-(AAAA) and 6S-(CCCC)) as dominating products (for experimental details, see the ESI†). Therefore, TR and rac-1,2-CHDA also underwent the narcissistic chiral self-sorting process.
The influence of facial building blocks was investigated by replacing C3h-symmetric TR with D3h-symmetric TFPT. The assembly of TFPT and rac-CHDA was carried out by following the literature procedure.17 Mass spectra suggested the clear formation of [4 + 6] polyhedra. But the NMR spectra of crude products were complicated (Fig. 3b). Chiral separation spectra clearly showed at least six stereoisomers in the products (Fig. 3d). This means that the assembly of TFPT and rac-CHDA did not undergo an apparent chiral self-sorting process. Another D3h-symmetric facial building block 1,3,5-triformyl benzene (TFB) also did not undergo the chiral self-sorting process when assembled with rac-1,2-CHDA.18 The contrast assembly behaviour between facial building blocks with and without facial interactions demonstrated the significance of non-covalent interactions in the DCC-based assembly process.
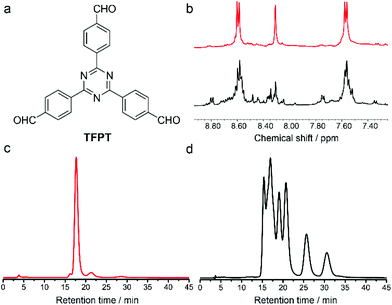 |
| Fig. 3 (a) Structure of TFPT, (b) NMR spectra of polyhedra assembled by TFPT and enantiopure (red) and rac-CHDA (black), (c), (d) chiral HPLC separation of crude products using (c) enantiopure CHDA and (d) rac-CHDA. | |
Conclusions
In conclusion, we achieved narcissistic chiral self-sorting of molecular face-rotating polyhedra through dynamic covalent bonds. van der Waals forces between faces lead to an impressively high degree of chiral self-sorting. Our research highlights the regulation of non-covalent forces on the DCC-based assembly process. The special form of chirality of molecular face-rotating polyhedra allowed each stereoisomer to be separated and characterized individually. This assembly system offers an opportunity for kinetic investigation of chiral self-sorting processes.
Conflicts of interest
There are no conflicts to declare.
Acknowledgements
This work is supported by the 973 Program (No. 2015CB856500), the NSFC (No. 21722304, 91427304, 21573181, 91227111 and 21102120) and Fundamental Research Funds for the Central Universities (No. 20720160050) of China. We thank Dr Xiao-Ye Wang, Ru-Qiang Lu and Hang Qu for discussions.
Notes and references
-
(a) S. J. Rowan, D. G. Hamilton, P. A. Brady and J. K. M. Sanders, J. Am. Chem. Soc., 1997, 119, 2578–2579 CrossRef CAS;
(b) A. Wu and L. Isaacs, J. Am. Chem. Soc., 2003, 125, 4831–4835 CrossRef CAS PubMed;
(c) B. H. Northrop, Y.-R. Zheng, K.-W. Chi and P. J. Stang, Acc. Chem. Res., 2009, 42, 1554–1563 CrossRef CAS PubMed;
(d) M. M. Safont-Sempere, G. Fernández and F. Würthner, Chem. Rev., 2011, 111, 5784–5814 CrossRef CAS PubMed;
(e) Z. He, W. Jiang and C. A. Schalley, Chem. Soc. Rev., 2015, 44, 779–789 RSC;
(f) H. Jȩdrzejewska and A. Szumna, Chem. Rev., 2017, 117, 4863–4899 CrossRef PubMed.
-
(a) C. Li, X. Shu, J. Li, J. Fan, Z. Chen, L. Weng and X. Jia, Org. Lett., 2012, 14, 4126–4129 CrossRef CAS PubMed;
(b) F. Wang, C. Han, C. He, Q. Zhou, J. Zhang, C. Wang, N. Li and F. Huang, J. Am. Chem. Soc., 2008, 130, 11254–11255 CrossRef CAS PubMed;
(c) Z. Huang, L. Yang, Y. Liu, Z. Wang, O. A. Scherman and X. Zhang, Angew. Chem., Int. Ed., 2014, 53, 5351–5355 CrossRef CAS PubMed;
(d) N. Tomimasu, A. Kanaya, Y. Takashima, H. Yamaguchi and A. Harada, J. Am. Chem. Soc., 2009, 131, 12339–12343 CrossRef CAS PubMed.
-
(a) J. R. Moffat and D. K. Smith, Chem. Commun., 2009, 3, 316–318 RSC;
(b) S. Cicchi, G. Ghini, L. Lascialfari, A. Brandi, F. Betti, D. Berti, P. Baglioni, L. Di Bari, G. Pescitelli, M. Mannini and A. Caneschi, Soft Matter, 2010, 6, 1655–1661 RSC;
(c) B. Adhikari, J. Nanda and A. Banerjee, Soft Matter, 2011, 7, 8913–8922 RSC.
-
(a) S. J. Rowan, D. J. Reynolds and J. K. M. Sanders, J. Org. Chem., 1999, 64, 5804–5814 CrossRef CAS;
(b) M. Wang, C. Wang, X.-Q. Hao, X. Li, T. J. Vaughn, Y.-Y. Zhang, Y. Yu, Z.-Y. Li, M.-P. Song, H.-B. Yang and X. Li, J. Am. Chem. Soc., 2014, 136, 10499–10507 CrossRef CAS PubMed;
(c) M. M. J. Smulders, A. Jimenez and J. R. Nitschke, Angew. Chem., Int. Ed., 2012, 51, 6681–6685 CrossRef CAS PubMed;
(d) A. M. Johnson, C. A. Wiley, M. C. Young, X. Zhang, Y. Lyon, R. R. Julian and R. J. Hooley, Angew. Chem., Int. Ed., 2015, 54, 5641–5645 CrossRef CAS PubMed;
(e) S. Klotzbach and F. Beuerle, Angew. Chem., Int. Ed., 2015, 54, 10356–10360 CrossRef CAS PubMed;
(f) K. Pandurangan, J. A. Kitchen, S. Blasco, E. M. Boyle, B. Fitzpatrick, M. Feeney, P. E. Kruger and T. Gunnlaugsson, Angew. Chem., Int. Ed., 2015, 54, 4566–4570 CrossRef CAS PubMed;
(g) K. Acharyya and P. S. Mukherjee, Chem. Commun., 2015, 51, 4241–4244 RSC;
(h) K. Acharyya, S. Mukherjee and P. S. Mukherjee, J. Am. Chem. Soc., 2013, 135, 554–557 CrossRef CAS PubMed.
- S. W. Sisco and J. S. Moore, Chem. Sci., 2014, 5, 81–85 RSC.
-
(a) C. Talotta, C. Gaeta, Z. Qi, C. A. Schalley and P. Neri, Angew. Chem., Int. Ed., 2013, 52, 7437–7441 CrossRef CAS PubMed;
(b) W. Jiang, Q. Wang, I. Linder, F. Klautzsch and C. A. Schalley, Chem. – Eur. J., 2011, 17, 2344–2348 CrossRef CAS PubMed;
(c) J.-F. Ayme, J. E. Beves, C. J. Campbell and D. A. Leigh, Angew. Chem., Int. Ed., 2014, 53, 7823–7827 CrossRef CAS PubMed.
-
(a) D. Ajami, J.-L. Hou, T. J. Dale, E. Barrett and J. Rebek Jr., Proc. Natl. Acad. Sci. U. S. A., 2009, 106, 10430–10434 CrossRef CAS PubMed;
(b) H. Jȩdrzejewska, M. Wierzbicki, P. Cmoch, K. Rissanen and A. Szumna, Angew. Chem., Int. Ed., 2014, 53, 13760–13764 CrossRef PubMed.
-
(a) C. Roche, H.-J. Sun, M. E. Prendergast, P. Leowanawat, B. E. Partridge, P. A. Heiney, F. Araoka, R. Graf, H. W. Spiess, X. Zeng, G. Ungar and V. Percec, J. Am. Chem. Soc., 2014, 136, 7169–7185 CrossRef CAS PubMed;
(b) A. Samanta, Z. Liu, S. K. M. Nalluri, Y. Zhang, G. C. Schatz and J. F. Stoddart, J. Am. Chem. Soc., 2016, 138, 14469–14480 CrossRef CAS PubMed;
(c) A. Pal, S. Karthikeyan and R. P. Sijbesma, J. Am. Chem. Soc., 2010, 132, 7842–7843 CrossRef CAS PubMed;
(d) J.-M. Lehn, Science, 2002, 295, 2400–2403 CrossRef CAS PubMed.
-
(a) L. J. Prins, J. Huskens, F. de Jong, P. Timmerman and D. N. Reinhoudt, Nature, 1999, 398, 498–502 CrossRef CAS;
(b) A. Wu, A. Chakraborty, J. C. Fettinger, R. A. Flowers II and L. Isaacs, Angew. Chem., Int. Ed., 2002, 41, 4028–4031 CrossRef CAS;
(c) A. T. Ten Cate, P. Y. W. Dankers, H. Kooijman, A. L. Spek, R. P. Sijbesma and E. W. Meijer, J. Am. Chem. Soc., 2003, 125, 6860–6861 CrossRef CAS PubMed.
-
(a) E. J. Enemark and T. D. P. Stack, Angew. Chem., Int. Ed., 1998, 37, 932–935 CrossRef CAS;
(b) M. Hutin, C. J. Cramer, L. Gagliardi, A. R. M. Shahi, G. Bernardinelli, R. Cerny and J. R. Nitschke, J. Am. Chem. Soc., 2007, 129, 8774–8780 CrossRef CAS PubMed;
(c) L.-L. Yan, C.-H. Tan, G.-L. Zhang, L.-P. Zhou, J.-C. Bünzli and Q.-F. Sun, J. Am. Chem. Soc., 2015, 137, 8550–8555 CrossRef CAS PubMed.
- C. Tsiamantas, X. de Hatten, C. Douat, B. Kauffmann, V. Maurizot, H. Ihara, M. Takafuji, N. Metzler-Nolte and I. Huc, Angew. Chem., Int. Ed., 2016, 55, 6848–6852 CrossRef CAS PubMed.
-
(a) C. D. Meyer, C. S. Joiner and J. F. Stoddart, Chem. Soc. Rev., 2007, 36, 1705–1723 RSC;
(b) M. Mastalerz, Angew. Chem., Int. Ed., 2010, 49, 5042–5053 CrossRef CAS PubMed;
(c) M. E. Belowich and J. F. Stoddart, Chem. Soc. Rev., 2012, 41, 2003–2024 RSC;
(d) Y. Jin, C. Yu, R. J. Denman and W. Zhang, Chem. Soc. Rev., 2013, 42, 6634–6654 RSC;
(e) S. J. Rowan, S. J. Cantrill, G. R. L. Cousins, J. K. M. Sanders and J. F. Stoddart, Angew. Chem., Int. Ed., 2002, 41, 898–952 CrossRef.
-
(a) M. Petryk, K. Biniek, A. Janiak and M. Kwit, CrystEngComm, 2016, 18, 4996–5003 RSC;
(b) D. Beaudoin, F. Rominger and M. Mastalerz, Angew. Chem., Int. Ed., 2017, 56, 1244–1248 CrossRef CAS PubMed.
- X. Wang, Y. Wang, H. Yang, H. Fang, R. Chen, Y. Sun, N. Zheng, K. Tan, X. Lu, Z. Tian and X. Cao, Nat. Commun., 2016, 7, 12469 CrossRef PubMed.
- Y. Wang, H. Fang, W. Zhang, Y. Zhuang, Z. Tian and X. Cao, Chem. Commun., 2017, 53, 8956–8959 RSC.
- M. Lal Saha and M. Schmittel, Org. Biomol. Chem., 2012, 10, 4651–4684 CAS.
- H. Ding, Y. Yang, B. Li, F. Pan, G. Zhu, M. Zeller, D. Yuan and C. Wang, Chem. Commun., 2015, 51, 1976–1979 RSC.
- G. Zhu, C. D. Hoffman, Y. Liu, S. Bhattacharyya, U. Tumuluri, M. L. Jue, Z. Wu, D. S. Sholl, S. Nair, C. W. Jones and R. P. Lively, Chem. – Eur. J., 2016, 22, 10743–10747 CrossRef CAS PubMed.
Footnote |
† Electronic supplementary information (ESI) available: Experimental details such as NMR, mass spectra, crystal data and possible isomers. CCDC 1560158 and 1562100. For ESI and crystallographic data in CIF or other electronic format see DOI: 10.1039/c7ob02727c |
|
This journal is © The Royal Society of Chemistry 2018 |
Click here to see how this site uses Cookies. View our privacy policy here.