DOI:
10.1039/D1QO01368H
(Research Article)
Org. Chem. Front., 2021,
8, 6704-6709
Alkoxycarbonyl radicals from alkyloxalyl chlorides: photoinduced synthesis of isoquinolinediones under visible light irradiation†
Received
13th September 2021
, Accepted 20th October 2021
First published on 22nd October 2021
Abstract
Alkyloxalyl chlorides, generated from alcohols and oxalyl chlorides, are used as alkoxycarbonyl radicals in the reaction of N-acryloyl benzamides under photocatalysis at room temperature. In this report, we demonstrate that this approach can be compatible with a variety of alcohol-containing pharmaceutically active compounds under visible light irradiation. A variety of isoquinoline-1,3(2H,4H)-diones are prepared in moderate to good yields.
Carbonylation reactions are of crucial importance in the field of modern organic synthesis since they provide access to carbonyl-containing derivatives, such as ketones, amides and carboxylic esters, which play an important role in fields ranging from organic chemistry to medical and pharmaceutical sciences.1 In these fields, acyl/carbamoyl/alkoxycarbonyl radicals are useful reactive intermediates which can be combined with carbon fragments to obtain unique carbonyl-containing derivatives.2 So far, many methods for the generation and application of acyl/carbamoyl radicals have been reported.3–12 For example, acyl radicals can be obtained either by single-electron reduction of acyl chlorides,4 anhydrides,5 and hypervalent iodine reagents,6 or upon oxidation of α-ketoacids7 and acyl silanes.8 Carbamoyl radicals can also be generated from the corresponding 4-carbamoyl dihydropyridines,9N-hydroxyphthalimido oxamides,10 oxalate monoamides11 and carbamoyl chlorides12 in the presence of a photocatalyst under visible light irradiation.
However, compared with acyl/carbamoyl radicals, alkoxycarbonyl radicals have remained largely unexplored.13 Although alkoxycarbonyl radicals can attack unsaturated C–C bonds leading to carboxylic esters, the concurrent decarboxylation step to form stable tertiary carbon radicals often plays a dominant role in synthesis. Thus, much effort has been devoted to alkoxycarbonyl radical decarboxylation through photocatalysis in recent years.14
Traditionally, alkoxycarbonyl radicals can be generated most commonly from the corresponding xanthates and selenides.15 Additionally, these radicals can also be formed from carbazates by treatment with metal catalysts and stoichiometric quantities of oxidants.16 Recently, it was reported that alkoxycarbonyl radicals could be produced by photoredox-catalyzed fragmentation of alkyl oxalates and methyl N-phthalimidoyl oxalates (Schemes 1a and b).13,14 The existing approaches for providing alkoxycarbonyl radical precursors often require multistep synthetic processes. Thus, the development of a robust strategy for the rapid generation of alkoxycarbonyl radicals under mild conditions, especially using readily available and inexpensive substrates, is highly desirable.
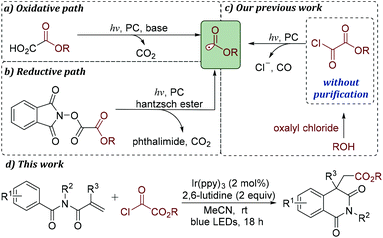 |
| Scheme 1 Generation of alkoxycarbonyl radicals under photoredox catalysis. | |
Xu and co-workers disclosed that single-electron reduction of aroyl chlorides with a photocatalyst would provide aroyl radicals.4 Inspired by this result, we expected that it might be possible to find a novel strategy for the generation of alkoxycarbonyl radicals from the corresponding acyl chloride under photocatalysis. Recently, we have reported that alkyloxalyl chlorides, generated in situ from the corresponding oxalyl chloride and alcohols, can be used as alkoxycarbonyl radical fragments under photoredox catalysis (Scheme 1c).17 To further demonstrate the advantage of this conversion, a variety of isoquinoline-1,3(2H,4H)-diones are prepared in moderate to good yields (Scheme 1d). An important feature of this alkoxycarbonylation/cyclization reaction is the capacity of alkyloxalyl chlorides to produce alkoxycarbonyl radicals through single-electron reduction/CO extrusion.
To verify the practicability of the above hypothesis, commercially available ethyl chlorooxoacetate (2a)18 as the alkoxycarbonyl radical precursor and N-methacryloyl-N-methylbenzamide (1a) as the radical trapper were selected as substrates for reaction development (Table 1). The reactions were performed at room temperature under visible light irradiation with a blue LED strip in the presence of Ir(ppy)3 as the photocatalyst. Since HCl would be released during the reaction process, 2,6-lutidine was added as the base. Gratifyingly, the desired alkoxycarbonylation/cyclization product (3a) was obtained in good yield (70%) (Table 1, entry 1). This result demonstrated that our design was feasible and promising. The yield of compound 3a was decreased to 37% when Ir(ppy)2(dtbbpy)PF6 was used as the photocatalyst (Table 1, entry 2). The donor−acceptor-type photocatalyst 3DPA2FBN, which is a strongly reducing organic photocatalyst,19 provided a lower yield (Table 1, entry 3). Next, the solvent effect was evaluated (Table 1, entries 4–7) and it was found that acetonitrile was the optimal reaction medium, giving rise to the corresponding product 3a in 76% yield (Table 1, entry 6). Subsequently, other bases were screened, and the result showed that inorganic bases were less efficient, while 2,6-di-tert-butylpyridine was comparably effective. Additionally, blank experiments demonstrated that both a photocatalyst and visible light were necessary for this alkoxycarbonylation/cyclization reaction (Table 1, entries 12 and 13). Meanwhile, the reaction could not occur when ethyl chloroformate (2a′) was used as the alkoxycarbonyl radical source (Table 1, entry 14). This result might be rationalized by the more negative reduction potential of alkyl chloroformates than the excited-state photocatalyst. With the optimized conditions in hand, we then investigated the scope of N-acryloyl benzamides in this reaction. As shown in Table 2, it was found that a wide range of N-methacryloyl benzamide derivatives bearing electron-donating (methyl, methoxy, tert-butyl and dimethyl) and electron-withdrawing (F, Cl and Br) substituents in the aromatic ring were suitable substrates for this transformation. Notably, reactions of para-tbutyl- and 3,5-dimethyl-substituted substrates afforded the acyl migration products 3e′ and 3f′, respectively. Substrates bearing different alkyl groups at the N-position of amides could readily convert into the isoquinoline-1,3(2H,4H)-diones (3i–3t). The excellent functional group tolerance of –Bn (3o), –CN (3p), and –CO2Et (3q), and a terminal unsaturated bond (3r) supported the practicality of this reaction. Additionally, chiral N-methacryloyl benzamides served as radical trappers in this alkoxycarbonylation/cyclization reaction, leading to the chiral isoquinoline-1,3(2H,4H)-diones (3s and 3t) in moderate yields. Acrylamides with a benzyl and phenyl group at the α-position converted into the target products 3u and 3v as well. To demonstrate the practicability of this alkoxycarbonylation/cyclization reaction, a gram-scale experiment was carried out, which provided the corresponding product 3a in 62% yield.
Table 1 Optimization of reaction parametersa,b

|
Entry |
PC |
Solvent |
Base |
Time |
3a (%) |
Unless otherwise noted, reaction conditions are as follows: 1a (0.2 mmol), 2a (0.6 mmol), photocatalyst (0.004 mmol), base (0.4 mmol), solvent (4 mL), and 36 W blue LEDs under a N2 atmosphere.
Yield determined by 1H NMR analysis using 1,3,5-trimethoxybenzene as an internal standard.
In the dark.
Compound 2a′ was used instead of ethyl chlorooxoacetate 2a.
|
1 |
Ir(ppy)3 |
DMF |
2,6-Lutidine |
12 h |
70 |
2 |
Ir(ppy)2(dtbbpy)PF6 |
DMF |
2,6-Lutidine |
12 h |
37 |
3 |
3DPA2FBN |
DMF |
2,6-Lutidine |
12 h |
47 |
4 |
Ir(ppy)3 |
MeCN |
2,6-Lutidine |
18 h |
76 |
5 |
Ir(ppy)3 |
THF |
2,6-Lutidine |
36 h |
14 |
6 |
Ir(ppy)3 |
DCM |
2,6-Lutidine |
48 h |
30 |
7 |
Ir(ppy)3 |
EA |
2,6-Lutidine |
48 h |
17 |
8 |
Ir(ppy)3 |
MeCN |
2,6-ditBu-Py |
18 h |
70 |
9 |
Ir(ppy)3 |
MeCN |
K2HPO4 |
18 h |
51 |
10 |
Ir(ppy)3 |
MeCN |
Na2HPO4 |
18 h |
19 |
11 |
Ir(ppy)3 |
MeCN |
KHCO3 |
18 h |
16 |
12 |
— |
MeCN |
2,6-Lutidine |
18 h |
0 |
13c |
Ir(ppy)3 |
MeCN |
2,6-Lutidine |
18 h |
0 |
14d |
Ir(ppy)3 |
MeCN |
2,6-Lutidine |
18 h |
0 |
Table 2 Photoinduced synthesis of isoquinolinediones under visible light irradiation from N-acryloyl benzamides and ethyl chlorooxoacetatesa
Unless otherwise noted, reaction conditions are as follows: 1 (0.2 mmol), 2a (0.6 mmol), Ir(ppy)3 (0.004 mmol), 2,6-lutidine (0.4 mmol), MeCN (4 mL), and 36 W blue LEDs under a N2 atmosphere.
|
|
Subsequently, various alkyloxalyl chlorides were examined, giving rise to the results shown in Table 3. It was found that alkyloxalyl chlorides generated directly from the corresponding alcohols could smoothly provide the alkoxycarbonyl radicals. The yield of the alkoxycarbonylation/cyclization product decreased to 85% (4avs.3p) when methyl oxalyl chloride was used as the radical precursor in the reaction with N-(2-cyanoethyl)-N-methacryloyl benzamide 1p. Further underscoring the compatibility of this transformation, some alkyloxalyl chlorides were obtained by treatment of alcohols with a slight excess of oxalyl chloride in DCM, which were employed in this radical cascade reaction after the removal of the solvent and the excess oxalyl chloride.20 The alkyloxalyl chloride derived from long-chain aliphatic alcohols was found to be successful in this transformation (4b). To further demonstrate the synthetic robustness of this conversion, we applied this approach for the construction of a series of diversely functionalized isoquinoline-1,3(2H,4H)-diones. Chlorooxoacetates derived from natural products including menthol (product 4c, 80% yield), (−)-borneol (product 4d, 58% yield), β-cholestanol (product 4e, 83% yield) and androsterone (product 4f, 81% yield) were workable as well. Chiral amino alcohol derivatives were satisfactory starting materials and could uniformly produce the synthetically valuable isoquinoline-1,3(2H,4H)-diones with good yields (4g and 4h).
Table 3 Photoinduced synthesis of isoquinolinediones under visible light irradiation from N-acryloyl benzamide 1p and alkyloxalyl chloridesa
Unless otherwise noted, reaction conditions are as follows: 1p (0.2 mmol), 2 (0.6 mmol), Ir(ppy)3 (0.004 mmol), 2,6-lutidine (0.4 mmol), MeCN (4 mL), and 36 W blue LEDs under a N2 atmosphere.
DMF was used instead of MeCN.
|
|
As mentioned above, the control experiments demonstrated that both visible light and photocatalyst were crucial for this alkoxycarbonylation/cyclization reaction. On the basis of these results and previous reports,17,21 we proposed a plausible mechanism as depicted in Scheme 2. Ethyl chlorooxoacetate (2a) could react with 2,6-lutidine to afford the corresponding acyl pyridinium salt (5).22 On the other hand, this photocatalytic cycle was initiated by the excitation of the photocatalyst Ir(ppy)3 (12) to the excited-state photocatalyst Ir(ppy)3*(13),22 which subsequently transferred a single electron to acyl pyridinium salt (5) to generate radical 6. Homolysis of the C–N bond would liberate 2,6-lutidine and produce acyl radical 7.22 The highly activated acyl radical 7 could rapidly go through CO extrusion and provide a stabilized alkoxycarbonyl radical 8. Radical 8 would then react with N-methacryloyl-N-methylbenzamide (1a), giving rise to a tertiary carbon radical intermediate 9. Subsequently, a radical-arene cyclization would occur leading to radical intermediate 10, which was readily oxidized by Ir(ppy)3+ to generate a cation intermediate 11 (path A). Alternatively, the species 10 could also be oxidized by acyl pyridinium salt (5) to providecation 11, along with the generated radical 6. After deprotonation, the desired product 3a would be produced.
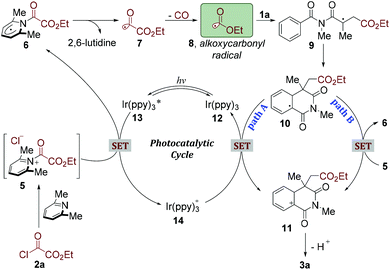 |
| Scheme 2 Proposed mechanism. | |
In conclusion, we have developed an alkoxycarbonylation/cyclization reaction of N-acryloyl benzamides and alkyloxalyl chlorides under photocatalysis at room temperature. The application of alkoxycarbonyl radicals in the intermolecular conjugate addition to electron-deficient alkenes has also been demonstrated. The key finding is the use of alkyloxalyl chlorides as alkoxycarbonyl radical precursors, which are generated from the corresponding alcohols and oxalyl chloride. With this approach, a variety of isoquinoline-1,3(2H,4H)-diones are prepared in moderate to good yields. Additionally, this strategy is compatible with a variety of alcohol-containing pharmaceutically active compounds.
Conflicts of interest
There are no conflicts to declare.
Acknowledgements
Financial support from the National Natural Science Foundation of China (No. 21871053), the Leading Innovative and Entrepreneur Team Introduction Program of Zhejiang (No. 2019R01005), and the Open Research Fund of the School of Chemistry and Chemical Engineering, Henan Normal University (2020ZD04) is gratefully acknowledged.
Notes and references
- For selected reviews, see:
(a) M. C. Willis, Transition Metal Catalyzed Alkene and Alkyne Hydroacylation, Chem. Rev., 2010, 110, 725 CrossRef CAS PubMed;
(b) J. Ruan and J. Xiao, From α-Arylation of Olefins to Acylation with Aldehydes: A Journey in Regiocontrol of the Heck Reaction, Acc. Chem. Res., 2011, 44, 614 CrossRef CAS PubMed;
(c) J. Liu, Z. Wei, H. Jiao, R. Jackstell and M. Beller, Toward Green Acylation of (Hetero)arenes: Palladium-Catalyzed Carbonylation of Olefins to Ketones, ACS Cent. Sci., 2018, 4, 30 CrossRef CAS PubMed;
(d) I. Ryu and N. Sonoda, Free-Radical Carbonylations: Then and Now, Angew. Chem., Int. Ed. Engl., 1996, 35, 1050 CrossRef;
(e) I. Ryu, Radical Carboxylations of Iodoalkanes and Saturated Alcohols Using Carbon Monoxide, Chem. Soc. Rev., 2001, 30, 16 RSC;
(f) A. Brennführer, H. Neumann and M. Beller, Palladium-Catalyzed Carbonylation Reactions of Aryl Halides and Related Compounds, Angew. Chem., Int. Ed., 2009, 48, 4114 CrossRef PubMed;
(g) Z. Chen, L.-C. Wang and X.-F. Wu, Carbonylative synthesis of heterocycles involving diverse CO surrogates, Chem. Commun., 2020, 56, 6016 RSC;
(h) L.-J. Cheng and N. P. Mankad, Copper-Catalyzed Carbonylative Coupling of Alkyl Halides, Acc. Chem. Res., 2021, 54, 2261 CrossRef CAS PubMed.
- For selected reviews, see:
(a) C. Raviola, S. Protti, D. Ravelli and M. Fagnoni, Photogenerated Acyl/Alkoxycarbonyl/Carbamoyl Radicals for Sustainable Synthesis, Green Chem., 2019, 21, 748 RSC;
(b) C. Chatgilialoglu, D. Crich, M. Komatsu and I. Ryu, Chemistry of Acyl Radicals, Chem. Rev., 1999, 99, 1991 CrossRef CAS PubMed;
(c) N. Chalotra, S. Sultan and B. A. Shah, Recent Advances in Photoredox Methods for Ketone Synthesis, Asian J. Org. Chem., 2020, 9, 863 CrossRef CAS.
-
(a) M. Zhang, J. Xie and C. Zhu, A General Deoxygenation Approach for Synthesis of Ketones from Aromatic Carboxylic Acids and Alkenes, Nat. Commun., 2018, 9, 3517 CrossRef PubMed;
(b) M. Zhang, X.-A. Yuan, C. Zhu and J. Xie, Deoxygenative Deuteration of Carboxylic Acids with D2O, Angew. Chem., Int. Ed., 2019, 58, 312 CrossRef CAS PubMed;
(c) R. Ruzi, J. Ma, X.-A. Yuan, W. Wang, S. Wang, M. Zhang, J. Dai, J. Xie and C. Zhu, Deoxygenative Arylation of Carboxylic Acids by Aryl Migration, Chem. – Eur. J., 2019, 25, 12724 CrossRef CAS PubMed;
(d) R. Ruzi, K. Liu, C. Zhu and J. Xie, Upgrading Ketone Synthesis Direct from Carboxylic Acids and Organohalides, Nat. Commun., 2020, 11, 3312 CrossRef CAS PubMed;
(e) X. Zhang and D. W. C. MacMillan, Direct Aldehyde C–H Arylation and Alkylation via the Combination of Nickel, Hydrogen Atom Transfer, and Photoredox Catalysis, J. Am. Chem. Soc., 2017, 139, 11353 CrossRef CAS PubMed;
(f) R. Sakamoto, N. Hirama and K. Maruoka, The Radical Acylarylation of N-Arylacrylamides with Aliphatic Aldehydes using the Photolysis of Hypervalent Iodine(III) Reagents, Org. Biomol. Chem., 2018, 16, 5412 RSC;
(g) B. Schweitzer-Chaput, M. A. Horwitz, E. de Pedro Beato and P. Melchiorre, Photochemical Generation of Radicals from Alkyl Electrophiles Using a Nucleophilic Organic Catalyst, Nat. Chem., 2019, 11, 129 CrossRef CAS PubMed.
-
(a) S.-M. Xu, J.-Q. Chen, D. Liu, Y. Bao, Y.-M. Liang and P.-F. Xu, Aroyl Chlorides as Novel Acyl Radical Precursors via Visible-Light Photoredox Catalysis, Org. Chem. Front., 2017, 4, 1331 RSC;
(b) C.-G. Li, G.-Q. Xu and P.-F. Xu, Synthesis of Fused Pyran Derivatives via Visible-Light-Induced Cascade Cyclization of 1,7-Enynes with Acyl Chlorides, Org. Lett., 2017, 19, 512 CrossRef CAS PubMed;
(c) Y. Liu, Q.-L. Wang, C.-S. Zhou, B.-Q. Xiong, P.-L. Zhang, C.-A. Yang and K.-W. Tang, Visible-Light-Mediated Ipso-Carboacylation of Alkynes: Synthesis of 3-Acylspiro[4,5]trienones from N-(p-Methoxyaryl)propiolamides and Acyl Chlorides, J. Org. Chem., 2018, 83, 2210 CrossRef CAS PubMed;
(d) Q.-S. Zhao, G.-Q. Xu, H. Liang, Z.-Y. Wang and P.-F. Xu, Aroylchlorination of 1,6-Dienes via a Photoredox Catalytic Atom-Transfer Radical Cyclization Process, Org. Lett., 2019, 21, 8615 CrossRef PubMed;
(e) Y.-L. Wei, J.-Q. Chen, B. Sun and P.-F. Xu, Synthesis of Indolo[2,1-a]isoquinoline Derivatives via Visible-Light-Induced Radical Cascade Cyclization Reactions, Chem. Commun., 2019, 55, 5922 RSC;
(f) D. V. Patil, H. Y. Kim and K. Oh, Visible Light-Promoted Friedel–Crafts-Type Chloroacylation of Alkenes to β-Chloroketones, Org. Lett., 2020, 22, 3018 CrossRef PubMed;
(g) Z. Lei, A. Banerjee, E. Kusevska, E. Rizzo, P. Liu and M.-Y. Ngai, β-Selective Aroylation of Activated Alkenes by Photoredox Catalysis, Angew. Chem., Int. Ed., 2019, 58, 7318 CrossRef PubMed.
-
(a) F. Pettersson, G. Bergonzini, C. Cassani and C.-J. Wallentin, Redox-Neutral Dual Functionalization of Electron-Deficient Alkenes, Chem. – Eur. J., 2017, 23, 7444 CrossRef PubMed;
(b) S. Dong, G. Wu, X. Yuan, C. Zou and J. Ye, Visible-light Photoredox Catalyzed Hydroacylation of Electron-Deficient Alkenes: Carboxylic Anhydride as an Acyl Radical Source, Org. Chem. Front., 2017, 4, 2230 RSC;
(c) M. Zhang, R. Ruzi, J. Xi, N. Li, Z. Wu, W. Li, S. Yu and C. Zhu, Photoredox-Catalyzed Hydroacylation of Olefins Employing Carboxylic Acids and Hydrosilanes, Org. Lett., 2017, 19, 3430 CrossRef PubMed;
(d) G. Bergonzini, C. Cassani and C. Wallentin, Acyl Radicals from Aromatic Carboxylic Acids by Means of Visible-Light Photoredox Catalysis, Angew. Chem., Int. Ed., 2015, 54, 14066 CrossRef PubMed;
(e) G. Bergonzini, C. Cassani, H. L. Olsson, J. Hçrberg and C. J. Wallentin, Visible-Light-Mediated Photocatalytic Difunctionalization of Olefins by Radical Acylarylation and Tandem Acylation/Semipinacol Rearrangement, Chem. – Eur. J., 2016, 22, 3292 CrossRef PubMed.
-
(a) W. Ji, H. Tan, M. Wang, P. Li and L. Wang, Photocatalyst-Free Hypervalent Iodine Reagent Catalyzed Decarboxylative Acylarylation of Acrylamides with α-Oxocarboxylic Acids Driven by Visible-Light Irradiation, Chem. Commun., 2016, 52, 1462 RSC;
(b) H. Huang, G. Zhang and Y. Chen, Dual Hypervalent Iodine(III) Reagents and Photoredox Catalysis Enable Decarboxylative Ynonylation under Mild Conditions, Angew. Chem., Int. Ed., 2015, 54, 7872 CrossRef PubMed;
(c) H. Tan, H. Li, W. Ji and L. Wang, Sunlight-Driven Decarboxylative Alkynylation of α-Keto Acids with Bromoacetylenes by Hypervalent Iodine Reagent Catalysis: A Facile Approach to Ynones, Angew. Chem., Int. Ed., 2015, 54, 8374 CrossRef PubMed.
-
(a) T. Xiao, L. Li and L. Zhou, Synthesis of Functionalized gem-Difluoroalkenes via a Photocatalytic Decarboxylative/Defluorinative Reaction, J. Org. Chem., 2016, 81, 7908 CrossRef PubMed;
(b) J.-Q. Chen, R. Chang, Y.-L. Wei, J.-N. Mo, Z.-Y. Wang and P.-F. Xu, Direct Decarboxylative–Decarbonylative Alkylation of α-Oxo Acids with Electrophilic Olefins via Visible-Light Photoredox Catalysis, J. Org. Chem., 2018, 83, 253 CrossRef PubMed;
(c) L. Chu, J. M. Lipshultz and D. W. C. MacMillan, Merging Photoredox and Nickel Catalysis: The Direct Synthesis of Ketones by the Decarboxylative Arylation of α-Oxo Acids, Angew. Chem., Int. Ed., 2015, 54, 7929 CrossRef PubMed;
(d) G. Z. Wang, R. Shang, W. M. Cheng and Y. Fu, Decarboxylative 1,4-Addition of α-Oxocarboxylic Acids with Michael Acceptors Enabled by Photoredox Catalysis, Org. Lett., 2015, 17, 4830 CrossRef PubMed;
(e) W. Cheng, R. Shang, H. Yu and Y. Fu, Room-Temperature Decarboxylative
Couplings of α-Oxocarboxylates with Aryl Halides by Merging Photoredox with Palladium Catalysis, Chem. – Eur. J., 2015, 21, 13191 CrossRef PubMed;
(f) T. Morack, C. Mück-Lichtenfeld and R. Gilmour, Bioinspired Radical Stetter Reaction: Radical Umpolung Enabled by Ion-Pair Photocatalysis, Angew. Chem., Int. Ed., 2019, 58, 1208 CrossRef PubMed;
(g) J.-J. Zhao, H.-H. Zhang, X. Shen and S. Yu, Enantioselective Radical Hydroacylation of Enals with α-Ketoacids Enabled by Photoredox/Amine Cocatalysis, Org. Lett., 2019, 21, 913 CrossRef PubMed.
- L. Capaldo, R. Riccardi, D. Ravelli and M. Fagnoni, Acyl Radicals from Acylsilanes: Photoredox-Catalyzed Synthesis of Unsymmetrical Ketones, ACS Catal., 2018, 8, 304 CrossRef.
- N. Alandini, L. Buzzetti, G. Favi, T. Schulte, L. Candish, K. D. Collins and P. Melchiorre, Amide Synthesis by Nickel/Photoredox-Catalyzed Direct Carbamoylation of (Hetero)Aryl Bromides, Angew. Chem., Int. Ed., 2020, 59, 5248 CrossRef PubMed.
- W. F. Petersen, R. J. K. Taylor and J. R. Donald, Photoredox-Catalyzed Reductive Carbamoyl Radical Generation: A Redox-Neutral Intermolecular Addition–Cyclization Approach to Functionalized 3,4-Dihydroquinolin-2-ones, Org. Lett., 2017, 19, 874 CrossRef PubMed.
- Q.-F. Bai, C. Jin, J.-Y. He and G. Feng, Carbamoyl Radicals via Photoredox Decarboxylation of Oxamic Acids in Aqueous Media: Access to 3,4-Dihydroquinolin-2(1H)-ones, Org. Lett., 2018, 20, 2172 CrossRef PubMed.
- E. de Pedro Beato, D. Mazzarella, M. Balletti and P. Melchiorre, Photochemical Generation of Acyl and Carbamoyl Radicals using a Nucleophilic Organic Catalyst: Applications and Mechanism Thereof, Chem. Sci., 2020, 11, 6312 RSC.
- Y. Slutskyy and L. E. Overman, Generation of the Methoxycarbonyl Radical by Visible-Light Photoredox Catalysis and Its Conjugate Addition with Electron-Deficient Olefins, Org. Lett., 2016, 18, 2564 CrossRef PubMed.
-
(a) G. L. Lackner, K. W. Quasdorf, G. Pratsch and L. E. Overman, Fragment Coupling and the Construction of Quaternary Carbons Using Tertiary Radicals Generated from tert-Alkyl N-Phthalimidoyl Oxalates by Visible-Light Photocatalysis., J. Org. Chem., 2015, 80, 6012 CrossRef PubMed;
(b) C. C. Nawrat, C. R. Jamison, Y. Slutskyy, D. W. C. MacMillan and L. E. Overman, Oxalates as Activating Groups for Alcohols in Visible Light Photoredox Catalysis: Formation of Quaternary Centers by Redox-Neutral Fragment Coupling, J. Am. Chem. Soc., 2015, 137, 11270 CrossRef PubMed;
(c) N. A. Weires, Y. Slutskyy and L. E. Overman, Angew. Chem., Int. Ed., 2019, 58, 8561 CrossRef PubMed.
-
(a) B. M. Trost, J. Waser and A. Meyer, Total Synthesis of (−)-Pseudolaric Acid B, J. Am. Chem. Soc., 2008, 130, 16424 CrossRef PubMed;
(b) W.-Y. Yu, W. N. Sit, K.-M. Lai, Z. Y. Zhou and A. S. C. Chan, Palladium-Catalyzed Oxidative Ethoxycarbonylation of Aromatic C−H Bond with Diethyl Azodicarboxylate, J. Am. Chem. Soc., 2008, 130, 3304 CrossRef PubMed;
(c) S. H. Kyne, C. H. Schiesser and H. Matsubarac, Multi-component Orbital Interactions during Oxyacyl Radical Addition Reactions Involving Imines and Electron-Rich Olefins, Org. Biomol. Chem., 2007, 5, 3938 RSC;
(d) M. D. Bachi and E. Bosch, Synthesis of α-Alkylidene-γ-Lactones by Intramolecular Addition of Alkoxycarbonyl Free-Radicals to Acetylenes, Tetrahedron Lett., 1986, 27, 641 CrossRef.
-
(a) T. Taniguchi, Y. Sugiura, H. Zaimoku and H. Ishibashi, Iron-Catalyzed Oxidative Addition of Alkoxycarbonyl Radicals to Alkenes with Carbazates and Air, Angew. Chem., Int. Ed., 2010, 49, 10154 CrossRef PubMed;
(b) X. Xu, Y. Tang, X. Li, G. Hong, M. Fang and X. Du, Iron-Catalyzed Arylalkoxycarbonylation of N-Aryl Acrylamides with Carbazates, J. Org. Chem., 2014, 79, 446 CrossRef PubMed;
(c) G. Wang, S. Wang, J. Wang, S.-Y. Chen and X.-Q. Yu, Synthesis of Oxindole-3-Acetates through Iron-Catalyzed Oxidative Arylalkoxycarbonylation of Activated Alkenes, Tetrahedron, 2014, 70, 3466 CrossRef;
(d) Z. Zong, S. Lu, W. Wang and Z. Li, Iron-Catalyzed Alkoxycarbonylation–Peroxidation of Alkenes with Carbazates and T-Hydro, Tetrahedron Lett., 2015, 56, 6719 CrossRef;
(e) Y. Tang, M. Zhang, X. Li, X. Xu and X. Du, Tetrabutylammonium Iodide-Catalyzed Radical Alkoxycarbonylation of Activated Alkenes: Synthesis of Isoquinolinedione Derivatives, Chin. J. Org. Chem., 2015, 35, 875 CrossRef;
(f) W. Kong, M. Casimiro, N. Fuentes, E. Merino and C. Nevado, Metal-Free Aryltrifluoromethylation of Activated Alkenes, Angew. Chem., Int. Ed., 2013, 52, 13086 CrossRef PubMed.
- J.-Q. Chen, X. Tu, Q. Tang, K. Li, L. Xu, S. Wang, M. Ji, Z. Li and J. Wu, Efficient Access to Aliphatic Esters by Photocatalyzed Alkoxycarbonylation of Alkenes with Alkyloxalyl Chlorides, Nat. Commun., 2021, 12, 5328 CrossRef PubMed.
- 0.5 kg/$227:supplier:Sigma-Aldrich and 0.5 kg/$202:supplier:TCI.
- E. Speckmeier, T. G. Fischer and K. Zeitler, A Toolbox Approach to Construct Broadly Applicable Metal-Free Catalysts for Photoredox Chemistry: Deliberate Tuning of Redox Potentials and Importance of Halogens in Donor–Acceptor Cyanoarenes, J. Am. Chem. Soc., 2018, 140, 15353 CrossRef PubMed.
- Y. Zhao, G. Wang, Y. Li, S. Wang and Z. Li, Design, Synthesis and Insecticidal Activities of Novel N–Oxalyl Derivatives of Neonicotinoid Compound, Chin. J. Chem., 2010, 28, 475 CrossRef.
- For selected examples, see:
(a) Y. Li, R. Mao and J. Wu,
N-Radical Initiated Aminosulfonylation of Unactivated C(sp3)–H Bond through Insertion of Sulfur Dioxide, Org. Lett., 2017, 19, 4472 CrossRef PubMed;
(b) T. Liu, Y. Li, L. Lai, J. Cheng, J. Sun and J. Wu, Photocatalytic Reaction of Potassium Alkyltrifluoroborates and Sulfur Dioxide with Alkenes, Org. Lett., 2018, 20, 3605 CrossRef PubMed;
(c) S. Ye, D. Zheng, J. Wu and G. Qiu, Photoredox-Catalyzed Sulfonylation of Alkyl Iodides, Sulfur Dioxide, and Electron-Deficient Alkenes, Chem. Commun., 2019, 55, 2214 RSC;
(d) J. Zhang, X. Li, W. Xie, J. Wu and S. Ye, Photoredox-Catalyzed Sulfonylation of O-Acyl Oximes via Iminyl Radicals with the Insertion of Sulfur Dioxide, Org. Lett., 2019, 21, 4950 CrossRef PubMed;
(e) X. Gong, M. Yang, J.-B. Liu, F.-S. He and J. Wu, Photoinduced Synthesis of alkylalkynyl Sulfones through A Reaction of Potassium Alkyltrifluoroborates, Sulfur Dioxide, and Alkynyl Bromides, Org. Chem. Front., 2020, 7, 938 RSC;
(f) X. Gong, M. Yang, J.-B. Liu, F.-S. He, X. Fan and J. Wu, A Metal-Free Route to Alkynyl Sulfones under Photoinduced Conditions with the Insertion of Sulfur Dioxide, Green Chem., 2020, 22, 1906 RSC.
-
(a) J. D. Nguyen, E. M. D'Amato, J. M. R. Narayanam and C. R. J. Stephenson, Engaging Unactivated Alkyl, Alkenyl and Aryl Iodides in Visible-Light-Mediated Free Radical Reactions, Nat. Chem., 2012, 4, 854 CrossRef PubMed;
(b) J.-Q. Chen, R. Chang, J.-B. Lin, Y.-C. Luo and P.-F. Xu, Photoredox-Induced Intramolecular 1,5-H Transfer Reaction of Aryl Iodides for the Synthesis of Spirocyclic γ-Lactams, Org. Lett., 2018, 20, 2395 CrossRef PubMed.
Footnotes |
† Electronic supplementary information (ESI) available. See DOI: 10.1039/d1qo01368h |
‡ These authors contributed equally. |
|
This journal is © the Partner Organisations 2021 |
Click here to see how this site uses Cookies. View our privacy policy here.