Green chemistry teaching in higher education: a review of effective practices†
Received
13th September 2011
, Accepted 12th January 2012
First published on 22nd February 2012
Abstract
This account reviews published green chemistry teaching resources in print and online literature and our experiences in teaching the subject to undergraduate students. Effective practices in lecture and laboratory are highlighted and ongoing challenges are addressed, including areas in cutting edge green chemistry research that impact its teaching in the undergraduate curriculum. In particular, the influence of green chemistry on the overall teaching of organic chemistry is discussed.
Introduction
As discussed recently (Anastas, 2011), the guiding theories and practices of green chemistry were first proposed twenty years ago and have since become entrenched in the chemical literature. The seminal book on the subject was published some years later which included a formulation of the Twelve Principles of Green Chemistry (Anastas and Warner, 1998). Subsequently, twelve more principles were suggested that are targeted at process chemists, chemical engineers and chemical technologists (Winterton, 2001). Although this timeframe implies that it is still a relatively young area, several greener chemical approaches were adopted many decades before the sustainability movement began. Some instances are highlighted in Table 1, and indicate, for example, that the notion of eliminating reaction solvents or using alternatives such as water or ionic liquids has a rich history. Table 1 additionally shows that a reaction considered green from one perspective (solvent-free) can also be viewed as decidedly “un-green” (using HgCl2 as a reagent) (Parker, 1914). Nevertheless, these illustrations give credence to the claim that “new chemical techniques are unnecessary to achieve greener chemistry. Rather, it requires redirection of current techniques and capabilities within a new set of individual expectations” (Tucker, 2010).
Table 1 Selected historical examples of greener chemical approaches
Greener approach |
Description |
Ref. |
Solvent-free transformations
|
Disruption of silver chloride by mechanical force (mechanochemistry) |
Lea, 1892
|
|
Dry reaction between HgCl2 and KI upon grinding |
Parker, 1914
|
|
Synthesis of 2,4-dichlorophenoxyacetic acid (component of Agent Orange) |
Manske, 1949
|
Alternative solvents
|
Water as the solvent for urea synthesis from ammonium cyanate |
Wöhler, 1828
|
|
Formation and use of ionic liquids |
Walden, 1914; Calloway, 1935 |
Catalysis
|
Vitamin B1 (thiamine) biocatalysis of condensation reactions |
Breslow, 1958
|
|
Organocatalysis of condensation reactions by amines |
Verley, 1899; Doebner, 1900; Cope, 1937 |
|
Enzyme-catalyzed organic reactivity |
Warburg, 1906; Pottevin, 1906; Rosenthaler, 1908 |
Multi-component reactions
|
Preparation of dihydropyridines and dihydropyrimidones |
Hantzsch, 1882; Biginelli, 1893 |
Biofeedstocks
|
Synthesis of furfural and levulinic acid (used to make 2-methyltetrahydrofuran, a greener solvent) from corn cobs and cane sugar, respectively |
Adams and Voorhees, 1921; McKenzie, 1929 |
Sonochemistry
|
Reaction rate enhancements by high frequency sound waves |
Richards and Loomis, 1927
|
Greener reagents
|
Pyridinium tribromide as an alternative brominating agent to molecular Br2 |
Djerassi and Scholz, 1948
|
As with any new area of scientific research, educators have contemplated strategies to integrate green principles into undergraduate and graduate curricula. One early course (“Introduction to Green Chemistry”) was offered at Carnegie Mellon University and described in the Journal of Chemical Education (JCE) (Collins, 1995). This represented the first pedagogical reference to green chemistry in JCE. Since that time, over 110 articles have appeared in JCE regarding an aspect of green instruction (Fig. 1). From January 1st 2004 until December 31st 2011, this amounted to an average of one publication per monthly issue. The need for these resources is emphasized by comments from graduate students after attending the third annual American Chemical Society Green Chemistry Summer School (GCSS) in Montréal, Canada. It was noted that “a common belief among the GCSS participants was that our education would have been significantly enhanced with the incorporation of green chemistry, beginning at the elementary level and continuing throughout graduate course work” (Kitchens et al., 2006). Other authors have reinforced the requirement of cultural change in chemical education, with statements such as “academically, a green chemistry culture can be created through higher expectations of sustainability taught via new curricula, and updated textbooks incorporating the 12 principles. This exemplification must become a primary leadership goal predicated upon a belief in the long-term advantage of superior, evolutionary, sustainable science” (Tucker, 2010).
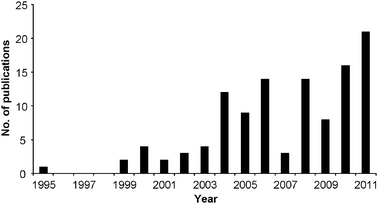 |
| Fig. 1 Annual number of publications dealing with green chemistry pedagogy (1995–2011, J. Chem. Educ.). | |
Given the wealth of resources available, and the documented importance of green chemistry education, this article reviews successful published instructional techniques in the field. It outlines the challenges faced in teaching green principles at the post-secondary level, focuses on advantageous approaches and concludes with a discussion of necessary improvements in green teaching and research. The paper is written from the perspective of two North American teaching faculty members who have fourteen years combined experience of teaching green chemistry to undergraduates from both lecture and laboratory perspectives.
Challenges in teaching green chemistry
1. Examples across the chemical sub-disciplines
Most literature examples of green chemistry in the context of optimized syntheses are from an organic perspective. This includes pharmaceutically important target molecules such as sildenafil citrate (Viagra™) (Dunn et al., 2004; Dicks and Batey, 2012; Edward, 2012) and ibuprofen (Advil™) (Cann and Connelly, 2000). As such, the vast majority of green teaching resources have been developed in the field of organic synthesis. However, the term “green chemistry” is not always used by industrial process chemists in their published works, and there are historical examples of optimized syntheses that clearly fit the goals and description of the Twelve Principles. Some of these methods go back a long way in the literature, and are well before any awareness of environmental impacts became fashionable (as described in the introduction to this article). There is a growing need to expand the range of pedagogical materials from other chemical subdisciplines (most notably within analytical/environmental and physical chemistry), although some resources do exist as outlined in Table 4.
2. Primary literature metric issues
There has been much confusion over the terminology of green chemistry metrics during the last twenty years (Andraos, 2012(a)). A lack of metric standardization in the primary research literature (and how each metric should be employed) has lead to significant misunderstandings. Indeed, “the field of green metrics is plagued by authors’ continuous changing of the names of the same ideas, which gives the impression that there is yet a new metric to be defined, with a new insight. This has been a serious liability towards the acceptance of this topic as a useful concept in the routine practice of tracking the optimization of chemical reactions” (Calvo-Flores, 2009). In some instances very similar approaches have been given subtly different names by different authors, which can hinder the teaching of fundamental green concepts. For example, the terminologies “E-factor”, “mass intensity”, “mass productivity” and “process mass intensity” all refer to metrics highlighting the amount of material used in a chemical process (Henderson et al., 2010). A rationale has recently been proposed why process mass intensity is the preferred metric choice in the pharmaceutical industry (Jiménez-González et al., 2011). This has prompted an editorial policy change in the submission of papers to Organic Process Research and Development which must now include some kind of metrics evaluation to assess the environmental impact and greenness of a chemical process or synthesis. Authors are also encouraged to follow green chemistry principles with respect to the choice of reaction solvent (Laird, 2012). Educators have an increasingly challenging task in deciding which concepts to teach and the specific literature to direct students towards. The use of green metrics has been discussed from a teaching perspective (Andraos and Sayed, 2007; Ribeiro et al., 2010).
3. Student textbook issues
Green chemistry ideas and examples are generally presented as “optional material” (usually as side bars and vignettes) in organic undergraduate textbooks (Smith, 2011; Vollhardt and Schore, 2011; McMurry, 2012). Items included are typically not part of the core material that students need to know (and will not be tested on), and are largely described in a “show and tell” manner. A related problem is that quantitative analysis of synthesis is seldom part of the chemical education students receive. Textbooks routinely present a “yield is everything” mentality, with little or no reference to overall reaction efficiencies or identification of reaction by-products. At the introductory level, discussions deal only with number of steps and overall yield as the key parameters for synthesis optimization.
There are additional issues with how the subject of organic chemistry is presented in some introductory textbooks. The following is an outline of some important concerns:
• textbooks are often organized according to functional groups, not patterns of reactivity
• chemical transformations are rarely (if ever) balanced
• there are few citations to the original literature, so students are forced to believe what the author says without checking for themselves the validity of the claims made
• examples are often not taken from the original literature, especially when discussing fundamental concepts such as molecular structure and bonding
• there is a disconnect between concepts introduced and the originator(s) of the idea, and the circumstances around which the idea arose (the mechanics of how research is done is not shown to the student)
• there is an overwhelming emphasis on individual examples (memorization) rather than on pattern recognition (understanding).
From a green chemistry perspective, there are also some textbook “myths” that impact the impressions students have about organic synthesis. These are listed below:
• the terms “side product” and “by-product” are synonymous
• convergent synthesis plans are always more material-efficient than linear plans
• short synthesis plans are always more material-efficient than long plans
• the amount of catalyst used in a reaction (catalyst loading) is much smaller than the substrate on which it acts.
4. Curricular issues
Perhaps surprisingly, there are still relatively few undergraduate green chemistry courses in Canada that are available for students to take (Andraos, 2012(a)). There are significantly more offerings in the United States, with one model published in JCE (Marteel-Parrish, 2007), and another taught at Monash University, Australia (Raston and Scott, 2001). Such courses are invariably considered electives, and are not mandatory to fulfill the requirements of an undergraduate chemistry major program. A common misconception within academia is that green chemistry is a “soft” and descriptive subject, with only rudimentary numerical analysis required (Amato, 1993). Quantitative reasoning is usually not considered a necessary skill in synthetic organic chemistry courses at any undergraduate level. In fact, the essential skill of balancing chemical equations is often not carried forward into organic courses from first year general courses, yet this is compulsory for the proper itemization and quantification of waste material produced in chemical reactions. Toxicology, hazard analysis, safety, and waste minimization strategies are subjects not routinely included in the core curriculum for the chemistry major degree. Finally, green chemistry is often mistaken as being synonymous with environmental chemistry amongst students and sometimes even research faculty.
Effective teaching methods
1. Stand-alone course versus cross-curricular implementation
An instructor planning to introduce green chemistry into an undergraduate program is typically faced with the following dilemma: is it better to design a brand-new, stand-alone course, or to try and add greener modules to existing courses across the curriculum? The pedagogical literature has shown that either approach can be successful, but that there are obvious “pros and cons” (Andraos, 2012(a)).
Launching a new offering with “green” in the title will capture undergraduate interest given the current publicized issues of sustainability and global environmental stewardship (Marteel-Parrish, 2007). Ideas and concepts can be thoroughly discussed and placed within the context of “real-world” case studies from the current chemical literature, leading to development of more advanced problems and assignments. Considering the extra time a dedicated course affords, students more clearly appreciate that for problems posed in this field there is no single “correct green answer”, but rather there are a range of options that need to be evaluated according to some criteria, and then a decision is made as to which “answer” is best. A multivariate green exercise has recently been published where students consider nine life-cycle assessment metrics in the context of an organic transformation (e.g. benzene to aniline) (Mercer et al., 2012). The instructor selects several literature methods for each transformation with the student goal of selecting the greenest route. In a similar vein at the University of Porto, Portugal, students search for the greenest metal-acetylacetonate synthesis experiment commonly included in the teaching laboratory literature, and attempt to improve its greenness (Ribeiro and Machado, 2011). This idea of considering multiple solutions and making decisions is typically a tremendous departure from their previous chemistry learning experiences. There is also a shift in how an instructor is to evaluate student assignments based on this paradigm.
Integration of a green chemistry course is preferable with other non-science related courses in areas of business, ethics, law and regulatory affairs, that have relevance in team-driven research strategies of the chemical industry. Conversely, a new course is time-consuming to prepare and may not find room within an already-crowded curriculum, especially if it is proposed as an elective. There will likely be a significant learning curve for the instructor(s) involved in delivering the course. Significant resistance may arise from research faculty who object to precious resources being spent in such a manner.
In comparison, the “greening” of existing courses has the advantage of being less time-consuming in terms of implementation. Fewer resources are required in terms of administrative costs. Students benefit from potentially seeing green principles from a variety of different perspectives in both lecture and laboratory venues, and new textbooks are unnecessary. However, green approaches will likely have to replace some more traditional content (which may be unpalatable), and material cannot be covered at the same level of detail compared with a stand-alone course. This strategy requires “buy-in” from a number of faculty members and instructors within a department, and ongoing close coordination between them.
2. Primary literature “real-world” case studies
The use of cutting-edge, up-to-the-minute case studies is an essential aspect of effective green chemistry teaching (Andraos, 2012(a)). These can be simply incorporated into either theoretical or practical components of almost any course. An emphasis has previously been placed on highlighting the United States Presidential Green Chemistry Challenge Awards (Cann, 1999; Marteel-Parrish, 2007). These annual recognitions are in five categories: the Greener Synthetic Pathways Award, the Greener Reaction Conditions Award, the Designing Greener Chemicals Award, the Small Business Award and the Academic Award (www.epa.gov/gcc/pubs/pgcc/award_categories.html). The project details of many awardees are suitable for inclusion in undergraduate curricula, Table 2 lists three examples from the five 2011 winners. Other instructive real-world organic teaching examples have been described (Cann and Dickneider, 2004). Finding current green literature written at an appropriate level for instructors and students at different academic levels to digest is straightforward. Excellent journals include, but are not limited to Organic Process Research and Development, ChemSusChem, Green Chemistry and Chemical & Engineering News (Table 2). Several articles in Green Chemistry specifically deal with undergraduate teaching methodologies (Matlack, 1999; Lennon et al., 2002; Tavener et al., 2003; Grant et al., 2004; Grant et al., 2005).
Table 2 Recent case studies suitable for undergraduate curricula
Journal/resource title |
Recent case study |
Ref. |
US Presidential Green Chemistry Challenge Awards
|
Surfactant for transition metal catalysis of organic reactions in water |
Ritter, 2011(a)
|
|
Halogen-free copolymer membranes for water purification |
Ritter, 2011(b)
|
|
Cost-effective microbial fermentation route to 1,4-butanediol |
Ritter, 2011(c)
|
Organic Process Research and Development
|
Enzyme-mediated preparation of Lyrica |
Martinez et al., 2008
|
|
Solvent-free and chemoselective carboxylic acid esterifications |
Rekha et al., 2009
|
ChemSusChem
|
Direct surfactant synthesis using dodecylbenzenesulfonic acid catalyst |
Gaudin et al., 2011
|
|
Flow microreactors for industrial scale processes |
Yoshida et al., 2011
|
Green Chemistry
|
Isolation of orange oil components using distillation from ionic liquid |
Bica et al., 2011
|
|
Perkin reaction in ionic liquid solvent |
Pawar et al., 2011
|
Chemical & Engineering News
|
Phasing out manufacture of a flame retardant (decabromodiphenyl ether) |
Hess, 2010
|
|
Production of hardened building materials with low energy demands |
Voith, 2010
|
Many web-based green chemistry materials have additionally been designed and published during the last decade. Table 3 illustrates a selected number of these to highlight the variety of features available. There are on-line repositories of successful teaching modules (some submitted by authors worldwide), websites to promote educator networking, metrics calculator programs and papers from a virtual green chemistry teaching conference. A significant number of these resources were developed from the primary literature, and more will undoubtedly appear as time progresses.
Table 3 Selected web-based green chemistry teaching resources
Resource |
Organizing institution |
Description |
Greener Education Materials for Chemists (GEMs) |
University of Oregon, USA |
searchable database to sort teaching resources (theoretical and practical) by academic level and desirable green principles (Haack et al., 2005) |
NOP (“Nachhaltigkeit im Organisch-chemischen Praktikum”): Sustainability in the Organic Chemistry Laboratory Course |
Universität Regensburg, Germany |
collection of laboratory experiments used to incorporate sustainability and green chemistry into undergraduate courses (Ranke et al., 2008) |
Green Chemistry Resource Exchange
|
American Chemical Society Green Chemistry Institute |
archive of materials concerning green chemistry and related novel technologies |
Green Chemistry Education Network (GCEdNet) |
University of Oregon, USA |
educator network to facilitate sharing of greener teaching strategies |
Green Chemistry Assistant
|
St. Olaf College, Minnesota, USA |
calculator to determine green parameters (e.g. theoretical atom economy) for a reaction of interest |
The Ecoscale
|
EcoSynth, Oostende, Belgium |
post-synthesis tool to evaluate “greenness” of an organic reaction (Van Aken et al., 2006) |
Educating the Next Generation: Green and Sustainable Chemistry |
American Chemical Society Committee on Computers in Chemical Education |
on-line green chemical education conference; seven academic papers presented during April–June 2010 |
3. Quantitative problem set questions & decision-making
As stressed previously, a common perception of green chemistry is that it is a qualitative discipline, rather than a quantitative one. Contrary to this, it is critical that instructors move away from a “show and tell” approach to a decision-making approach which is based on numerical data. A written assignment has been included in an upper-level green chemistry course that requires undergraduates to critique several synthesis plans towards a “real-world” molecule (e.g. a cosmetic or a dyestuff), using sustainability principles (Andraos, 2012(a)). Students independently select a target compound themselves from a list and their work is evaluated in terms of both content and presentation. It is noteworthy that one undergraduate report from this assignment has been published in a peer-reviewed journal (Andraos and Izhakova, 2006). Adopting this approach leads to student discovery that green chemistry is all about decision making—by its very nature, it is often an indefinite and ambiguous field. The final decision is a choice based on weighing favourable and unfavourable factors until an acceptable compromise is achieved, although the main goal is reaching certainty when a truly “green” optimized plan is established (Mercer et al., 2012). The quest for “greening” reactions is an iterative process that is never fully reached. There is always room for improvement as new reactions and methodologies are constantly being discovered. Important target molecules (such as the ones analyzed in the assignment described here) are always revisited for trying out new synthetic strategies. A corollary key concept is that the evaluation of “greenness” is a relative comparison and not an absolute one. This leads to the realization that if there is only one documented synthesis plan available for a given target molecule then its “greenness” ranking cannot be determined formally, although it may have some green attributes according to the Twelve Principles.
4. Laboratory resources
It is clear from peer-reviewed materials that a meaningful way to teach and learn green chemistry is in a laboratory setting. The first experiment published to appeal to organic instructors was “an environmentally benign synthesis of adipic acid” (Reed and Hutchison, 2000). Since that time, dedicated organic laboratory textbooks have reached the market (Kirchhoff and Ryan, 2002; Doxsee and Hutchison, 2004; Roesky and Kennepohl, 2009). This literature provides detailed experimental notes for students to follow and a green perspective on each performed reaction. Reviews of undergraduate solvent-free reactions and aqueous organic transformations have also been compiled (Dicks, 2009(a), 2009(b)). More recently, a contributed textbook (“Green Organic Chemistry in Lecture and Laboratory”) seeks to summarize major developments made in the field during the last decade (Dicks, 2012). Surprisingly, however, a recent survey of over 130 undergraduate organic chemistry laboratories in the United States showed that although waste reduction was a significant consideration, green practical techniques were deemed relatively unimportant (Martin et al., 2011).
From these published textbooks, one may begin to think that green principles only impact organic chemists. However, book chapters have been written on (i) teaching green analytical chemistry with laboratory examples (Gron, 2009) and (ii) incorporating environmental issues into inorganic chemistry (Huheey, 1996). A search of the recent pedagogical literature identifies a number of appropriate experiments in “non-organic” areas (Table 4), illustrating the cross-curricular impact of green chemistry. More work is needed in these venues to develop a comprehensive suite of practical resources that facilitates implementation within larger and smaller courses. The journal Green Chemistry has additionally published educational efforts from a laboratory perspective (Warner et al., 2001; Houri and Wehbe, 2003; McKenzie et al., 2004).
Table 4 Greener undergraduate laboratory experiments in “non-organic” areas
Field/sub-discipline |
Experiment description |
Ref. |
Introductory/General |
Colligative properties of fatty acids |
McCarthy and Gordon-Wylie, 2005; Iacobucci et al., 2006 |
|
Stoichiometry: composition of a sodium carbonate/sodium bicarbonate mixture |
Cacciatore and Sevian, 2006
|
|
Nanoparticle synthesis using a plant leaf extract |
Richardson et al., 2006
|
|
Solubility, equilibrium, and periodicity concepts surrounding Group II hydroxides |
Cacciatore et al., 2008
|
|
Calculating the formula of copper(II) chloride dihydrate |
Klingshirn et al., 2008
|
|
Superconductor synthesis: the Meissner effect |
She and Liu, 2008
|
Analytical
|
Spectrophotometric measurement of creatinine in urine |
Correia et al., 2004
|
|
Measurement of mercury in cow and goat milk by atomic fluorescence |
Armenta and de la Guardia, 2011
|
|
Electrochemical cell for cyclic voltammetry |
Olson and Buhlmann, 2010
|
Biological
|
Purification of Escherichia coli DNA using laundry detergent |
Sims et al., 2010
|
Environmental
|
Extraction of metal contaminants from environmental samples with micelles |
Giokas et al., 2003
|
Inorganic
|
Preparation of tetraamido-N Cu(III) and Co(III) complexes |
Uffelman et al., 2004(a), 2004(b)
|
|
Cation qualitative analysis via spot tests |
Sidhwani and Chowdhury, 2008
|
|
Synthesis of Group II metal oxalate hydrates |
Canal, 2009
|
|
Metalloporphyrins as oxidation catalysts |
Clark et al., 2012
|
|
Solvent-free synthesis of Cu(II) phthalocyanine |
Sharma et al., 2011
|
Materials
|
Alkanethiol monolayer formation on gold films |
McKenzie et al., 2004
|
Physical
|
Synthesis and analysis of quantum dot nanocrystals |
Boatman et al., 2005
|
|
Properties of biodiesel: heat of combustion, density and cloudpoint |
Akers et al., 2006
|
|
Energy content and viscosity of biofuels |
Wagner et al., 2010
|
|
Density measurements using a Galilean thermometer |
Priest et al., 2011
|
Direct application of the founding Twelve Principles in a practical environment is now so straightforward that it makes little sense for educators to avoid “greening” their experiments. Most contemporary students have a heightened sense of environmental responsibility, and consider it important to be taught about modern approaches towards laboratory sustainability (Kitchens et al., 2006). They are, for example, initially surprised that water is an excellent solvent for many organic reactions, having often heard the opposite during lecture classes. After learning about “traditional” textbook reaction solvents such as dichloromethane and acetonitrile, they embrace performing reactions under solventless conditions. A novel green laboratory teaching approach has been adopted where data generation and interpretation leads to development of a principle statement (Teixeira et al., 2010). Students are guided to devise a more sustainable solvent system for Grignard reactivity based upon their own acquired data and that provided to them. A one-term, integrated project for the undergraduate organic laboratory involves synthesis of Nylon 6,6 from cyclohexanol (Dintzner et al., 2012). The product of one reaction is used as a starting material for the following one in an effort to minimize waste, and students are exposed to several other green principles in the context of a familiar target compound.
A third-year undergraduate catalytic chemistry course (“Organic Synthesis Techniques”) has been designed at the University of Toronto where green chemistry is a thread running through both lecture and laboratory components (Dicks and Batey, 2012). A number of novel pedagogical experiments featuring catalysis are incorporated and listed in Table 5, along with additional green aspects of each reaction. For each system, students undertake metric analysis (calculation of experimental atom economies and waste production). This becomes especially instructive when “traditional” and “modern” approaches towards the same Biginelli reaction are employed (Aktoudianakis et al., 2009). The traditional Biginelli reaction involves heating an aromatic aldehyde, ethyl acetoacetate and urea with mineral acid catalyst in ethanol solvent for 90 min to form a dihydropyrimidone. In comparison, the modern solventless method utilizes ZnCl2 as the catalyst, reducing the heating time to 20 min. This addresses two of the Twelve Principles: “use of auxiliary substances should be made unnecessary wherever possible” and “energy requirements of chemical processes should be recognized and should be minimized” (Anastas and Warner, 1998). Each student performs both procedures within one laboratory session so that quantitative comparison between the reactions is straightforward.
Table 5 Green reactivity features in Organic Synthesis Techniques
Reaction |
Catalytic approach |
Additional green features |
Ref. |
Williamson ether synthesis |
phase-transfer catalysis |
aqueous reactivity |
Vanden Eynde and Mailleux, 2001
|
Verley-Doebner condensation |
organocatalysis |
— |
Stabile and Dicks, 2003
|
Biginelli reaction |
Brønsted acid catalysis |
high atom economy, reduction in solvent usage |
Aktoudianakis et al., 2009
|
Biginelli reaction |
Lewis acid catalysis |
high atom economy, solvent-free reactivity, reduced energy requirements |
Aktoudianakis et al., 2009
|
Primary alcohol oxidation |
transition metal catalysis (Ru(VII)) |
mild conditions, short reaction time |
Koroluk et al., 2011
|
Suzuki reaction |
transition metal catalysis (Pd) |
aqueous reactivity & purification solvent |
Aktoudianakis et al., 2008
|
Heck reaction |
transition metal catalysis (Pd(II)) |
aqueous reactivity & purification solvent |
Cheung et al., 2007
|
The impact of being trained to critically evaluate transformations from a green perspective is apparent towards the end of Organic Synthesis Techniques. Each class member is assigned an individualized target compound to synthesize in two laboratory periods from a given starting material. They are encouraged to generally employ green chemistry principles, adopting catalytic measures where possible. Students are responsible for all aspects of planning and synthesis execution and routinely incorporate a number of sustainable strategies (e.g. using solvents derived from biomass and greener reagents, solvent-free reactivity, reactions under mild conditions) (Dicks and Batey, 2012).
5. Different “shades of green”
The term “shades of green” has been applied to describe the variable environmental friendliness of different reaction solvents (Clark and Tavener, 2007). This is instructive terminology to share with undergraduates, who are much more familiar (and comfortable) with a “correct” answer to a chemical problem. It should be emphasized that applying green chemistry principles to a system is a comparative exercise and not an absolute one, and that it is not “all or nothing” (Kerr and Brown, 2009; Bennett, 2010). An asymptotic approach has been outlined where students consider the following three questions after performing any laboratory work (Goodwin, 2004): (i) “what was green about the experiment?”; (ii) “what was not green?”; (iii) “how could the experiment be made greener?”. It is certainly the case that “all that glitters is not green” in the chemical research literature—a point elaborated on in the “inaccurate assertions of “greenness”” section concluding this article.
6. Retooling introductory general and organic courses
In order to facilitate the introduction of either a new green chemistry course or separate units into a number of different offerings, some important curricular issues should be addressed. Firstly, it is ideal if the instructor charged with designing and implementing a green course is the same one who teaches at least one introductory prerequisite course. A need exists to integrate important principles into first-year general chemistry courses, particularly dealing with stoichiometry discussions. An attempt to achieve this in the context of some introductory exercises has been published (Song et al., 2004). Also, both lectures and laboratories associated with such courses are ideal for discussing the principles of process atom economy and E-factor. Secondly, there are specific items in introductory organic chemistry courses (typically taught in second-year) that require revision and/or amendment. These include the following major points:
• how to consistently balance chemical equations, indicating the presence of all organic and inorganic products
• how to clearly represent organic molecules on paper/using chemical drawing software, keeping the same structural aspect throughout a synthetic pathway
• how to locate the starting materials of a chemical synthesis in the desired product structure
• making it known to students from the outset the complete classification of organic reaction types: substitutions, rearrangements, redox reactions, additions (includes couplings, multi-component reactions, cyclizations, condensations), and eliminations
• how to identify nucleophilic/electrophilic sites in any kind of chemical structure
• how to identify BrØnsted-type and Lewis-type acidic/basic sites in any kind of chemical structure
• establishing connections between correctly balancing an equation, pinpointing starting materials in the target product structure and constructing a reasonable curved arrow mechanism for every new reaction taught.
Payoffs of green chemistry thinking
There are major advantages afforded to undergraduates by inclusion of green principles into their educational experience. As most materials have been developed for organic courses, three important skills typically acquired from them are outlined below with specific examples.
• Deeper analysis and richer discussion of synthesis performances by comparative analysis. In a third-year undergraduate Industrial and Green Chemistry course, students analyze multiple synthesis plans towards three synthetic targets: ibuprofen, pravadoline and a dihydrodiazepine (Andraos, 2012(a)). Each synthesis plan is taken from the primary chemical literature. Each example illustrates the ubiquitous problem that, for most of the time, no one plan has all the “right” fully optimized characteristics in every category. By exposing students to such well-documented real-world scenarios they begin to appreciate the difficulties involved in reaction optimization. They become able to directly link that the endeavours of optimization and “greening” are one and the same – a point emphasized by Laird’s editorial (Laird, 2012). They also discover that because chemistry is an experimental science governed by compromise, optimization inevitably involves prioritization of variables. They additionally learn that metrics are important tools to verify claims of greenness made in the literature and to proofread experimental procedures.
• Critical evaluation of merits and bottlenecks in synthesis plans, consequently offering creative solutions that can address the shortcomings. In the same course, students undertake innovative problem set questions covering various aspects of synthesis optimization. These include reaction network analysis, cost analysis, energy input analysis, comparison of linear, convergent, and divergent strategies, green metrics analysis for material efficiency and probability analysis based on target thresholds for reaction “greenness”. They suggest multiple synthesis plans for a single target molecule given various constraints simulating real-world situations, including availability of source starting materials and threshold limits on atom economies for reactions and the overall proposed synthesis plans (Andraos, 2012(a)).
• Increased memory retention of fundamental concepts learned, particularly if real-world industrial chemistry examples are discussed. A nice illustrative example is the three industrial syntheses of beta-carotene, a food colourant, which highlights the discovery and application of three fundamental organic reactions that students learn about in introductory organic chemistry courses: the Wittig reaction (BASF synthesis) (Nuerrenbach et al., 1977), the hydrogenation of alkynes to cis-olefins using Lindlar’s catalyst (Hoffmann-LaRoche synthesis) (Isler et al., 1947; Inhoffen et al., 1950; Isler et al., 1956; Surmatis and Ofner, 1961), and the McMurry coupling of ketones to olefins (McMurry and Fleming, 1974). Telling students the background story behind the discovery of these reactions not only helps them remember the reactions, but also illustrates the circumstances, motivation, and mechanics of how real research is done in an industrial setting.
Conclusions—areas for improvement in teaching and research
1. Inaccurate assertions of “greenness”
A symbiotic relationship exists between research and teaching aspects of any academic discipline. The next generation of researchers “learn their trade” in the undergraduate classroom and modern teaching practices are shaped by current research trends. As mentioned earlier, there are many false claims of “greenness” in the chemical literature. Often an argument is made on the basis of only one of the Twelve Principles. This represents a narrow view rather than a multivariate global approach which considers all material and energy consumption, and the environmental impact of any waste produced. An example here is the oft-quoted declaration of a “solventless” reaction. In the vast majority of cases, this only applies to no reaction solvent being used—it does not include or take into account any solvents used in product work-up or purification operations (Dicks, 2009(a)). A “dry-laboratory” teaching approach has been reported where students focus on the impact of auxiliary substances such as extraction solvents and separation agents as sources of waste after a solvent-free organic transformation (Van Arnum, 2005).
In a similar fashion, just because a reaction is run in water or in an ionic liquid does not make it inevitably “green”, and simply because a reaction begins with a biofeedstock does not make it automatically “sustainable”. Interestingly, the journal Green Chemistry has revised its editorial policy on accepting papers for publication on the subject of ionic liquids precisely because of false advertisements of ionic liquids as “green solvents” (Welton, 2011). Multi-component reactions (MCRs) and “one-pot” reactions are not necessarily “green”—even though they generally proceed with high atom economy, they may require toxic reagents/solvents or chromatographic separation of products. A similar situation exists for reactions performed under catalytic conditions. The tetrapropylammonium perruthenate (TPAP) catalysis of primary alcohol oxidation to an aldehyde has been undertaken and analyzed by undergraduates within the context of the Twelve Principles (Koroluk et al., 2011). Student laboratory reports reflect the understanding that simply because a reaction employs a catalyst, it is not automatically a perfectly green process. A recent statistical survey of over 1400 such reactions published up to 2010 as part of a larger evaluation of a synthesis database showed that 54% of them would satisfy simultaneously the basic criteria of kernel reaction mass efficiencies (excluding all solvents and auxiliary materials) exceeding 60% and reaction yields exceeding 80% (Andraos, 2011(b)). These kinds of reality checks compel educators to ensure that students learn to adopt a balanced modus operandi when assessing “greenness”.
2. Attention to experimental detail
The itemization of experimental procedural details needs to dramatically improve in journal articles and patents, if any reliable estimates of waste production analysis are to be made. There is often casual reporting of quantities of materials used in work-up and purification procedures (e.g. volumes of all extraction solvents, volumes of chromatographic packing materials, volumes of elution solvents, masses of drying agents, masses of gaseous reagents). Reaction yields may not be quoted or may be incorrectly calculated. The frequency of revealing reaction performance in patents is lower than in journal articles, which impacts on the metrics evaluation of good industrial examples taken from the patent literature to illustrate green chemistry ideas. Evaluation of plans and reactions should include the synthesis of ionic liquid solvents and specialized catalysts or ligands, as these are routinely omitted from the main synthesis evaluation. Often the structure of a specialized catalyst involves a more complicated synthesis than the target product that the catalyst is designed to help generate. This lack of detail severely hampers the decision-making process between the relative “greenness” of two or more synthetic routes. Biocatalytic transformations are generally poorly documented in terms of the needs of a chemist to evaluate reaction performance. Reaction yields are often reported as molar yields with respect to the substrate of interest, which may or may not be the limiting reagent. Stoichiometrically balanced equations may not be possible to write down for such reactions since the chemistries of the transformations may not be fully known.
3. Energy and environmental considerations
The assessment of energy consumption is not routinely disclosed as part of the standard protocol in reporting experimental procedures. This becomes particularly problematic for proper evaluation of microwave-based chemical transformations against traditional heating methods since these are often quoted as greener than conventional procedures, with little convincing hard evidence. Often papers quote some fragmented combination of power consumption in watts, reaction time, or temperature of reaction without accounting for the intrinsic efficiency of the microwave apparatus used. This state of affairs makes it difficult to determine the actual energy consumption. The commonly-held belief that these kinds of reactions are energy efficient has been recently challenged (Razzaq and Kappe, 2008). Additionally, reliable environmental impact metrics data (which are important for life cycle analysis) are often difficult to find since they may be scattered widely in the literature, or are found in unfamiliar sources to most chemists. This impacts the incorporation of such metrics in the evaluation of synthesis routes beyond material consumption. An attempt has been made to rectify this situation in the context of a holistic problems-based assignment for undergraduate students in a combined third-year green and environmental chemistry course taught at Queen’s University, Canada (Mercer et al., 2012).
4. Pedagogical gaps
If one revisits the Twelve Principles, certain gaps become apparent in the pedagogical literature. Although catalytic reactivity has been heavily exploited in student laboratories, there are relatively few examples of catalysts being effectively recycled and reused (Edward, 2012). Recent academic research has focused on improving this situation (Fan et al., 2002; Trindade et al., 2009; Zeng et al., 2009; Zeng et al., 2010; Zeng et al., 2011), and the hope is that approaches such as these will filter into undergraduate curricula. Similarly, organic chemistry students are routinely taught about the essential need for protecting groups during certain transformations. There is currently a great deal of interest in devising new protecting group-free syntheses (Hoffmann, 2006; Young and Baran, 2009; Roulland, 2011), which will require novel reagents and reactivity. An extension of the concept of atom economy to include “redox economy” as part of the evaluation of synthesis strategy has also gained attention (Burns et al., 2009; Newhouse et al., 2009).
Though water is the most often used reagent in organic synthesis for protonation, hydration, and hydrolysis reactions, and is also the medium of choice for work-up washes, it is not universally included in cost calculations of input materials in a synthesis plan. Research into increasing its use as a reaction solvent for a wide variety of organic reactions is gaining traction in the undergraduate laboratory curriculum (Dicks, 2009(b); Sauer, 2012). Widespread success in these and associated fields may lead to a re-writing of undergraduate textbooks as a paradigm shift evolves (Tucker, 2010). The emerging field of continuous flow reactors to carry out reactions instead of traditional round-bottomed flasks promises to revolutionize the scope of reaction possibilities including control of thermodynamic and kinetic products by changing flow rates of reagents (Ley and Baxendale, 2002(a), 2002(b); Ley and Baxendale, 2008; Wiles and Watts, 2008; Yoshida et al., 2008; Yoshida, 2010; Glasnov and Kappe, 2011; Yoshida et al., 2011). However, there has not yet been any demonstration of this technique in an undergraduate laboratory setting, nor has there been any reported substantive evidence of reduction in solvent demand by metrics evaluation. Finally, as mentioned previously, quantification of energy consumption and costs for chemical reactions has received little attention from both research and teaching perspectives (Gronnow et al., 2005).
Summary
In this paper we have reviewed approaches towards green chemistry education with the aim of highlighting effective instructional strategies as well as ongoing challenges. As instructors in this exciting field we have gained greater personal satisfaction in teaching and appreciation for the subject of chemistry. Particularly satisfying from this work is the accomplishments we have seen in the students we have had the privilege to teach over the last decade. It is clear that much work remains to be done to incorporate green chemistry into the accepted mainstream education of future chemists. We hope that instructors reading this account will be inspired to join this worthy enterprise.
Notes and references
- Adams R. and Voorhees V., (1921), Furfural, Org. Synth. Vol. 1, 49–52.
- Akers S. M., Conkle J. L., Thomas S. N. and Rider K. B., (2006), Determination of the heat of combustion of biodiesel using bomb calorimetry. A multidisciplinary undergraduate chemistry experiment, J. Chem. Educ., 83, 260–262.
- Aktoudianakis E., Chan E., Edward A. R., Jarosz I., Lee V., Mui L., Thatipamala S. S. and Dicks A. P., (2008), “Greening up” the Suzuki reaction, J. Chem. Educ., 85, 555–557.
- Aktoudianakis E., Chan E., Edward A. R., Jarosz I., Lee V., Mui L., Thatipamala S. S. and Dicks A. P., (2009), Comparing the traditional with the modern - a greener, solvent-free dihydropyrimidone synthesis, J. Chem. Educ., 86, 730–732.
- Amato I., (1993), The slow birth of green chemistry, Science, 259, 1538–1541.
- Anastas P., (2011), Twenty years of green chemistry, Chem. Eng. News, 89(26), 62–65.
- Anastas P. T. and Warner J. C., (1998), Green chemistry: theory and practice. New York: Oxford University Press.
- Andraos J. and Izhakova J., (2006), Perspective on the application of green chemistry principles to total synthesis design, Chimica Oggi., 24, 31–36.
- Andraos J. and Sayed M., (2007), On the use of “green” metrics in the undergraduate organic chemistry lecture and lab to assess the mass efficiency of organic reactions, J. Chem. Educ., 84, 1004–1010.
- Andraos J., (2012(a)), Designing a green organic chemistry lecture course, in Dicks A. P. (ed.), Green organic chemistry in lecture and laboratory (pp. 29–68). Boca Raton, FL: CRC Press.
- Andraos J., (2011(b)), A database tool for process chemists and chemical engineers to gauge the material and synthetic efficiencies of synthesis plans to industrially important targets, Pure Appl. Chem., 83, 1361–1378.
- Armenta S. and de la Guardia M., (2011), Determination of mercury in milk by cold vapor atomic fluorescence: a green analytical chemistry laboratory experiment, J. Chem. Educ., 88, 488–491.
- Bennett G. D., (2010), Green chemistry as an expression of environmental ethics, in Sharma, S. K. and Mudhoo, A. (ed.), Green chemistry for environmental sustainability (p. 116). Boca Raton, FL: CRC Press.
- Bica K., Gaertner P. and Rogers R. D., (2011), Ionic liquids and fragrances - direct isolation of orange essential oil, Green Chem., 13, 1997–1999.
- Biginelli P., (1893), Aldureides of ethylic acetoacetate and ethylic oxalacetate, Gazz. Chim. Ital., 23, 360–416.
- Boatman E. M., Lisensky G. C. and Nordell K. J., (2005), A safer, easier, faster synthesis for CdSe quantum dot nanocrystals, J. Chem. Educ., 82, 1697–1699.
- Breslow R., (1958), Mechanism of thiamine action. IV. Evidence from studies on model systems, J. Am. Chem. Soc., 80, 3719–3726.
- Burns N. Z., Baran P. S. and Hoffmann R. W., (2009), Redox economy in organic synthesis, Angew. Chem. Int. Ed., 48, 2854–2867.
- Cacciatore K. L. and Sevian H., (2006), Teaching lab report writing through inquiry: a green chemistry stoichiometry experiment for general chemistry, J. Chem. Educ., 83, 1039–1041.
- Cacciatore K. L., Amado J., Evans J. J. and Sevian H., (2008), Connecting solubility, equilibrium, and periodicity in a green, inquiry experiment for the general chemistry laboratory, J. Chem. Educ., 85, 251–253.
- Calloway N. O., (1935), The Friedel–Crafts syntheses, Chem. Rev., 17, 327–392.
- Calvo-Flores F. G., (2009), Sustainable chemistry metrics, ChemSusChem, 2, 905–919.
- Canal J. P., (2009), An inorganic green chemistry experiment: the preparation and analysis of Group II metal oxalate hydrates, Chem. Educator, 14, 26–29.
- Cann M. C., (1999), Bringing state-of-the-art, applied, novel, green chemistry to the classroom by employing the Presidential Green Chemistry Challenge awards, J. Chem. Educ., 76, 1639–1641.
- Cann M. C. and Connelly M. E., (2000), Real-world cases in green chemistry. Washington, DC: American Chemical Society.
- Cann M. C. and Dickneider T. A., (2004), Infusing the chemistry curriculum with green chemistry using real-world examples, web modules, and atom economy in organic chemistry courses, J. Chem. Educ., 81, 977–980.
- Cheung L. L. W., Aktoudianakis E., Chan E., Edward A. R., Jarosz I., Lee V., Mui L., Thatipamala S. S. and Dicks A. P., (2007), A microscale Heck reaction in water, Chem. Educator, 12, 77–79.
- Clark J. H. and Tavener S. J., (2007), Alternative solvents: shades of green, Org. Process Res. Dev., 11, 149–155.
- Clark R. A., Stock A. E. and Zovinka E. P., (2012), Metalloporphyrins as oxidation catalysts: moving toward “greener” chemistry in the inorganic chemistry laboratory, J. Chem. Educ., 89, 271–275.
- Collins T., (1995), Introducing green chemistry in teaching and research, J. Chem. Educ., 72, 965–966.
- Cope A. C., (1937), Condensation reactions. I. The condensation of ketones with cyanoacetic esters and the mechanism of the Knoevenagel reaction, J. Am. Chem. Soc., 59, 2327–2330.
- Correia P. R. M., Siloto R. C., Cavicchioli A., Oliveira P. V. and Rocha F. R. P., (2004), Green analytical chemistry in undergraduate laboratories: flow-injection determination of creatinine in urine with photochemical treatment of waste, Chem. Educator, 9, 242–246.
- Dicks A. P., (2009(a)), Solvent-free reactivity in the undergraduate organic laboratory, Green Chem. Lett. Rev., 2, 87–100.
- Dicks A. P., (2009(b)), A review of aqueous organic reactions for the undergraduate teaching laboratory, Green Chem. Lett. Rev., 2, 9–21.
- Dicks A. P., (2012), Green organic chemistry in lecture and laboratory (ed.), Boca Raton, FL: CRC Press.
- Dicks A. P. and Batey R. A., (2012), Greening the organic curriculum: development of an undergraduate catalytic chemistry course, J. Chem. Educ., submitted for publication.
- Dintzner M. R., Kinzie C. R., Pulkrabek K. and Arena A. F., (2012), The cyclohexanol cycle and synthesis of Nylon 6,6: green chemistry in the undergraduate organic laboratory, J. Chem. Educ., 89, 262–264.
- Djerassi C. and Scholz C. R., (1948), Brominations with pyridine hydrobromide perbromide, C5H5N·HBr·Br2,J. Am. Chem. Soc., 70, 417–418.
- Doebner O., (1900), Synthesis of sorbic acid, Chem. Ber., 33, 2140–2142.
- Doxsee K. M. and Hutchison J. E., (2004), Green organic chemistry—strategies, tools, and laboratory experiments. Pacific Grove, CA: Brooks/Cole.
- Dunn P. G., Galvin S. and Hettenbach K., (2004), The development of an environmentally benign synthesis of sildenafil citrate (Viagra™) and its assessment by green chemistry metrics, Green Chem., 6, 43–48.
- Edward A. R., (2012), Organic waste management and recycling, in Dicks A. P. (ed.), Green organic chemistry in lecture and laboratory (pp. 199–224). Boca Raton, FL: CRC Press.
- Fan Q. H., Li Y. M. and Chan A. S. C., (2002), Recoverable catalysts for asymmetric synthesis, Chem. Rev.102, 3385–3466.
- Gaudin P., Jacquot R., Marion P., Pouilloux P. and Jérôme F., (2011), Acid-catalyzed etherification of glycerol with long-alkyl-chain alcohols, ChemSusChem, 4, 719–722.
- Giokas D. L., Paleologos E. K. and Karayannis M. I., (2003), Micelle-mediated extraction of heavy metals from environmental samples: an environmental green chemistry laboratory experiment, J. Chem. Educ., 80, 61–64.
- Glasnov T. N. and Kappe C. O., (2011), Continuous-flow syntheses of heterocycles, J. Heterocycl. Chem., 48, 11–30.
- Goodwin T. E., (2004), An asymptotic approach to the development of a green organic chemistry laboratory, J. Chem. Educ., 81, 1187–1190.
- Grant S., Freer A. A., Winfield J. M., Gray C., Overton T. L. and Lennon D., (2004), An undergraduate teaching exercise that explores contemporary issues in the manufacture of titanium dioxide on the industrial scale, Green Chem., 6, 25–32.
- Grant S., Freer A. A., Winfield J. M., Gray C. and Lennon D., (2005), Introducing undergraduates to green chemistry: an interactive teaching exercise, Green Chem., 7, 121–128.
- Gron L. U., (2009), Green analytical chemistry: application and education, in Anastas P. T., Levy I. J. and Parent K. E. (ed.), Green chemistry education: changing the course of chemistry, Am. Chem. Soc. Symp. Ser., 1011, pp. 103–116.
- Gronnow M. J., White R. J., Clark J. H. and Macquarrie D. J., (2005), Energy efficiency in chemical reactions: a comparative study of different reaction techniques, Org. Process Res. Dev., 9, 516–518.
- Haack J. A., Hutchison J. E., Kirchhoff M. M. and Levy I. J., (2005), Going green: lecture assignments and lab experiences for the college curriculum, J. Chem. Educ., 82, 974–976.
- Hantzsch A., (1882), Synthesis of pyridine derivatives from ethyl acetoacetate and aldehydammonia, Liebigs Ann. Chem., 215, 1–82.
- Henderson R. K., Constable D. J. C. and Jiménez-González C., (2010), Green chemistry metrics, in Dunn P. J., Wells A. S. and Williams M. T. (ed.), Green chemistry in the pharmaceutical industry (pp. 24–25). Weinheim, Germany: Wiley-VCH.
- Hess G., (2010), Industry drops flame retardant, Chem. Eng. News, 88(1), 10.
- Hoffmann R. W., (2006), Protecting-group-free synthesis, Synthesis, 3531–3541.
- Houri A. and Wehbe H., (2003), Towards an environmentally friendly chemistry laboratory: managing expired chemicals, Green Chem., 5, G49–G50.
- Huheey J. E., (1996), Incorporating environmental issues into the inorganic curriculum, in Anastas P. T. and Williamson T. C. (ed.), Green Chemistry: Designing Chemistry for the Environment, Am. Chem. Soc. Symp. Ser., 626, 232–238.
- Iacobucci S., Jaworek-Lopes C., Wang P., Phun L., Wilbur G. and Dobson A., (2006), A green, clean colligative properties experiment, Chem. Educator, 11, 102–104.
- Inhoffen H. H., Bohlmann F., Bartram K., Rummert G. and Pommer H., (1950), Synthesen in der carotinoid-reihe. 15. Uber die darstellung von trans und von 9,9′-mono-cis-beta-carotin, Ann. Chem., 570, 54–69.
- Isler O., Huber W., Ronco A. and Kofler M., (1947), Synthese des vitamin-A, Helv. Chim. Acta, 30, 1911–1927.
- Isler O., Lindlar H., Montavon M., Ruegg R. and Zeller P., (1956), Synthesen in der carotinoid-reihe. 1. Die technische synthese von beta-carotin, Helv. Chim. Acta, 39, 249–259.
- Jiménez-González C., Ponder C. S., Broxterman Q. B. and Manley J. B., (2011), Using the right green yardstick: why process mass intensity is used in the pharmaceutical industry to drive more sustainable processes, Org. Process Res. Dev., 15, 912–917.
- Kerr M. E. and Brown D. M., (2009), Using green chemistry to enhance faculty professional development opportunities, in Anastas P. T., Levy, I. J. and Parent K. E. (ed.), Green chemistry education: changing the course of chemistry, Am. Chem. Soc. Symp. Ser., 1011, p. 27.
- Kirchhoff M. and Ryan, M. A., (ed.), (2002), Greener approaches to undergraduate chemistry experiments. Washington, DC: American Chemical Society.
- Kitchens C., Charney R., Naistat D., Farrugia J., Clarens A., O’Neil A., Lisowski C. and Braun B., (2006), Completing our education. Green chemistry in the curriculum, J. Chem. Educ., 83, 1126–1129.
- Klingshirn M. A., Wyatt A. F., Hanson R. M. and Spessard G. O., (2008), Determination of the formula of a hydrate: a greener alternative, J. Chem. Educ., 85, 819–821.
- Koroluk K. J., Skonieczny S. and Dicks A. P., (2011), Microscale catalytic and chemoselective TPAP oxidation of geraniol, Chem. Educator, 16, 307–309.
- Laird, T., (2012), Green chemistry is good process chemistry, Org. Process Res. Dev., 16, 1–2.
- Lea M. C., (1892), Disruption of silver halides by mechanical force, Am. J. Sci., 43, 527–531.
- Lennon D., Freer A. A., Winfield J. M., Landon P. and Reid N., (2002), An undergraduate teaching initiative to demonstrate the complexity and range of issues typically encountered in modern industrial chemistry, Green Chem., 4, 181–187.
- Ley S. V. and Baxendale I. R., (2002(a)), New tools and concepts for modern organic synthesis, Nature Rev. Drug Disc., 1, 573–586.
- Ley S. V. and Baxendale I. R., (2002(b)), Organic synthesis in a changing world, The Chemical Record, 2, 377–388.
- Ley S. V. and Baxendale I. R., (2008), The changing face of organic synthesis, Chimia, 62, 162–168.
- Manske R. H. F., (1949), Process of preparing 2,4-dichlorophenoxyacetic acid, US 2471575, New York, US Rubber Co.
- Marteel-Parrish A. E., (2007), Toward the greening of our minds: a new special topics course, J. Chem. Educ., 84, 245–247.
- Martin C. B., Schmidt M. and Soniat M., (2011), A survey of the practices, procedures, and techniques in undergraduate organic chemistry teaching laboratories, J. Chem. Educ., 88, 1630–1638.
- Martinez C. A., Hu S., Dumond Y., Tao J., Kelleher P. and Tully L., (2008), Development of a chemoenzymatic manufacturing process for pregabalin, Org. Process Res. Dev., 12, 392–398.
- Matlack A., (1999), Teaching green chemistry, Green Chem., 1, G19–G20.
- McCarthy S. M. and Gordon-Wylie S. W., (2005), A greener approach for measuring colligative properties, J. Chem. Educ., 82, 116–119.
- McKenzie B. F., (1929), Levulinic acid, Org. Synth.9, 50–51.
- McKenzie L. C., Huffman L. M., Parent K. E., Hutchison J. E. and Thompson J. E., (2004), Patterning self-assembled monolayers on gold. Green materials chemistry in the teaching laboratory, J. Chem. Educ., 81, 545–548.
- McKenzie L. C., Thompson J. E., Sullivan R. and Hutchison J. E., (2004), Green chemical processing in the teaching laboratory: a convenient liquid CO2 extraction of natural products, Green Chem., 6, 355–358.
- McMurry J., (2012), Organic chemistry, 8th ed., Belmont, CA, Thomson Higher Education, p. 982.
- McMurry J. E. and Fleming M. P., (1974), New method for reductive coupling of carbonyls to olefins - synthesis of beta-carotene, J. Am. Chem. Soc., 96, 4708–4709.
- Mercer S. M., Andraos J. and Jessop P. G., (2012), Choosing the greenest synthesis: a multivariate metric green chemistry exercise, J. Chem. Educ., 89, 215–220.
- Newhouse T., Baran P. S. and Hoffmann R. W., (2009), The economies of synthesis, Chem. Soc. Rev., 38, 3010–3021.
- Nuerrenbach A., Paust J., Pommer H., Schneider J. and Schulz B., (1977), Hydroperoxide oxidation of phosphorus ylides - new and useful route to symmetrical carotenoids, Ann. Chem., 1146–1159.
- Olson E. J. and Buhlmann P., (2010), Minimizing hazardous waste in the undergraduate analytical laboratory: a microcell for electrochemistry, J. Chem. Educ., 87, 1260–1261.
- Parker L. H., (1914), Reactions by trituration, J. Chem. Soc. Trans., 105, 1504–1516.
- Pawar P. M., Jarag K. J. and Shankarling G. S., (2011), Environmentally benign and energy efficient methodology for condensation: an interesting facet to the classical Perkin reaction, Green Chem., 13, 2130–2134.
- Pottevin H., (1906), Reversible diastatic effects. Formation and decomposition of esters under the influence of the diastase pancreas, Bull. Soc. Chim. Fr., 35, 693–696.
- Priest M. A., Padgett L. W. and Padgett C. W., (2011), Demonstrating the temperature dependence of density via construction of a Galilean thermometer, J. Chem. Educ., 88, 983–985.
- Ranke J., Bahadir M., Eissen M. and Koenig B., (2008), Developing and disseminating NOP: an online, open-access, organic chemistry teaching resource to integrate sustainability concepts in the laboratory, J. Chem. Educ., 85, 1000–1005.
- Raston C. L. and Scott J. L., (2001), Teaching green chemistry. Third-year-level module and beyond, Pure Appl. Chem., 73, 1257–1260.
- Razzaq T. and Kappe C. O., (2008), On the energy efficiency of microwave-assisted organic reactions, ChemSusChem1, 123–132.
- Reed S. M. and Hutchison J. E., (2000), Green chemistry in the organic teaching laboratory: an environmentally benign synthesis of adipic acid, J. Chem. Educ., 77, 1627–1629.
- Rekha V. V., Ramani M. V., Ratnamala A., Rupakalpana V., Subbaraju G. V., Satyanarayana C. and Someswara Rao C., (2009), A simple, efficient, green, cost effective and chemoselective process for the esterification of carboxylic acids, Org. Process Res. Dev., 13, 769–773.
- Ribeiro M. T. C., Costa D. A. and Machado A. A. S. C., (2010), “Green Star”: a holistic green chemistry metric for evaluation of teaching laboratory experiments, Green. Chem. Lett. Rev., 3, 149–159.
- Ribeiro M. G. T. C. and Machado A. A. S. C., (2011), Metal-acetylacetonate synthesis experiments: which is greener?, J. Chem. Educ., 88, 947–953.
- Richards W. T. and Loomis A. L., (1927), The chemical effects of high frequency sound waves I. A preliminary survey, J. Am. Chem. Soc., 49, 3086–3100.
- Richardson A., Janiec A., Chan B. C. and Crouch R. D., (2006), Synthesis of silver nanoparticles: an undergraduate laboratory using a green approach, Chem. Educator, 11, 331–333.
- Ritter S. K., (2011(a)), Presidential Green Chemistry Academic Award: Bruce H. Lipshutz, University of California, Santa Barbara, http://pubs.acs.org/cen/news/89/i26/8926notw1b.html (accessed Aug 2011).
- Ritter S. K., (2011(b)), Presidential Green Chemistry Greener Reaction Conditions Award: Kraton Performance Polymers, Houston, http://pubs.acs.org/cen/news/89/i26/8926notw1f.html (accessed Aug 2011).
- Ritter S. K., (2011(c)), Presidential Green Chemistry Greener Synthetic Pathways Award: Genomatica, San Diego, http://pubs.acs.org/cen/news/89/i26/8926notw1e.html (accessed Aug 2011).
- Roesky H. and Kennepohl D., (ed.), (2009), Experiments in green and sustainable chemistry. Weinheim: Wiley-VCH.
- Rosenthaler L., (1908), Enzyme-effected asymmetric syntheses, Biochem. Z., 14, 238–253.
- Roulland E., (2011), Protecting-group-free total syntheses: a challenging approach, Angew. Chem. Int. Ed., 50, 1226–1127.
- Sauer E. L. O., (2012), Organic reactions under aqueous conditions, in Dicks, A. P. (ed.), Green organic chemistry in lecture and laboratory (pp. 103–130). Boca Raton, FL: CRC Press.
- Sharma R. K., Sharma C. and Sidhwani I. T., (2011), Solventless and one-pot synthesis of Cu(II) phthalocyanine complex: a green chemistry experiment, J. Chem. Educ., 88, 86–87.
- She J.-L. and Liu R.-S., (2008), A simplified synthetic experiment of YBa2Cu3O7−x superconductor for first-year chemistry laboratory, J. Chem. Educ., 85, 825–826.
- Sidhwani I. T. and Chowdhury S., (2008), Greener alternative to qualitative analysis for cations without H2S and other sulfur-containing compounds, J. Chem. Educ., 85, 1099–1101.
- Sims P. A., Branscum K. M., Kao L. and Keaveny V. R., (2010), An inexpensive, relatively green, and rapid method to purify genomic DNA from Escherichia coli: an experiment for the undergraduate biochemistry laboratory, J. Chem. Educ., 87, 1113–1115.
- Smith J. G., (2011), Organic chemistry (3rd ed., pp. 450–451). New York, NY: McGraw-Hill.
- Song Y., Wang Y. and Geng Z., (2004), Some exercises reflecting green chemistry concepts, J. Chem. Educ., 81, 691–692.
- Stabile R. G. and Dicks A. P., (2003), Two-step semi-microscale preparation of a cinnamate ester sunscreen analog, J. Chem. Educ., 81, 1488–1491.
- Surmatis J. D. and Ofner A., (1961), A new synthesis of trans-beta-carotene and decapreno-beta-carotene, J. Org. Chem., 26, 1171–1173.
- Tavener S., Hardy J., Hart N. and Goddard A., (2003), Teaching green chemistry: from lemons to lemonade bottles, Green Chem., 5, G46–G48.
- Teixeira J. M., Nedrow Byers J., Perez M. G. and Holman R.W., (2010), The question-driven laboratory exercise: a new pedagogy applied to a green modification of Grignard reagent formation and reaction, J. Chem. Educ., 87, 714–716.
- Trindade A. F., Gois P. M. P. and Afonso C. A. M., (2009), Recyclable stereoselective catalysts, Chem. Rev., 109, 418–514.
- Tucker J. L., (2010), Green chemistry: cresting a summit toward sustainability, Org. Process Res. Dev., 14, 328–331.
- Uffelman E. S., Doherty J. R., Schultze C., Burke A. L., Bonnema K. R., Watson T. T. and Lee III D. W., (2004(a)), Microscale syntheses, reactions, and 1H NMR spectroscopic investigations of square planar macrocyclic tetraamido-N Cu(III) complexes relevant to green chemistry, J. Chem. Educ., 81, 182–185.
- Uffelman E. S., Doherty J. R., Schultze C., Burke A. L., Bonnema K. R., Watson T. T. and Lee III D. W., (2004(b)), Microscale syntheses, reactions, and 1H NMR spectroscopic investigations of square planar macrocyclic tetraamido-N Co(III) complexes relevant to green chemistry, J. Chem. Educ., 81, 325–329.
- Van Aken K., Strekowski L. and Patiny L., (2006), EcoScale, a semi-quantitative tool to select an organic preparation based on economical and ecological parameters, Beilstein J. Org. Chem., 2, no. 3.
- Van Arnum S. D., (2005), An approach towards teaching green chemistry fundamentals, J. Chem. Educ., 82, 1689–1692.
- Vanden Eynde J. J. and Mailleux I., (2001), Quaternary ammonium salt-assisted organic reactions in water: alkylation of phenols, Synth. Commun., 31, 1–7.
- Verley A., (1899), Condensation of the citral with malonic acid, Bull. Soc. Chim. Fr., 21, 414–418.
- Voith M., (2010), Seeking to cement a green future, Chem. Eng. News, 88(4), 20–21.
- Vollhardt P. and Schore N., (2011), Organic chemistry structure and function (6th ed., p. 103). New York, NY: W. H. Freeman.
- Wagner E. P., Koehle M. A., Moyle T. M. and Lambert P. D., (2010), How green is your fuel? Creation and comparison of automotive biofuels, J. Chem. Educ., 87, 711–713.
- Walden P., (1914), Molecular weights and electrical conductivity of several fused salts, Bull. Acad. Imper. Sci. (St. Petersburg), 405–422.
- Warburg O., (1906), Cleavage of the leucine esters by pancreas ferment, Z. Physiol. Chem., 48, 205–213.
- Warner M. G., Succaw G. L. and Hutchison J. E., (2001), Solventless syntheses of mesotetraphenylporphyrin: new experiments for a greener organic chemistry laboratory curriculum, Green Chem., 3, 267–270.
- Welton T., (2011), Ionic liquids in Green Chemistry, Green Chem., 13, 225.
- Wiles C. and Watts P., (2008), Continuous flow reactors, a tool for the modern synthetic chemist, Eur. J. Org. Chem., 1655–1671.
- Winterton N., (2001), Twelve more green chemistry principles, Green Chem., 3, G73–G75.
- Wöhler F., (1828), Ueber künstliche Bildung des Harnstoffs, Ann. Phys., 88, 253–256.
- Yoshida J., Nagaki A. and Yamada T., (2008), Flash chemistry: fast chemical synthesis by using microreactors, Chem. Eur. J., 14, 7450–7459.
- Yoshida J., (2010), Flash chemistry: fast organic synthesis, in Microsystems. New York, NY: Wiley.
- Yoshida J., Kim H. and Nagaki A., (2011), Green and sustainable chemical synthesis using flow microreactors, ChemSusChem, 4, 331–340.
- Young I. S. and Baran P. S., (2009), Protecting-group-free synthesis as an opportunity for invention, Nat. Chem., 1, 193–205.
- Zeng T., Song G. and Li C.-J., (2009), Separation, recovery and reuse of N-heterocyclic carbene catalysts in transesterification reactions, Chem. Commun., 6249–6251.
- Zeng T., Chen W-W., Cirtiu C. M., Moores A., Song G. and Li C.-J., (2010), Fe3O4 nanoparticles, a robust and magnetically recoverable catalyst for three-component coupling of aldehyde, alkyne and amine, Green Chem., 12, 570–573.
- Zeng T., Yang L., Hudson R., Song G., Moores A. R. and Li C.-J., (2011), Fe3O4 nanoparticle-supported copper(I) pybox catalyst: magnetically recoverable catalyst for enantioselective direct-addition of terminal alkynes to imines, Org. Lett., 13, 442–445.
Footnote |
† This article is part of a themed issue on sustainable development and green chemistry in chemistry education. |
|
This journal is © The Royal Society of Chemistry 2012 |
Click here to see how this site uses Cookies. View our privacy policy here.