DOI:
10.1039/C8DT03443E
(Paper)
Dalton Trans., 2018,
47, 16938-16943
Full compositional control of PbSxSe1−x thin films by the use of acylchalcogourato lead(II) complexes as precursors for AACVD†
Received
23rd August 2018
, Accepted 26th September 2018
First published on 16th November 2018
Abstract
Selenium and sulfur derivatives of lead(II) acylchalcogourato complexes have been used to deposit PbSxSe1−x thin films by AACVD. By variation of the mole ratio of sulfur and selenium precursors in the aerosol feed solution the full range of compositions of PbSxSe1−x was obtained, i.e. 0 ≥ x ≥ 1. The films showed no contaminant phases demonstrating the potential for acylchalcogourato metal complexes as precursors for metal chalcogenide thin films. The crystal structure for bis[N,N-diethyl-N′-2-naphthoylthioureato]lead(II) was solved and displayed the expected decreases in Pb–E bond lengths from the previously reported selenium variant.
Introduction
Lead chalcogenide nanomaterials have potential within photovoltaic devices due to their advantageous bulk bandgaps in the infra-red region (PbS = 0.41 eV, PbSe = 0.28 eV and PbTe = 0.31 eV) and the ability to tune the band gap by quantum confinement. The production of alloyed lead chalcogenide thin films allows for additional band gap tuning thus giving further control over the region of the electromagnetic spectrum collected. In this work we present a route to PbSxSe1−x thin films, where 0 ≥ x ≥ 1, using acylchalcogourea lead(II) complexes as precursors via aerosol assisted chemical vapour deposition (AACVD).
AACVD is a well-established technique for the production of thin films of metal chalcogenides. Single source precursors have been used as precursors for thin films by AACVD, which is a well-established route to synthesize a wide range of metal chalcogenides, CdE, PbE, NiE, SnS, ZnE etc. and has been reviewed previously by O'Brien et al.1–3 In particular lead chalcogenide films have been produced using lead complexed to dithiocarbamates,4–6 selenoureas,7–10 dichalcogenoimidophosphinates,5,11–13 dichalcogenophosphates14,15 and xanthates16–21 There are fewer reports on the use of single source precursors forming lead selenides due to the synthesis of noxious carbon diselenide when synthesising dithiocarbamates and xanthates. N-Acyl chalcogoureas offer a convenient route to a carbon–chalcogen double bond due to the availability and ease of the use of potassium selenocyanate and potassium thiocyanate as precursors. The ability to use potassium selenocyanate as a precursor for N-acylselenoureas is an attractive alternative to common selenium sources, e.g. carbon diselenide, as the starting material is potassium selenocyanate, an air stable solid at standard temperature and pressure, and the synthesis of the acylselenourea ligand is performed under air without heating.
Metal N-acylchalcogourea complexes have been previously explored to produce binary metal sulfide nanocrystals containing lead or iron by O'Brien et al. When using bis[N,N-diethyl-N′-naphthoylselenoureato]lead(II) in the presence of gold nanoparticles the precursor yielded PbSe nanorods with large aspect ratios, i.e. diameters of 8 to 25 nm, and lengths of 100 nm to a micron.8 By the use of a lower molecular weight complex, bis(N,N-diisobutyl-N′-(4-nitrobenzoyl)selenoureato)lead(II) Akhtar et al. produced PbSe thin films by AACVD and nanocrystals by a solvothermal synthesis.22 In combination with lead(II) ethylxanthate, bis[N,N-diethyl-N′-2-naphthoylselenocogoureato]lead(II) was used to produce thin films of PbSxSe1−xvia solution processing and thermolysis.23 The tris(N,N-diethyl-N′-naphthoylselenoureato)iron(III) analogue was used in AACVD to form FeSe films on silicon substrates and when using solvothermal methods in the presence of oleylamine produced FeS2.10
We report the use of the sulfur and selenium derivatives of bis[N,N-diethyl-N′-2-naphthoylchalcogoureato]lead(II) to synthesise PbSxSe1−x films with controlled compositions of sulfur and selenium. The composition of the films is controlled by variation of the mole fraction of the precursors used in the aerosol feed solution in the AACVD process and shows the expected slight deviation from Vegard's law as expected for PbSxSe1−x.
Experimental
Chemicals used
2-Naphthoyl chloride (98%, Sigma-Aldrich), acetone (≥99.9%, Sigma-Aldrich), potassium selenocyanate (97%, Sigma-Aldrich), diethylamine (≥99.5%, Sigma-Aldrich), ethanol (≥99.8%, Sigma-Aldrich), diethylether (≥99.8%, Sigma-Aldrich), potassium thiocyanate (≥99%, Sigma-Aldrich) and tetrahydrofuran (≥99.9%, Sigma-Aldrich) were used. All syntheses were performed under an inert nitrogen atmosphere and the work ups performed in air.
Synthesis of N,N-diethyl-N′-2-naphthoylselenourea (1)
The synthesis of N,N-diethyl-N′-2-naphthoylselenourea is adapted from the procedure by Douglass et al.24,25 In brief, a solution of 2-naphthoyl chloride (4.61 g, 24.2 mmol) in acetone (30 ml) was added dropwise to a stirred solution of potassium selenocyanate (3.48 g, 24.2 mmol) and was stirred for a further 15 minutes resulting in a green/yellow solution. A solution of diethylamine (1.77 g, 24.2 mmol) in acetone (10 ml) was added dropwise and stirred for 15 minutes resulting in an orange solution. N,N-Diethyl-N′-2-naphthoylselenourea was extracted from acetone by addition of diethylether (50 ml). The diethylether layer was collected and removed in vacuo resulting in a red solid which was recrystallised from warm ethanol to give orange crystals. Yield = 33% elemental analysis: found: C, 55.65; H, 5.60; N, 8.50; calc.: C, 57.66; H, 5.41; N, 8.41. 1H NMR (400 MHz, CDCl3) δ 8.69 (s, 1H, Ar), 8.37 (s, 1H, NH), 7.98–7.84 (m, 4H, Ar), 7.66–7.54 (m, 2H, Ar), 4.18 (q, J = 7.1 Hz, 2H, CH2), 3.66 (q, J = 7.2 Hz, 2H, CH2), 1.44 (t, J = 7.1 Hz, 3H, CH3), 1.35 (t, J = 7.2 Hz, 3H, CH3). 77Se NMR (400 MHz, CDCl3 with PhSeSePh) δ 479.23 (s, C
Se). νmax/cm−1 1649.1(s) (C
O). M.p. = 134–136 °C.
Synthesis of N,N-diethyl-N′-2-naphthoylthiourea (2)
The synthesis of 2 is identical to that of 1 except that potassium thiocyanate (1.96 g, 24.2 mmol) was used in place of potassium selenocyanate and yellow crystals were obtained. Yield = 34% elemental analysis: found: C, 67.26; H, 6.78; N, 9.82; S, 11.08; calc.: C, 67.10; H, 6.30; N, 9.80; S, 11.20. 1H NMR (400 MHz, CDCl3) δ 8.71 (s, 1H, Ar), 8.34 (s, 1H, NH), 8.03–7.74 (m, 4H, Ar), 7.56 (m, 2H, Ar), 4.03 (d, J = 6.8 Hz, 2H, CH2), 3.63 (d, J = 6.9 Hz, 2H, CH2), 1.33 (m, 6H, CH3). m/z 285.0 (M − H 100%) νmax/cm−1 1677.4(s) (C
O). M.p. = 105–106 °C.
Synthesis of bis[N,N-diethyl-N′-2-naphthoylselenoureato]lead(II) (3)
The synthesis of bis[N,N-diethyl-N′-2-naphthoylselenoureato]lead(II) is adapted from the synthesis by Akhtar et al.8 In brief, 2 (2.00 g, 6.0 mmol) was dissolved in 50 ml of warm ethanol followed by the addition of sodium ethoxide (0.41 g, 6.0 mmol). The solution was stirred for 20 minutes and a solution of lead acetate (1.14 g, 3.0 mmol) in ethanol (50 ml) was added dropwise. This was stirred for a further 20 minutes and was allowed to cool to room temperature forming a yellow precipitate. The precipitate was recrystallized from hexane/toluene to give yellow crystals.
Yield = 78% elemental analysis: found: C, 44.30; H, 3.81; N, 6.19; Pb, 23.80; calc.: C, 44.10; H, 3.90; N, 6.19; Pb, 23.68. 1H NMR (400 MHz, CDCl3) δ 8.60 (s, 2H, Ar), 8.12 (dd, J = 8.6, 1.6 Hz, 2H, Ar), 7.81 (dd, J = 31.0, 7.8 Hz, 4H, Ar), 7.73 (d, J = 8.6 Hz, 2H, Ar), 7.49–7.34 (m, 4H, Ar), 3.85–3.63 (m, 8H, CH2), 1.17 (t, J = 7.1 Hz, 6H, CH3), 1.00 (t, J = 7.1 Hz, 6H, CH3). 77Se NMR (400 MHz, CDCl3 with PhSeSePh) δ 292.23 (s, C–Se). m/z 873.2 (M+, 100%), 541.1 (78), 111.3 (30), 1409.4 (50). M.p. = 149 °C.
Synthesis of bis[N,N-diethyl-N′-2-naphthoylthioureato]lead(II) (4)
The synthesis of 4 is the same as that for 3 except that 2 (1.72 g, 6.0 mmol) was used in place of 1 with colourless crystals being collected following recrystallisation. Yield = 65.3% elemental analysis: found: C, 49.6; H, 4.2; N,7.2; S, 8.1; Pb, 25.2; calc.: C, 49.4; H, 4.4; N, 7.2; S, 8.2; Pb, 26.7. 1H NMR (400 MHz, CDCl3) δ 8.68 (s, 2H), 8.21 (dd, J = 8.6, 1.6 Hz, 2H), 7.92–7.77 (m, 6H), 7.49 (m, 4H, Ar), 3.84 (q, J = 7.1 Hz, 4H, CH2), 3.75 (q, J = 7.1 Hz, 4H, CH2), 1.23 (t, J = 7.1 Hz, 6H, CH3), 1.05 (t, J = 7.1 Hz, 6H, CH3). m/z 779.3 (M+, 100%), 493.1 (38), 287.1 (30), 1270.5 (24). M.p. = 198–199 °C.
AACVD procedure
In a typical deposition 200 mg of precursor was dissolved in tetrahydrofuran (20 ml). This was placed in a 100 ml round bottom flask with an inlet for the carrier gas (argon) and an outlet connected to an open ended quartz furnace tube. The tube was loaded with eight glass substrates (approx. 1 cm × 2 cm). The flask and tubing was purged with argon at a flow rate of 200 SCCM for 5 minutes prior to heating to 350 °C using a Carbolite tube furnace. An aerosol was generated by placing the round bottom flask in a water bath above a piezo-electric modulator of a PIFCO ultrasonic humidifier (model 1077) and carried to the furnace tube by the argon flow. Depositions were carried out for 30 minutes at the target temperature before being allowed to cool to room temperature.
Crystal structure determination
Crystals for single crystal X-ray diffraction studies were grown using vapour diffusion of diethylether into a solution of precursor in chloroform. Measurements were made using graphite monochromated Cu-Kα radiation on a Bruker Prospector diffractometer. The structures were solved by direct methods and refined by full-matrix least squares on F2. All calculations were carried out using the SHELXTL package Version 6.10.26 Non-hydrogen atoms were refined with anisotropic atomic displacement parameters. Hydrogen atoms were placed in calculated positions, assigned isotropic thermal parameters and allowed to ride on their parent carbon atoms.
Instrumentation
Thermogravimetric analysis (TGA) and elemental analysis were performed at the University of Manchester Microanalytical Laboratory, using a Thermo Scientific Flash 2000 organic elemental analyser and a Seiko SSC/S200 under N2. TGA profiles were acquired from room temperature to 600 °C with a heating rate of 10 °C min−1. Electron microscopy was performed using an FEI XL-30 scanning electron microscope (SEM) in a secondary electron configuration. 1H NMR spectra were collected using a Bruker AVANCE III 400 MHz spectrometer. X-ray diffraction (XRD) patterns were acquired with a Bruker D8 Advance diffractometer equipped with a Cu Kα source.
Results and discussion
Precursor characterisation
The structure of bis[N,N-diethyl-N′-2-naphthoylthioureato]lead(II) is shown in Fig. 1 and Table 1. The structure exhibits a stereochemically active inert pair resulting in a distorted square pyramidal structure. The structure is similar to that of the previously reported bis[N,N-diethyl-N′-2-naphthoylselenoureato]lead(II) with the exception of the Pb–S bonds being on average 0.8 Å shorter than their Pb–Se equivalents.8
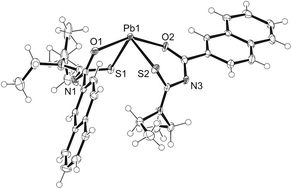 |
| Fig. 1 A thermal ellipsoid plot representing the structure of 4. CCDC ref. 1005699.† | |
Table 1 Selected bond lengths and angles for 4
Bond or angle |
Value |
Pb(1)–S(1) |
2.7080(13) Å |
Pb(1)–S(2) |
2.6996(12) Å |
O(1)–Pb(1) |
2.394(3) Å |
O(2)–Pb(1) |
2.450(3) Å |
O(2)–Pb(1)–S(1) |
76.09(9)° |
S(2)–Pb(1)–S(1) |
100.38(4)° |
The TGA profiles show considerable differences between the breakdown of the sulphur (a) and selenium (b) precursors, Fig. 2. From the first derivatives of the TGA profiles, ESI Fig. 1,† it can be seen that bis[N,N-diethyl-N′-2-naphthoylthioureato]lead(II), (a), has an onset at 146.3 °C and a three step decomposition whereas bis[N,N-diethyl-N′-2-naphthoylselenoureato]lead(II), (b), has a higher onset temperature at 210.6 °C and a two-step decomposition. This difference in the number of steps is surprising considering the similarities between the complexes.
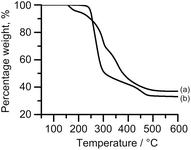 |
| Fig. 2 TGA of 4 & 3 shown as (a) & (b) respectively. | |
AACVD of mixtures of 3 & 4
The XRD patterns of all the compositions displayed cubic patterns as expected for PbE solids. Fig. 3(a) shows three patterns all showing a cubic pattern with shifts in all reflections across the patterns as the selenium content is increased. Inspection of the 422 reflection over increments of xSe = 0.05 is shown in Fig. 3(b). The gradual shift in the 422 reflection can be seen from that of pure PbS, xSe = 0, to pure PbSe, xSe = 0.5. The movement across the entire mole fraction range is shown in Fig. 3(c). A linear relationship between the mole fraction of the precursors in the CVD flask and the position of the 422 reflection can be observed between xSe = 0 and 0.4. From xSe = 0.5 to 1 the 422 reflection matches with the position in the PbSe reference pattern (ICDD 00-006-0354) indicating that the bis[N,N-diethyl-N′-2-naphthoylthioureato]lead(II) is no longer being incorporated into the films. Linear regression of the region between xSe = 0 and 0.4 results in a minimum xSe of 0.43 for pure PbSe.
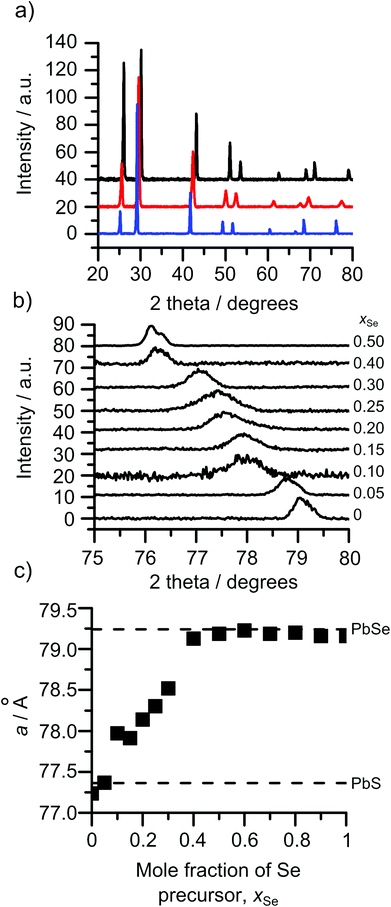 |
| Fig. 3 XRD analysis of the PbSxSe1−x films. (a) XRD patterns from xSe = 0 (black), xSe = 0.25 (red) and xSe = 0.5 (blue). (b) XRD patterns of the 422 reflection from xSe = 0 to 0.5. (c) Shows a plot of mole fraction against the position of the 422 reflection as compared to the values from the reference patterns (PbSe ICDD 00-006-0354 and PbS ICDD 00-005-0592) shown with dashed lines. | |
Comparison of the film composition by ICP-AES with the lattice constant, a, allows for examination in relation to Vegard's law, Fig. 4. The films exhibited a linear change in the lattice parameter with decreased sulphur content. This matches with the predicted behaviour of PbSxSe1−x which is expected to show a subtle deviation from linear behaviour.27
 |
| Fig. 4 (a) A plot showing the relationship between the mole fraction of the precursor used and the resulting composition of the film of PbSxSe1−x. (b) A Vegard plot for the PbSxSe1−x films between the mole fraction of the Se precursor, x(Se precursor), 0 to 0.6. Composition of the produced films was determined by ICP-AES analysis of Pb and Se. The dashed line represents ideal Vegardrian behaviour between PbS and PbSe using a from ICDD PbSe 00-006-0354 and ICDD PbS 00-005-0592. | |
Scanning electron micrographs show dramatic variations in the size and shape of the nanocrystals formed when altering the precursor mole fraction as shown in Fig. 5. Variation in the nanocrystal size could be a consequence of the difference in the initial breakdown temperature of the two complexes as shown in the TGA profiles, Fig. 2. The PbSe samples, i.e. where x(Se precursor) is 0.5 & 0.6 (e & f), show distinctly larger nanocrystals and less uniform films. EDX mapping was used to confirm that a uniform alloy was made, Fig. 6, with Pb, S and Se being found evenly spread across the film. Photographs of the films showed the typical dark brown colour when moving through the range of compositions of the PbSxSe1−x films, ESI Fig. 2.†
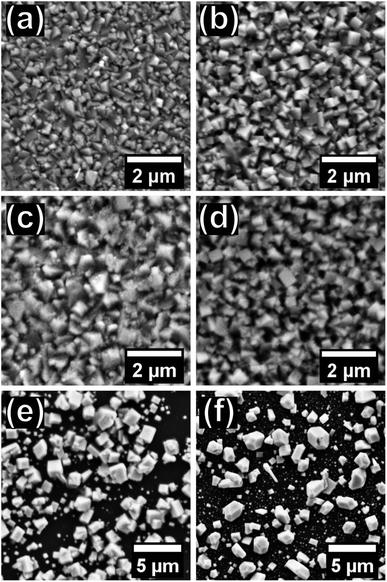 |
| Fig. 5 Scanning electron micrographs of PbSxSe1−x films produced from x(Se precursor) = 0, 0.1, 0.2, 0.3, 0.4, 0.5 and 0.6 represented by (a) to (f) respectively. | |
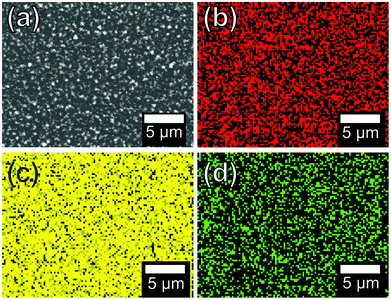 |
| Fig. 6 An SEM image for the alloyed films produced using x(Se precursor) = 0.3 (a) and the corresponding EDX maps for Pb, S and Se (b), (c) and (d) respectively. | |
Conclusions
AACVD of bis[N,N-diethyl-N′-2-naphthoylthioureato]lead(II) and bis[N,N-diethyl-N′-2-naphthoylselenoureato]lead(II) mixtures has successfully been used to make PbSxSe1−x films of controlled chalcogen compositions. The combination of precursors successfully makes alloyed nanocrystals and not two populations of nanocrystals of binary lead chalcogenide compositions. The precursors used performed as ideal single source precursors for AACVD due to the absence of additional undesired phases from the other elements present in the precursor. The relationship between the mole fraction of bis[N,N-diethyl-N′-2-naphthoylselenoureato]lead(II), xSe, and the position of the 422 reflection is linear between xSe = 0 and 0.43.
Conflicts of interest
There are no conflicts to declare.
Acknowledgements
POB and PDM would like to acknowledge funding from the Engineering and Physical Sciences Research Council (EPSRC) grants # EP/K010298/1 and EP/K039547/1. TEE and POB would like to thank the Nigerian Government for the PhD studentship that supported TEE.
Notes and references
- N. O. Boadi, M. A. Malik, P. O'Brien and J. A. M. Awudza, Dalton Trans., 2012, 41, 10497–10506 RSC.
- N. L. Pickett and P. O'Brien, Chem. Rec., 2001, 1, 467–479 CrossRef CAS PubMed.
- M. A. Malik, M. Afzaal and P. O'Brien, Chem. Rev., 2010, 110, 4417–4446 CrossRef CAS PubMed.
- M. Afzaal, K. Ahmad and P. O'Brien, J. Mater. Chem., 2012, 22, 12731–12735 RSC.
- M. Afzaal, K. Ellwood, N. L. Pickett, P. O'Brien, J. Raftery and J. Waters, J. Mater. Chem., 2004, 14, 1310–1315 RSC.
- T. Trindade and P. O'Brien, Chem. Vap. Deposition, 1997, 3, 75–77 CrossRef CAS.
- M. J. Moloto, N. Revaprasadu, G. A. Kolawole, P. O'Brien and M. A. Malik, S. Afr. J. Sci., 2005, 101, 463–465 CAS.
- J. Akhtar, M. Akhtar, M. A. Malik, P. O'Brien and J. Raftery, J. Am. Chem. Soc., 2012, 134, 2485–2487 CrossRef CAS PubMed.
- J. Akhtar, M. A. Malik, S. K. Stubbs, P. O'Brien, M. Helliwell and D. J. Binks, Eur. J. Inorg. Chem., 2011, 2011, 2984–2990 CrossRef CAS.
- M. Akhtar, J. Akhtar, M. A. Malik, F. Tuna, M. Helliwell and P. O'Brien, J. Mater. Chem., 2012, 22, 14970–14975 RSC.
- N. O. Boadi, P. D. McNaughter, M. Helliwell, M. A. Malik, J. A. M. Awudza and P. O'Brien, Inorg. Chim. Acta, 2016, 453, 439–442 CrossRef CAS.
- J. S. Ritch, T. Chivers, K. Ahmad, M. Afzaal and P. O'Brien, Inorg. Chem., 2010, 49, 1198–1205 CrossRef CAS PubMed.
- A. C. Onicha, N. Petchsang, T. H. Kosel and M. Kuno, ACS Nano, 2012, 6, 2833–2843 CrossRef CAS PubMed.
- T. Duan, W. Lou, X. Wang and Q. Xue, Colloids Surf., A, 2007, 310, 86–93 CrossRef CAS.
- L. Bolundut, I. Haiduc, G. Kociok-Khön and K. C. Molloy, Rev. Roum. Chim., 2010, 55, 741–746 CAS.
- N. Pradhan, B. Katz and S. Efrima, J. Phys. Chem. B, 2003, 107, 13843–13854 CrossRef CAS.
- J. Akhtar, M. Afzaal, M. A. Vincent, N. A. Burton, I. H. Hillier and P. O'Brien, Chem. Commun., 2011, 47, 1991–1993 RSC.
- J. M. Clark, G. Kociok-Kohn, N. J. Harnett, M. S. Hill, R. Hill, K. C. Molloy, H. Saponia, D. Stanton and A. Sudlow, Dalton Trans., 2011, 40, 6893–6900 RSC.
- P. D. McNaughter, J. C. Bear, A. G. Mayes, I. P. Parkin and P. O'Brien, R. Soc. Open Sci., 2017, 4, 170383 CrossRef CAS PubMed.
- P. D. McNaughter, S. A. Saah, M. Akhtar, K. Abdulwahab, M. A. Malik, J. Raftery, J. A. M. Awudza and P. O'Brien, Dalton Trans., 2016, 45, 16345–16353 RSC.
- E. A. Lewis, P. D. McNaughter, Z. Yin, Y. Chen, J. R. Brent, S. A. Saah, J. Raftery, J. A. M. Awudza, M. A. Malik, P. O'Brien and S. J. Haigh, Chem. Mater., 2015, 27, 2127–2136 CrossRef CAS.
- J. Akhtar, M. A. Malik, S. K. Stubbs, P. O'Brien, M. Helliwell and D. J. Binks, Eur. J. Inorg. Chem., 2011, 2011, 2984–2990 CrossRef CAS.
- S. A. Saah, P. D. McNaughter, M. A. Malik, J. A. M. Awudza, N. Revaprasadu and P. O'Brien, J. Mater. Sci., 2018, 53, 4283–4293 CrossRef CAS.
- I. B. Douglass, J. Am. Chem. Soc., 1937, 59, 740–742 CrossRef CAS.
- I. B. Douglass and F. B. Dains, J. Am. Chem. Soc., 1934, 56, 1408–1409 CrossRef CAS.
- G. M. Sheldrick, Acta Crystallogr., Sect. A: Found. Crystallogr., 2008, 64, 112–122 CrossRef CAS PubMed.
- Naeemullah, G. Murtaza, R. Khenata, N. Hassan, S. Naeem, M. N. Khalid and S. Bin Omran, Comput. Mater. Sci., 2014, 83, 496–503 CrossRef CAS.
Footnote |
† Electronic supplementary information (ESI) available. CCDC 1005699. For ESI and crystallographic data in CIF or other electronic format see DOI: 10.1039/c8dt03443e |
|
This journal is © The Royal Society of Chemistry 2018 |