DOI:
10.1039/D1QO01320C
(Research Article)
Org. Chem. Front., 2021,
8, 6663-6669
Wanzlick's equilibrium in tri- and tetraaminoolefins†
Received
5th September 2021
, Accepted 13th October 2021
First published on 14th October 2021
Abstract
The dissociation mechanism of electron-rich olefins into their parent carbenes has been a controversial topic since Wanzlick's pioneering work. Herein, we present a combined synthetic and computational study on the formation (dissociation, respectively) of hetero- and homo-carbene dimers derived from benzimidazolin-2-ylidenes (benzNHCs), imidazolidin-2-ylidenes (saNHC), and cyclic (alkyl) (amino) carbenes (CAACs) through sublimation (in vacuo) as well as in condensed phase. We quantify the effect of proton catalysis and report that even triaminoolefins dissociate to their free carbenes, yet only under proton catalysis. Accordingly, we report how the judicious choice of the base (KOtBu vs. KHMDS) and solvent (hexane/benzene vs. THF) allows N,N′-dimethylbenzimidazolin-2-ylidene to be obtained quantitatively as a metastable, kinetic product. This free carbene had been previously reported to dimerize directly to the olefin-dimer, which is the thermodynamic product.
Electron-rich olefins serve as valuable organic materials and reagents.1 This is arguably due to the presence of three stable redox states.2–4 Thereby, the radical cations5,6 show peculiar photo-chemical and conductive properties.7–10 In their neutral, reduced redox state, they are of use as organic redox agents.11–15 Nitrogen-containing derivatives such as enetetramines (tetraaminoolefins, respectively) 1 are dimers of N-heterocyclic carbenes (NHCs) 2.16 While the dimerization is unfavorable for unsaturated (“conventional”) NHCs,17–19 more π-acidic carbenes dimerize.20 On the contrary to carbene chemistry, where dimerization is undesired, it is required to control dissociation of their dimers in organic electronics. One approach, albeit synthetically tedious, is the installation of tethers.21 Alternatively, steric and electronic effects may control the dissociation/association equilibrium. However, there has been a long debate on the mechanistic intricacies surrounding potential involvement of proton catalysis. Already in the 1960s, Wanzlick proposed that free carbenes form through direct dissociation of the enetetramine (Scheme 1).22–24 Later, Lemal and Winberg reconsidered the suggested equilibrium and emphasized the importance of proton catalysis involving transient 3 due to the requirement for the addition of catalytic amounts of proton sources, such as mineral acids.25,26 This was also observed by Arduengo for stable thiazol-2-ylidenes which only dimerized upon the presence of thiazolium salts.27 Almost 40 years later, Alder showed that 3 is integral to the formation of 1.28,29 Murphy reported on tethered olefins, where dissociation occurred likely through proton catalysis.30 Denk showed that NHCs dimerize slowly under aprotic conditions.31 Further, he presented evidence for carbene metathesis (“crossover”) between different enetetramines with small N-substituents (e.g.Me1, R = Me and Et1, R = Et) in solution under heating.32,33 Hahn and Lemal revealed benzimidazolin-2-ylidene formation upon heating the respective dimers in solution using in situ1H NMR spectroscopic studies.34–37 These results were further corroborated by computations.38–41 To the best of our knowledge, experimental investigations during sublimation (i.e. gas phase), devoid of the serendipitous presence of protons as is the case in solution in glassware remain hitherto elusive.42 Furthermore, studies on the dissociation of triaminoolefins remain to be reported. Thus, we communicate herein on the sublimation of heterodimers43 derived from benzNHCs, saNHCs, and CAACs as well as their protonated derivatives equivalent to Lemal's intermediate 3.44 Complemented by computations, we quantify the effect of proton-catalysis and show how to avoid/slow down the undesired dimerization of transient carbenes (dissociation of electron-rich olefins, respectively).
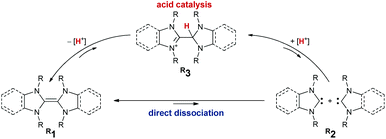 |
| Scheme 1 Acid catalysis in the equilibrium between tetraaminoolefins 1 and NHC monomers 2. | |
Synthesis
It has been reported that deprotonation of benzimidazolium salt Me5 by KOtBu gives dimer Me6 (Scheme 2, right).45 To our surprise, when using KHMDS [KN(SiMe3)2] as a base in benzene at room temperature, we obtained instead a mixture of the dimer (44%, Fig. S1†) and the free carbene Me4 (56%, Scheme 2, left). The latter, unambiguously identified through a signal in the 13C NMR spectrum at 218 ppm, proved kinetically surprisingly stable with a half-life t1/2 of more than one day (c = 0.4 M) and consequently required several days to dimerize with a pseudo-second order rate law (Fig. S8†). Following the deprotonation of a suspension of Me5 by KOtBu in C6D6, however, indeed corroborated the instantaneous and quantitative formation of Me6. These observations suggest that HOtBu (pKa = 16.5)46 catalyzes the dimerization, whereas less acidic HN(SiMe3)2 (pKa = 25.8)47 does not (or to a much lesser extent, respectively). Indeed, adding small amounts of HOtBu to the free carbene Me4 led to the immediate and quantitative formation of Me6. In contrast, free carbene Me4 could be obtained quantitatively by adding Me5 to KHMDS dissolved in a 1
:
1 mixture of benzene and hexane at 5 °C under moderately dilute conditions (c = 0.04 M; ≈3% dimerization after one day at room temperature; Fig. S3 and S4†). Even removing the solvent, i.e. isolation of the free carbene, led to only ≈4% dimerization (Fig. S5†). The deprotonation by lithium diisopropylamide (LDA) in benzene formed quantitatively the lithium complex (δcarbene = 207 ppm), which converted by ≈2% to the dimer within three days (c = 0.4 M; Fig. S6 and S7†).
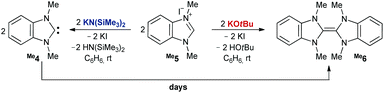 |
| Scheme 2 The rate for the formation of 6 from 5 is dependent on the base. | |
To further elucidate the dimerization (dissociation, respectively) mechanism, we synthesized a series of carbene heterodimers48 with varying steric and electronic properties (Scheme 3, top). Following our previously reported route for the formation of Me10viaMe9,43 we obtained Et9 (61%) and Et10 (86%) analytically pure. Salts R9 represent the triamino-equivalent of Lemal's transient intermediate 3. Conversely, the synthesis of the saNHC
benzNHC tetraaminoolefins R13 (Scheme 3, bottom) proved more challenging.
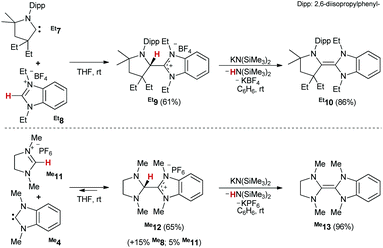 |
| Scheme 3 The CAAC-derived protonated heterodimer Et9 and olefins Et10, Me13 do not dissociate in solution at room temperature (top), whereas saNHC-derived Me12 stands in equilibrium with the starting materials Me4 and Me11. | |
In the case of salt Me12, the in situ1H NMR spectroscopic analysis in DMSO indicated that the reaction leveled out at approximately 80% conversion after 1 h, with the concomitant presence of imidazolinium- (Me11, 5%) and benzimidazolium (Me8, 15%) salt (Fig. S14†). In fact, after prolonged reaction time, we observed the slow formation of Me13. In case of Et13, we even did not manage to obtain useful conversion (>5%) to Et12. Overall, these results are consistent with the rare reports28,49–51 of protonated enetetramines (“Lemal's intermediate”) and suggest that not only the addition of Me4 to Me11 proceeds essentially isoergic, but equally the protonation of Me13. Still, treatment of the mixture with KHMDS afforded, after workup, olefin Me13 in satisfactory purity (Fig. S16†).
Sublimation experiments
Upon heating the triaminoolefins Me10 and Et10in vacuo to 185 °C and 165 °C, respectively, yellow crystals formed at the walls of the sublimation flask (Scheme 4).
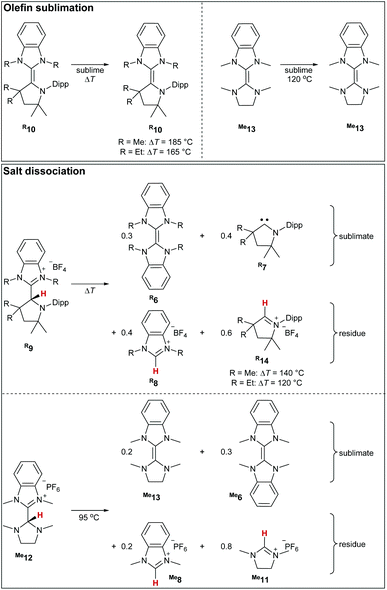 |
| Scheme 4 Whereas sublimation was observed for the electron-rich olefins R10 and Me13 (top), dissociation and subsequent separation of volatile and non-volatile products were obtained for their protonated congeners R9 and Me12 (bottom). All experiments were conducted at 5 × 10−3 mbar. | |
The 1H NMR spectroscopic analysis identified these crystals as the starting material without the concomitant formation of Me6 (Fig. S18†). In addition, no metathesis occurred when heating a mixture of Me10, and Et10 (Fig. S22†). We conclude that such triaminoolefins do not dissociate at these temperatures in the absence of proton catalysis. This seems to be also the case for enetetramine Me13, where only starting material was obtained in the sublimate after heating it to 120 °C. In case of the salts Me9, Et9, and Me12, dissociation and subsequent sublimation of homo- and heterodimers occurred at considerably lower temperatures (140 °C, 120 °C, 95 °C) than found for the olefins (Scheme 4, bottom). Accordingly, the presence of free carbene, as well as homodimers, confirms dissociation. For instance, in case of Me9, the sublimed material consisted of the homodimers and the free CAAC, respectively (Me6: 30%; Me7: 40%), whereas the residue consisted of both benzimidazolium- (Me8: 40%) as well as cyclic iminium (Me14: 60%) salt. Overall, we conclude that both tetra- and triaminoolefins dissociate readily under proton catalysis on gentle heating.
Computational analysis
Computational investigations at the DLPNO-CCSD(T)/def2-TZVPP//BLYP-D3BJ/def2-TZVPP level of theory were performed. Consistent with the experiment, where no dissociation was observed at temperatures as high as 185 °C, the dissociation of triaminoolefin Me10 is predicted to occur with a high barrier of ΔG≠ = +200 kJ mol−1 (Fig. 1, top) and considerably endergonic (ΔG = +76 kJ mol−1). The barrier for ethyl derivative Et10 is significantly lower with ΔG≠ = +172 kJ mol−1, yet still too high to form Et4 and Et7 (ΔG = +53 kJ mol−1) under mild conditions. Tetraaminoolefin Me13 is expected to dissociate with ΔG≠ = +146 kJ mol−1 (ΔG = +55 kJ mol−1), which seems consistent with dissociation starting at a (slightly) higher temperature than the sublimation temperature of 120 °C. Also here, the introduction of ethyl groups (Et13) further facilitates dissociation (ΔG≠ = +138 kJ mol−1; ΔG = +43 kJ mol−1).
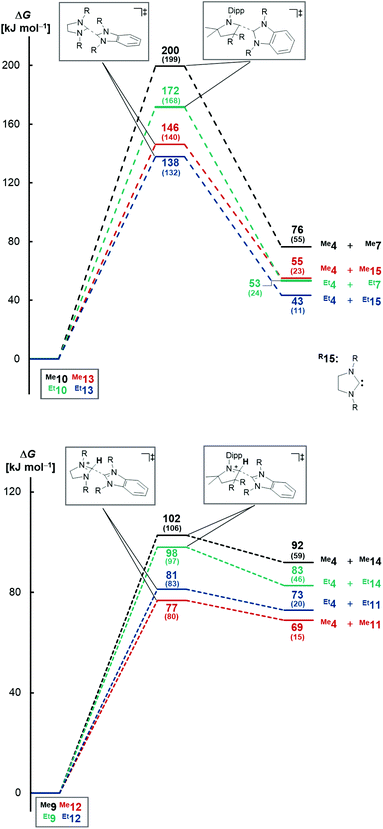 |
| Fig. 1 Calculations at the DLPNO-CCSD(T)/def2-TZVPP//BLYP-D3BJ/def2-TZVPP level of theory quantify acid catalysis for dissociation of tri- and tetraaminoolefins. Values in parentheses relate to implicit solvation in THF. | |
In contrast, the dissociation from the salts, representing the key elementary step of proton catalysis, requires lower activation energies (Fig. 1, bottom). Whereas the Gibbs free activation energy for Me9 (ΔG≠ = +102 kJ mol−1) and Et9 (ΔG≠ = +98 kJ mol−1) suggests dissociation at elevated temperatures, the value of ΔG≠ = +77 kJ mol−1 (ΔG = +69 kJ mol−1) found for saNHC–benzNHC salts Me12 and ΔG≠ = +81 kJ mol−1 (ΔG = +73 kJ mol−1) for Et12 is indicative for dissociation at milder temperatures. Modeling the dissociation in condensed phase using implicit solvation in THF confirms that the dissociation of Me12 (ΔG = +15 kJ mol−1) and Et12 (ΔG = +20 kJ mol−1) proceeds almost isoergic in solution with low barriers (Me12, ΔG≠ = +80 kJ mol−1; Et12, ΔG≠ = +83 kJ mol−1), consistent with a reaction occurring at room temperature. Salts Me9 and Et9 are kinetically (Me9, ΔG≠ = +106 kJ mol−1; Et9, ΔG≠ = +97 kJ mol−1) and thermodynamically (Me9, ΔG = +59 kJ mol−1; Et9, ΔG = +46 kJ mol−1) more stable. These results in agreement with the experimental difficulties to isolate Me12 in analytically pure form (vide supra). Overall, we conclude that proton catalysis lowers the activation energy for dissociation for the methyl-substituted enetetramine by ΔΔG≠ = 146 kJ mol−1–77 kJ mol−1 = 69 kJ mol−1 (ΔΔG = 138 kJ mol−1–81 kJ mol−1 = 57 kJ mol−1 for the ethyl-substituted derivative) and in case of the methyl-functionalized triaminoolefin by ΔΔG = 200 kJ mol−1–102 kJ mol−1 = 98 kJ mol−1 (ΔΔG = 172 kJ mol−1–98 kJ mol−1 = 74 kJ mol−1 for the ethyl-substituted derivative).
Conclusion
The dissociation of electron-rich olefins into carbenes was studied in the absence of serendipitous proton sources. To this goal, representatives of tri- and tetraaminoolefins composed of NHCs and CAACs, and their protonated congeners, alleged key intermediates in a proton-catalysis pathway, were synthesized. Based on sublimation at elevated temperatures as well as the computational analysis, we quantified proton-catalysis and showed that not only tetraaminoolefins, but as well triaminoolefins may dissociate. However, acid catalysis is crucial in case of the latter, whereas the former dissociate under heating potentially as well through a direct mechanism. Eventually, we outlined how N,N′-dimethylbenzimidazolin-2-ylidene, previously reported to dimerize instantaneously, can be isolated through deprotonation with KN(SiMe3)2 in a mixture of benzene and hexane. This free carbene turned out to be metastable over the course of days, whereas weaker bases such as KOtBu lead to direct dimer formation. Thus, this work serves as a guideline on how to generate transient carbenes and engineer thermodynamically and kinetically stable electron rich olefins being of use in organic electronics.
Author contributions
The project idea was conceived by D.M., who also directed all work and wrote the manuscript. J.M. performed the computations, contributed to the synthesis, and wrote the first draft. M. K. synthesized all compounds and performed the sublimation experiments. S.G. performed exploratory synthetic studies.
Conflicts of interest
There are no conflicts to declare.
Acknowledgements
We thank the RRZ Erlangen for computational resources. We also thank K. Meyer for his support.
Notes and references
- M. Bendikov, F. Wudl and D. F. Perepichka, Tetrathiafulvalenes, Oligoacenenes, and Their Buckminsterfullerene Derivatives: The Brick and Mortar of Organic Electronics, Chem. Rev., 2004, 104, 4891–4946 CrossRef CAS PubMed.
- R. W. Hoffmann, Reactions of Electron-Rich Olefins, Angew. Chem., Int. Ed. Engl., 1968, 7, 754–765 CrossRef CAS.
- J. Hocker and R. Merten, Reactions of Electron-Rich Olefins with Proton-Active Compounds, Angew. Chem., Int. Ed. Engl., 1972, 11, 964–973 CrossRef CAS.
- M. F. Lappert, The coordination chemistry of electron-rich alkenes (enetetramines), J. Organomet. Chem., 1988, 358, 185–213 CrossRef CAS.
- J. Messelberger, A. Grünwald, S. J. Goodner, F. Zeilinger, P. Pinter, M. E. Miehlich, F. W. Heinemann, M. M. Hansmann and D. Munz, Aromaticity and sterics control whether a cationic olefin radical is resistant to disproportionation, Chem. Sci., 2020, 11, 4138–4149 RSC.
- Y. Kim and E. Lee, Stable Organic Radicals Derived from N-Heterocyclic Carbenes, Chem. – Eur. J., 2018, 24, 19110–19121 CrossRef CAS PubMed.
- R. J. Mortimer, Electrochromic materials, Chem. Soc. Rev., 1997, 26, 147–156 RSC.
- N. Martin, Tetrathiafulvalene: the advent of organic metals, Chem. Commun., 2013, 49, 7025–7027 RSC.
- D. Canevet, M. Salle, G. Zhang, D. Zhang and D. Zhu, Tetrathiafulvalene (TTF) derivatives: key building-blocks for switchable processes, Chem. Commun., 2009, 2245–2269 RSC.
- J. Messelberger, A. Grünwald, P. Pinter, M. M. Hansmann and D. Munz, Carbene derived diradicaloids-building blocks for singlet fission?, Chem. Sci., 2018, 9, 6107–6117 RSC.
- J. Broggi, T. Terme and P. Vanelle, Organic electron donors as powerful single-electron reducing agents in organic synthesis, Angew. Chem., Int. Ed., 2014, 53, 384–413 CrossRef CAS PubMed.
- J. A. Murphy, Discovery and Development of Organic Super-Electron-Donors, J. Org. Chem., 2014, 79, 3731–3746 CrossRef CAS PubMed.
- E. Doni and J. A. Murphy, Evolution of neutral organic super-electron-donors and their applications, Chem. Commun., 2014, 50, 6073–6087 RSC.
- S. Rohrbach, R. S. Shah, T. Tuttle and J. A. Murphy, Neutral Organic Super Electron Donors Made Catalytic, Angew. Chem., Int. Ed., 2019, 58, 11454–11458 CrossRef CAS PubMed.
- Y. Zhao, M. Rollet, L. Charles, G. Canard, D. Gigmes, P. Vanelle and J. Broggi, Switching from single to simultaneous free radical and anionic polymerization with enamine-based organic electron donors, Angew. Chem., 2021, 60, 19389–19396 CrossRef CAS PubMed.
- For concise and recent reviews on carbenes and carbene ligands, see:
(a) M. N. Hopkinson, C. Richter, M. Schedler and F. Glorius, An overview of N-heterocyclic carbenes, Nature, 2014, 510, 485–496 CrossRef CAS PubMed;
(b) R. S. Ghadwal, Carbon-based two electron σ-donor ligands beyond classical N-heterocyclic carbenes, Dalton Trans., 2016, 45, 16081–16095 RSC;
(c) D. Munz, Pushing Electrons-Which Carbene Ligand for Which Application?, Organometallics, 2018, 37, 275–289 CrossRef CAS;
(d) P. Bellotti, M. Koy, M. N. Hopkinson and F. Glorius, Recent advances in the chemistry and applications of N-heterocyclic carbenes, Nat. Rev. Chem., 2021, 5, 711–725 CrossRef CASFor a comprehensive review on carbenes in main group chemistry, see:
(e) V. Nesterov, D. Reiter, P. Bag, P. Frisch, R. Holzner, A. Porzelt and S. Inoue, NHCs in Main Group Chemistry, Chem. Rev., 2018, 118, 9678–9842 CrossRef CAS PubMedFor thematic issues and books on NHCs, see:
(f) A. J. Arduengo III and G. Bertrand, Carbenes Introduction, Chem. Rev., 2009, 109, 3209–3210 CrossRef PubMed;
(g)
S. Díez González, N-Heterocyclic Carbenes: From Laboratory Curiosities to Efficient Synthetic Tools, Royal Society of Chemistry, Cambridge, UK, 2010 RSC;
(h) T. Rovis and S. P. Nolan, Stable Carbenes: From ‘Laboratory Curiosities’ to Catalysis Mainstays, Synlett, 2013, 1188–1189 CrossRef CAS;
(i)
S. P. Nolan, N-Heterocyclic Carbenes: Effective Tools for Organometallic Synthesis, Wiley-VCH, Weinheim, Germany, 2014 Search PubMed;
(j)
H. V. Huynh, The Organometallic Chemistry of N-heterocyclic Carbenes, John Wiley & Sons, Hoboken, New Jersey, USA, 2017 CrossRef;
(k) F. E. Hahn, Introduction: Carbene Chemistry, Chem. Rev., 2018, 118, 9455–9456 CrossRef CAS PubMed. For a leading review with a focus on cyclic singlet carbenes, see:
(l) M. Melaimi, M. Soleilhavoup and G. Bertrand, Stable Cyclic Carbenes and Related Species beyond Diaminocarbenes, Angew. Chem., Int. Ed., 2010, 49, 8810–8849 CrossRef CAS PubMed. For a visual tutorial on isolating free carbenes and heterodimers, see:
(m) A. Grünwald, S. J. Goodner and D. Munz, Isolating Free Carbenes, their Mixed Dimers and Organic Radicals, J. Visualized Exp., 2019, e59389 Search PubMed.
- A. J. Arduengo III, R. L. Harlow and M. Kline, A stable crystalline carbene, J. Am. Chem. Soc., 1991, 113, 361–363 CrossRef.
- R. P. Thummel, V. Goulle and B. Chen, Bridged derivatives of 2,2′-biimidazole, J. Org. Chem., 1989, 54, 3057–3061 CrossRef CAS.
- Z. Shi and R. P. Thummel, Bridged bibenzimidazolium salts and their conversion to ureaphanes, Tetrahedron Lett., 1994, 35, 33–36 CrossRef CAS.
- A. J. Arduengo III, J. R. Goerlich and W. J. Marshall, A stable diaminocarbene, J. Am. Chem. Soc., 1995, 117, 11027–11028 CrossRef.
- T. A. Taton and P. Chen, A stable tetraazafulvalene, Angew. Chem., Int. Ed. Engl., 1996, 35, 1011–1013 CrossRef CAS.
- H. W. Wanzlick and E. Schikora, Ein Neuer Zugang Zur Carben-Chemie, Angew. Chem., Int. Ed. Engl., 1960, 72, 494–494 CrossRef CAS.
- H. W. Wanzlick and E. Schikora, Ein Nucleophiles Carben, Chem. Ber./Recl., 1961, 94, 2389–2393 CrossRef CAS.
- H. W. Wanzlick, Aspects of Nucleophilic Carbene Chemistry, Angew. Chem., Int. Ed. Engl., 1962, 1, 75–80 CrossRef.
- D. M. Lemal, R. A. Lovald and K. I. Kawano, Tetraaminoethylenes. The Question of Dissociation, J. Am. Chem. Soc., 1964, 86, 2518–2519 CrossRef CAS.
- H. E. Winberg, J. E. Carnahan, D. D. Coffman and M. Brown, Tetraaminoethylenes, J. Am. Chem. Soc., 1965, 87, 2055–2056 CrossRef CAS.
- A. J. Arduengo III, J. R. Goerlich and W. J. Marshall, A Stable Thiazol-2-ylidene and Its Dimer, Liebigs Ann., 1997, 365–374 CrossRef.
- R. W. Alder, L. Chaker and F. P. V. Paolini, Bis(diethylamino)carbene and the mechanism of dimerisation for simple diaminocarbenes, Chem. Commun., 2004, 2172–2173 RSC.
- R. W. Alder, M. E. Blake, L. Chaker, J. N. Harvey, F. Paolini and J. Schütz, When and How Do Diaminocarbenes Dimerize?, Angew. Chem., Int. Ed., 2004, 43, 5896–5911 CrossRef CAS PubMed.
- P. I. Jolly, S. Zhou, D. W. Thomson, J. Garnier, J. A. Parkinson, T. Tuttle and J. A. Murphy, Imidazole-derived carbenes and their elusive tetraazafulvalene dimers, Chem. Sci., 2012, 3, 1675–1679 RSC.
- M. K. Denk, A. Thadani, K. Hatano and A. J. Lough, Steric Stabilization of Nucleophilic Carbenes, Angew. Chem., Int. Ed. Engl., 1997, 36, 2607–2609 CrossRef CAS.
- M. K. Denk, K. Hatano and M. Ma, Nucleophilic carbenes and the wanzlick equilibrium: A reinvestigation, Tetrahedron Lett., 1999, 40, 2057–2060 CrossRef CAS.
- Y. Liu and D. M. Lemal, Concerning the “Wanzlick equilibrium”, Tetrahedron Lett., 2000, 41, 599–602 CrossRef CAS.
- Y. Liu, P. E. Lindner and D. M. Lemal, Thermodynamics of a Diaminocarbene−Tetraaminoethylene Equilibrium, J. Am. Chem. Soc., 1999, 121, 10626–10627 CrossRef CAS.
- F. E. Hahn, L. Wittenbecher, D. Le Van and R. Fröhlich, Evidence for an Equilibrium between an N-heterocyclic Carbene and Its Dimer in Solution, Angew. Chem., Int. Ed., 2000, 39, 541–544 CrossRef CAS PubMed.
- V. P. W. Böhm and W. A. Herrmann, The “Wanzlick Equilibrium”, Angew. Chem., Int. Ed., 2000, 39, 4036–4038 CrossRef.
- J. W. Kamplain and C. W. Bielawski, Dynamic covalent polymers based upon carbene dimerization, Chem. Commun., 2006, 1727–1729 RSC.
- K. Młodzikowska, A. A. Rajkiewicz, K. Grela and B. Trzaskowski, Boron–boron, carbon–carbon and nitrogen–nitrogen bonding in N-heterocyclic carbenes and their diazaboryl and triazole analogues: Wanzlick equilibrium revisited, New J. Chem., 2018, 42, 6183–6190 RSC.
- M.-J. Cheng, C.-L. Lai and C.-H. Hu, Theoretical study of the Wanzlick equilibrium, Mol. Phys., 2004, 102, 2617–2621 CrossRef CAS.
- A. Poater, F. Ragone, S. Giudice, C. Costabile, R. Dorta, S. P. Nolan and L. Cavallo, Thermodynamics of N-Heterocyclic Carbene Dimerization: The Balance of Sterics and Electronics, Organometallics, 2008, 27, 2679–2681 CrossRef CAS.
- D. C. Graham, K. J. Cavell and B. F. Yates, Dimerization mechanisms of heterocyclic carbenes, J. Phys. Org. Chem., 2005, 18, 298–309 CrossRef CAS.
- For a mass spectrometry and computational study that suggests Lemal's intermediate to be preferred over the hydrogen bonded dimer C–H⋯:C in case of saturated NHCs and almost isoergic for imidazolin-derived aromatic NHCs, see:
(a) M. Paul, E. Detmar, M. Schlangen, M. Breugst, J.-M. Neudörfl, H. Schwarz, A. Berkessel and M. Schäfer, Intermediates of N-Heterocyclic Carbene (NHC) Dimerization Probed in the Gas Phase by Ion Mobility Mass Spectrometry: C−H⋯:C Hydrogen Bonding Versus Covalent Dimer Formation, Chem. – Eur. J., 2019, 25, 2511–2518 CrossRef CAS PubMed. For the hydrogen bonded dimer C–H⋯:C, see:
(b) A. J. Arduengo III, S. F. Gamper, M. Tamm, J. C. Calabrese, F. Davidson and H. A. Craig, A Bis(carbene)-Proton Complex: Structure of a C-H-C Hydrogen Bond, J. Am. Chem. Soc., 1995, 117, 572–573 CrossRef.
- D. Munz, J. Chu, M. Melaimi and G. Bertrand, NHC–CAAC Heterodimers with Three Stable Oxidation States, Angew. Chem., Int. Ed., 2016, 55, 12886–12890 CrossRef CAS PubMed.
- For the first report of a CAAC, see:
(a) V. Lavallo, Y. Canac, C. Präsang, B. Donnadieu and G. Bertrand, Stable Cyclic (Alkyl)(Amino)Carbenes as Rigid or Flexible, Bulky, Electron-Rich Ligands for Transition-Metal Catalysts: A Quaternary Carbon Atom Makes the Difference, Angew. Chem., Int. Ed., 2005, 44, 5705–5709 CrossRef CAS PubMed. For reviews on CAACs, see:
(b) M. Soleilhavoup and G. Bertrand, Cyclic (Alkyl)(Amino)Carbenes (CAACs): Stable Carbenes on the Rise, Acc. Chem. Res., 2015, 48, 256–266 CrossRef CAS PubMed;
(c) S. Roy, K. C. Mondal and H. W. Roesky, Cyclic Alkyl(amino) Carbene Stabilized Complexes with Low Coordinate Metals of Enduring Nature, Acc. Chem. Res., 2016, 49, 357–369 CrossRef CAS PubMed;
(d) M. Melaimi, R. Jazzar, M. Soleilhavoup and G. Bertrand, Cyclic (Alkyl)(amino)carbenes (CAACs): Recent Developments, Angew. Chem., Int. Ed., 2017, 56, 10046–10068 CrossRef CAS PubMed;
(e) U. S. D. Paul and U. Radius, What Wanzlick Did Not Dare To Dream: Cyclic (Alkyl)(amino)carbenes (cAACs) as New Key Players in Transition-Metal Chemistry, Eur. J. Inorg. Chem., 2017, 3362–3375 CrossRef CAS;
(f) R. Jazzar, M. Soleilhavoup and G. Bertrand, Cyclic (Alkyl)- and (Aryl)-(amino)carbene Coinage Metal Complexes and Their Applications, Chem. Rev., 2020, 120, 4141–4168 CrossRef CAS PubMed. For the synthesis of donor substituted CAACs, see:
(g) J. Chu, D. Munz, R. Jazzar, M. Melaimi and G. Bertrand, Synthesis of Hemilabile Cyclic (Alkyl)(amino)carbenes (CAACs) and Applications in Organometallic Chemistry, J. Am. Chem. Soc., 2016, 138, 7884–7887 CrossRef CAS PubMed.
- This finding was reproduced in THF:
(a) G. Tintori, A. Fall, N. Assani, Y. Zhao, D. Bergé-Lefranc, S. Redon, P. Vanelle and J. Broggi, Generation of powerful organic electron donors by water-assisted decarboxylation of benzimidazolium carboxylates, Org. Chem. Front., 2021, 8, 1197–1205 RSC.
- W. Reeve, C. M. Erikson and P. F. Aluotto, A new method for the determination of the relative acidities of alcohols in alcoholic solutions. The nucleophilicities and competitive reactivities of alkoxides and phenoxides, Can. J. Chem., 1979, 57, 2747–2754 CrossRef CAS.
- R. R. Fraser, T. S. Mansour and S. Savard, Acidity measurements on pyridines in tetrahydrofuran using lithiated silylamines, J. Org. Chem., 1985, 50, 3232–3234 CrossRef CAS.
- For recent and leading examples on carbene derived heterodimers, see:
(a) A. J. Arduengo III, F. Davidson, H. V. R. Dias, J. R. Goerlich, D. Khasnis, W. J. Marshall and T. K. Prakasha, An Air Stable Carbene and Mixed Carbene “Dimers”, J. Am. Chem. Soc., 1997, 197, 12742–12749 CrossRef;
(b) P. W. Antoni and M. M. Hansmann, Pyrylenes: A New Class of Tunable, Redox-Switchable, Photoexcitable Pyrylium–Carbene Hybrids with Three Stable Redox-States, J. Am. Chem. Soc., 2018, 140, 14823–14835 CrossRef PubMed;
(c) P. W. Antoni, T. Bruckhoff and M. M. Hansmann, Organic Redox Systems Based on Pyridinium-Carbene Hybrids, J. Am. Chem. Soc., 2019, 141, 9701–9711 CrossRef CAS PubMed;
(d) D. Mandal, R. Dolai, N. Chrysochos, P. Kalita, R. Kumar, D. Dhara, A. Maiti, R. S. Narayanan, G. Rajaraman, C. Schulzke, V. Chandrasekhar and A. Jana, Stepwise Reversible Oxidation of N-Peralkyl-Substituted NHC–CAAC Derived Triazaalkenes: Isolation of Radical Cations and Dications, Org. Lett., 2017, 19, 5605–5608 CrossRef CAS PubMed;
(e) D. Mandal, R. Dolai, R. Kumar, S. Suhr, N. Chrysochos, P. Kalita, R. S. Narayanan, G. Rajaraman, C. Schulzke, B. Sarkar, V. Chandrasekhar and A. Jana, Influence of N-Substitution on the Formation and Oxidation of NHC–CAAC-Derived Triazaalkene, J. Org. Chem., 2019, 84, 8899–8909 CrossRef CAS PubMed;
(f) M. K. Nayak, S. Suhr, N. Chrysochos, H. Rawat, C. Schulzke, V. Chandrasekhar, B. Sarkar and A. Jana, Tethered CAAC–CAAC dimers: oxidation to persistent radical cations and bridging-unit dependent reactivity/stability of the dications, Chem. Commun., 2021, 57, 1210–1213 RSC;
(g) J. Stubbe, S. Suhr, J. Beerhues, M. Nößler and B. Sarkar, The transformations of a methylene-bridged bis-triazolium salt: a mesoionic carbene based metallocage and analogues of TCNE and NacNac, Chem. Sci., 2021, 12, 3170–3178 RSC. For electrochemical studies on hetero- as well as homodimers, see:
(h) J. R. Ames, M. A. Houghtaling, D. L. Terrian and T. P. Mitchell, Annulated derivatives of 2, 2′-biimidazole, 2-(2′-imidazolyl) benzimidazole, and 2, 2′-bibenzimidazole, Can. J. Chem., 1997, 75, 28–36 CrossRef CAS. For a tethered heterodimer, see:
(i) S. Simonovic, J.-C. Frison, H. Koyuncu, A. C. Whitwood and R. E. Douthwaite, Addition of N-Heterocyclic Carbenes to Imines: Phenoxide Assisted Deprotonation of an Imidazolium Moiety and Generation of Breslow Intermediates Derived from Imines, Org. Lett., 2009, 11, 245–247 CrossRef CAS PubMed. For heterodimers derived from NHCs and aldehydes (Breslow intermediates), see:
(j) A. Berkessel, S. Elfert, V. R. Yatham, J.-M. Neudörfl, N. E. Schlörer and J. H. Teles, Umpolung by N-heterocyclic carbenes: generation and reactivity of the elusive 2,2-diamino enols (Breslow intermediates), Angew. Chem., Int. Ed., 2012, 51, 12370–12374 CrossRef CAS PubMed;
(k) A. Berkessel, V. R. Yatham, S. Elfert and J.-M. Neudörfl, Characterization of the Key Intermediates of Carbene-Catalyzed Umpolung by NMR Spectroscopy and X-Ray Diffraction: Breslow Intermediates, Homoenolates, and Azolium Enolates, Angew. Chem., Int. Ed., 2013, 52, 11158–11162 CrossRef CAS PubMed;
(l) J. Rehbein, S. M. Ruser and J. Phan, NHC-catalysed benzoin condensation - is it all down to the Breslow intermediate?, Chem. Sci., 2015, 6, 6013–6018 RSC. For carbene dimers separated by arene spacers, see:
(m) M. M. Hansmann, M. Melaimi, D. Munz and G. Bertrand, Organic Mixed Valence Compounds Derived from Cyclic (Alkyl)(amino)carbenes, J. Am. Chem. Soc., 2018, 140, 2546–2554 CrossRef CAS PubMed;
(n) G. Kundu, S. De, S. Tothadi, A. Das, D. Koley and S. S. Sen, Saturated N–Heterocyclic Carbene Based Thiele's Hydrocarbon with a Tetrafluorophenylene Linker, Chem. – Eur. J., 2019, 25, 16533–16537 CrossRef CAS PubMed;
(o) A. Maiti, J. Stubbe, N. I. Neuman, P. Kalita, P. Duari, C. Schulzke, V. Chandrasekhar, B. Sarkar and A. Jana, CAAC-Based Thiele and Schlenk Hydrocarbons, Angew. Chem., 2020, 59, 6729–6734 CrossRef CAS PubMed;
(p) A. Maiti, S. Chandra, B. Sarkar and A. Jana, Acyclic diaminocarbene-based Thiele, Chichibabin, and Müller hydrocarbons, Chem. Sci., 2020, 11, 11827–11833 RSC.
- M. F. Lappert, S. Álvarez, G. Aullón, R. Fandos, A. Otero, A. Rodríguez, S. Rojas and P. Terreros, Reactivity of a super-electron-rich olefin derived from cyclam, Eur. J. Inorg. Chem., 2009, 1851–1860 CrossRef CAS.
- U. S. D. Paul and U. Radius, Ligand versus Complex: C−F and C−H Bond Activation of Polyfluoroaromatics at a Cyclic (Alkyl)(Amino)Carbene, Chem. – Eur. J., 2017, 23, 3993–4009 CrossRef CAS PubMed.
- Y. T. Chen and F. Jordan, Reactivity of the thiazolium C2 ylide in aprotic solvents: novel experimental evidence for addition rather than insertion reactivity, J. Org. Chem., 1991, 56, 5029–5038 CrossRef CAS.
Footnotes |
† Electronic supplementary information (ESI) available. See DOI: 10.1039/d1qo01320c |
‡ Both authors contributed equally. |
|
This journal is © the Partner Organisations 2021 |
Click here to see how this site uses Cookies. View our privacy policy here.