DOI:
10.1039/D0QM00623H
(Research Article)
Mater. Chem. Front., 2021,
5, 347-354
Multifunctional AIE-ESIPT dual mechanism tetraphenylethene-based Schiff base for inkless rewritable paper and a colorimetric/fluorescent dual-channel Zn2+ sensor†
Received
25th August 2020
, Accepted 22nd September 2020
First published on 30th September 2020
Abstract
Organic fluorescent materials have attracted extensive interests due to their wide applications. However, many organic fluorescent materials are single functional and suffer from complicated synthesis and purification, which significantly limits their practical applications. Herein, a multifunctional tetraphenylethene-based Schiff base (TPESB) was designed via a combination of AIE and ESIPT mechanisms and facilely developed through a one-pot reaction of two commercially available compounds, which exhibit dramatic absorption/fluorescence dual-mode color changes induced by water and zinc ions. By employing TPESB as an imaging layer and water as an ink, an eco-friendly inkless rewritable paper was developed showing great potential application in anti-counterfeiting and information security. Moreover, TPESB can serve as a colorimetric/fluorescent dual-channel sensor for Zn2+ ions with high sensitivity, high selectivity, fast response time, and low detection limit (38.9 nM). Additionally, TPESB displays low cytotoxicity and has been successfully applied for sensing of Zn2+ in living cells. The presented strategy will provide a reference for developing multifunctional materials and expanding their wider application fields.
Introduction
Organic fluorescent materials have aroused wide attention because of their extensive applications in fluorescent sensors, anti-counterfeiting, information storage and biological imaging.1–12 Traditional fluorescent dyes exhibit outstanding photophysical properties in dilute solutions, however, their fluorescence significantly decreased or was completely quenched in the aggregated or solid state due to the aggregation-caused quenching (ACQ) effect.13,14 The discovery of the aggregation-induced emission (AIE) phenomenon provided a new view for addressing the ACQ effect and developing highly fluorescent solid state materials.13 Functionalized AIEgens have been widely applied in physics-, chemistry- and biology-related fields showing great prospects in dealing with safety, health and energy problems after nearly 20 years of development.15–22
Generally, rational molecular design is an efficient approach to develop functional organic fluorescent materials. However, the complicated and time-consuming synthesis-purification process limits the practical application of organic fluorescent materials. Moreover, although the past decades have witnessed significant progress of organic fluorescent materials, most of them are single functional materials for only one kind of application and have very limited application scope.23–26 Therefore, developing multifunctional organic fluorescent materials with a simple synthetic process is highly desired, which will reduce production cost, save time and labour and show great prospects in practical application. Schiff base compounds have attracted particular interest due to their simple synthesis steps, convenient purification process, multi-stimulus-responsive properties and various applications.27–31 The facile synthetic procedure and multi-stimulus-responsive behaviour of Schiff base compounds enable them to be good candidates for developing organic fluorescent materials with simple synthesis and multiple functions.
In this study, we designed and developed an AIE and excited state intramolecular proton transfer (ESIPT) dual mechanism Schiff base compound (TPESB) through a one-pot reaction. The highly distorted structural AIE molecule, tetraphenylethene (TPE), was selected as the molecular skeleton for avoiding the ACQ effect in the solid state. The hydroxyl group on TPE was expected to form an intramolecular H-bond with the nitrogen atom for achieving the ESIPT process. Additionally, the adjacent nitrogen and oxygen atoms and hydroxyl groups were conjectured to respond to external stimuli, thus changing their photophysical properties. Careful investigation revealed that the absorption and fluorescence of TPESB can be significantly changed by water and zinc ions. Taking advantage of these stimulus-responsive properties, TPESB was successfully applied in an inkless rewritable paper and a colorimetric/fluorescent dual-channel sensor for Zn2+ ions with high sensitivity, high selectivity and low detection limit.
Results and discussion
Synthesis and characterization
The synthesis procedure for TPESB is illustrated in Scheme 1 and the detailed synthesis information can be found in the ESI.†TPESB was facilely developed via a one-pot reaction of commercially available compounds 2-hydroxy-5-(1,2,2-triphenylvinyl)benzaldehyde and o-phenylenediamine. The pure TPESB can be easily obtained by recrystallization avoiding complicated synthesis and time-consuming purification processes. The structure of TPESB was fully characterized by NMR spectroscopy and mass spectrometry.
 |
| Scheme 1 The synthesis procedure of TPESB. | |
Photophysical properties
The UV-vis absorption and photoluminescence (PL) spectra of TPESB in tetrahydrofuran (THF) are illustrated in Fig. S1 (ESI†). TPESB shows maximum absorption wavelength at 325 nm and two emission bands at about 410 and 590 nm, respectively. The 410 nm emission band is attributed to the enol form of TPESB, while the longer emission peak at 590 nm can be ascribed to its keto form resulting from the ESIPT reaction.32,33 The two emission bands of TPESB can be significantly changed in different solvents indicating their sensitivity to the environment (Fig. S2, ESI†). TPESB exhibits a large Stokes shift of 265 nm, which will avoid self-absorption and interference in fluorescence measurements.
Aggregation-induced emission (AIE)
The AIE properties of TPESB were investigated by adding various water fractions to its THF solution. All THF with different water content mixtures were freshly prepared immediately prior to the AIE measurement. As is shown in Fig. S3 (ESI†), TPESB exhibits very weak red emission in pure THF solution. However, when a large amount of water was added (90%), the weak emission was significantly increased (about 10 times). Therefore, TPESB is an AIE-active compound.
Water-induced absorption/fluorescence dual-mode color changes
An interesting phenomenon that the absorbance and fluorescence changed with time after low water fraction was added into its THF solution was observed in the investigation of AIE properties. As illustrated in Fig. 1, TPESB exhibited a maximum absorption peak at about 325 nm in THF and no other absorption bands were observed. However, when 10% water was added into its THF solution, a new absorption band at about 400–500 nm was detected and its absorbance gradually increased with time (Fig. 1A). The initially colourless solution gradually changed into obvious yellow after 10% water was added. Moreover, the emission intensity gradually increased and the emission colour changed from weak red emission to intense yellow emission after 10% water was added (Fig. 1E). Such absorption/fluorescence dual-mode colour changes were also observed in 20%, 30% and even 1% water fraction THF solutions (Fig. 1B, C, F, G and Fig. S4, ESI†). However, when the water fraction is further increased (50% to 90%), this phenomenon can not be seen anymore (Fig. 1D and H).
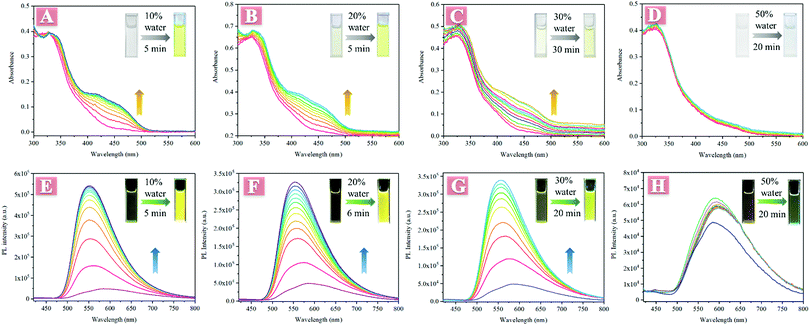 |
| Fig. 1 (A) The absorbance spectra change with time after addition of 10% water fraction. (B) The absorbance spectra change with time after addition of 20% water fraction. (C) The absorbance spectra change with time after addition of 30% water fraction. (D) The absorbance spectra change with time after addition of 50% water fraction. (E) The PL spectra change with time after addition of 10% water fraction. (F) The PL spectra change with time after addition of 20% water fraction. (G) The PL spectra change with time after addition of 30% water fraction. (H) The PL spectra change with time after addition of 50% water fraction. Insets: The images of changes of the samples with 10%, 20%, 30% and 50% water fractions before and after addition of water. Images in (A–D) were taken under ambient light; insets in (E–H) were taken under 365 nm UV light. For PL measurement, TPESB concentration: 10−5 M, and excitation wavelength: 365 nm. | |
The weak emission of TPESB in dilute solution and low water-fraction solutions can be attributed to the following reasons. Firstly, the photoinduced electron transfer (PET) effect can significantly quench the emission of TPESB.34–36 Secondly, the free rotation of the benzene rings will largely consume excited state energy through a non-radiative channel, which will quench the emission seriously.16 Based on these considerations, the interesting phenomenon may result from the following reasons. Firstly, water molecules can form intermolecular H-bonds with TPESB, which may restrict the PET effect, and thus enhance the emission intensity. The formation of intermolecular H-bonds can be verified by changes in NMR spectra before and after addition of water. As is shown, the 1H signals were significantly broadened after addition of 10% water, suggesting the intermolecular interaction between water and TPESB (Fig. S5, ESI†). Secondly, addition of water may lead to formation of aggregates, which will inhibit non-radioactive decay by restricted intramolecular rotation (RIR). The formation of aggregates can be supported by these factors: (1) the typical tails in the visible region caused by Mie scattering (Fig. 1A–C);37–40 (2) solid particles were observed by SEM after addition of water (Fig. S6, ESI†); (3) precipitation of larger aggregates can be seen after a certain period of aging time (Fig. S7, ESI†) and (4) the fluorescence lifetime became longer after addition of water (Fig. S8, ESI†). However, when a large amount of water is injected, TPESB molecules will immediately form tight-packing nanoparticles that are hardly affected by surrounding water molecules, which can be reasonable for the absence of absorbance/fluorescence changes in high water mixtures. The PL and absorption spectra of high water-fraction samples exhibit only normal fluctuation with time, and no obvious enhancement of intensity can be observed (Fig. S9 and S10, ESI†). The absorbance change may be also attributed to the intermolecular interaction/aggregation of TPESB molecules for the formation of nanoparticles.38,40–42 This speculation can be supported by experimental UV data of TPESB nanoparticles. As illustrated, in high water-fraction samples, an obvious shoulder band at about 420–520 nm can be observed compared to samples with 10% water fraction (Fig. S11, ESI†). This new shoulder band is similar to that observed in low water-fraction samples and should be attributed to the formation of nanoparticles.42 The tails in the visible region caused by Mie scattering and the SEM images indicate the formation of aggregates in high water-fraction samples (Fig. S12, ESI†). The morphology of the formed nanoparticles in high water-fraction samples is similar to that observed in Fig. S6B (ESI†). These results indicate that the absorbance/fluorescence changes result from the formation of aggregates.
Application of TPESB in inkless rewritable paper
Taking advantage of this interesting phenomenon, an eco-friendly inkless rewritable paper was developed by employing TPESB as the imaging layer and water as ink. This inkless rewritable paper was developed by a layer-by-layer process,43,44 and has great prospects in anti-counterfeiting, information security and data storage (Fig. 2A). Firstly, the filter paper served as the bottom layer and then a passivation layer, poly(ethylene glycol) (PEG), was directly coated on the filter paper. Finally, PEG integrated with TPESB was employed as the imaging layer. PEG performs multiple functions and helps in enhancing the practical usability of this rewritable paper. PEG can passivate the hydroxyl groups of paper, prevent the formation of microcrystalline aggregates and increase the opportunity of interaction between water and TPESB due to its good swellability for water.43
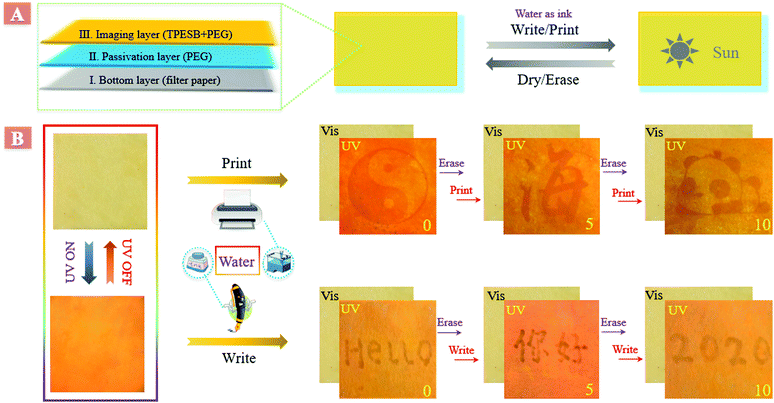 |
| Fig. 2 (A) Schematic illustrations of three-layer structure of the inkless rewritable paper and the print/write–erase process. (B) Photographs of patterns, letters and numbers printed/written on the inkless rewritable paper during 10 consecutive print/write–erase rounds by using pure water as ink. | |
As illustrated, employing water as the ink, different patterns, letters and figures can be printed and written on the produced paper by using a commercial water-jet printer and fountain pen (Fig. 2B). The printed/written information is almost invisible under ambient light and can be clearly seen under UV light. Moreover, the printed/written information can be self-erased in minutes and can be erased in seconds by drying. New information can be reprinted/written on the paper immediately after previous data were erased. All printed/written patterns exhibited good visibility without contrast loss during 10 consecutive print/write–erase rounds, which can be also supported by the little changed emission intensity (Fig. S13, ESI†). The rewritable, self-erased and water-stimulus-responsive properties of the fabricated paper have great potential applications in anti-counterfeiting.
Additionally, we found that the self-erasing time of the printed/written information can be adjusted from minutes to hours by varying the ratio of water and ethylene glycol (EG). Therefore, this rewritable paper can be applied in information security (Fig. 3). As is shown, by changing the ratio of water and EG, three kinds of inks were developed. The written number “8888” on the produced paper using these inks was almost invisible under visible light but can be clearly seen under 365 UV light. The originally encrypted “8888” changed into new encrypted “9608” because of the evaporation of ink 3 after 360 seconds passed. After 1.5 hours, the encrypted number “9608” transformed into the final information “3579” due to evaporation of ink 2. Then, the decrypted information “3579” will be self-erased in about 4 hours. The encrypted time can be dynamically changed by changing the ratio of EG and water, which increases the flexibility and practicability in practical application. Different information can be acquired at different times and only those who know the evolution process can read all the information, which improves the security of data significantly. In addition, this rewritable paper can also be applied in data storage due to this self-erasing time control strategy.
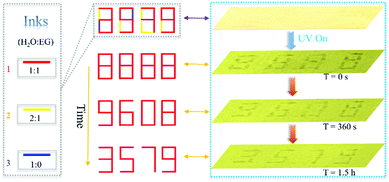 |
| Fig. 3 Application of the inkless rewritable paper in information safety. | |
Zn2+ ion induced absorption/fluorescence dual-mode colour changes
Further investigation found that Zn2+ ions can also induce absorption/fluorescence dual-mode colour changes of TPESB. As illustrated, TPESB exhibits a weak emission peak around 600 nm, which blue-shifted to 550 nm after addition of Zn2+ ions (Fig. 4A). The emission colour changes from the original weak red emission to intense yellow emission immediately after Zn2+ ions were added indicating fast response time. Moreover, a new absorption band around 490 nm emerged after Zn2+ ions were introduced and the solution colour changes from colourless to yellow (Fig. 4A). Such changes of absorbance and emission spectra of TPESB make it a potential colorimetric/fluorescent dual-channel sensor for Zn2+ ions.
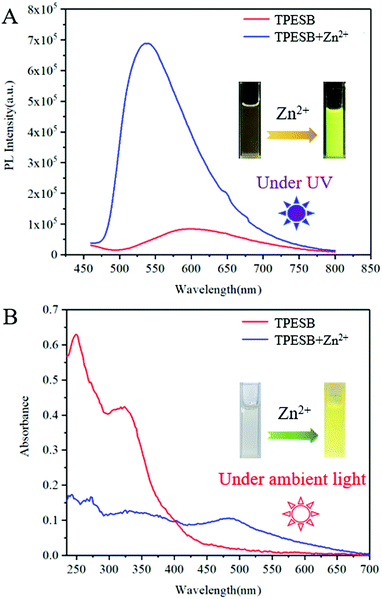 |
| Fig. 4 Changes of PL (A) and UV-vis absorption spectra (B) of TPESB in THF/water mixture (v/v = 1/1) before and after addition of Zn2+ ions. Insets: The images of TPESB in a THF/water mixture (v/v = 1/1) before and after addition of Zn2+ ions taken under 365 UV (A) and ambient light (B), respectively. For PL and absorption spectral measurements, TPESB concentration: 10−5 M, excitation wavelength: 365 nm. | |
Application of TPESB as a colorimetric/fluorescent dual-channel sensor for Zn2+ ions
In order to test the selectivity of TPESB to Zn2+ ions, various representative interferents such as Li+, Na+, K+, Mg2+, Mn2+, Co2+, Ni2+, Cd2+, Fe2+, Fe3+, Ag+, Pb2+, Ba2+, Cs+, NH4+, Bi3+ and Sn4+ are introduced to investigate their impact on the fluorescence and absorption spectra. As illustrated, the emission intensity and emission wavelengths of TPESB exhibit slight changes after addition of the above interfering ions and the fluorescence “turn-on” phenomenon was observed only when Zn2+ was added (Fig. 5A and B). In addition, the absorbance spectra also show little changes after addition of these interfering ions and only Zn2+ can result in the significant changes of the absorbance spectrum (Fig. 5E and F). These results indicate the high selectivity of TPESB for Zn2+ ions. The competitive experiment shows that the fluorescence and absorbance intensity resulting from the mixture of competitive ions and Zn2+ ions is comparable with that induced by Zn2+ only, which indicates a good anti-interference property of TPESB (Fig. S14, ESI†).
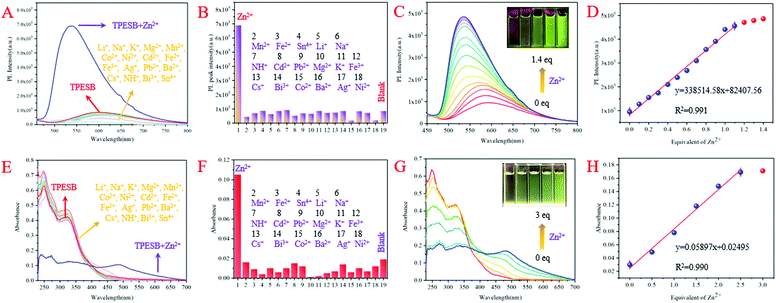 |
| Fig. 5 (A) PL spectra of TPESB in THF/water mixture (v/v = 1/1) after addition of different ions. (B) PL peak intensity of TPESB in THF/water mixture (v/v = 1/1) in the presence of different ions, the data are extracted from (A). (C) PL spectral changes of TPESB in THF/water mixture (v/v = 1/1) after addition of different [Zn2+]. Inset of (C): images of TPESB in THF/water mixture (v/v = 1/1) after addition of different [Zn2+], from left to right: 0 equiv., 0.2 equiv., 0.4 equiv., 0.8 equiv. and 1 equiv. (D) The fluorescence peak intensity as a function of [Zn2+]. (E) The absorption spectra of TPESB in THF/water mixture (v/v = 1/1) after addition of different ions. (F) Absorbance (490 nm) of TPESB in THF/water mixture (v/v = 1/1) in the presence of different ions, the data are extracted from (E). (G) The absorption spectral changes of TPESB in THF/water mixture (v/v = 1/1) after addition of different [Zn2+]. Inset of (G): images of TPESB in THF/water mixture (v/v = 1/1) after addition of different [Zn2+], from left to right: 0 equiv., 0.5 equiv., 1 equiv., 1.5 equiv. and 3 equiv. (H) The absorbance of TPESB as a function of [Zn2+]. For PL and absorbance measurements, TPESB concentration: 10−5 M, and excitation wavelength: 365 nm. | |
The PL and absorbance spectral changes of TPESB in THF/water mixture (v/v = 1/1) were further measured after various concentrations of Zn2+ ions were added. The fluorescence intensity increases dramatically with increasing Zn2+ ion concentration accompanied by the emission change from weak red to strong yellow emission (Fig. 5C). A new absorption band at about 450–500 nm emerges and gradually increases in intensity with increasing Zn2+ ion concentration (Fig. 5G). In addition, the colour of the solution changes from originally colourless to yellow with gradually increasing Zn2+ ion concentration. As can be seen from Fig. 5D and H, the emission and absorbance intensity of TPESB increases almost linearly with increasing Zn2+ ion concentrations from 0 to 1 equiv. and from 0–3 equiv., respectively, which has fitted linear relationships with [Zn2+]. Therefore, TPESB can be used as a dual-channel sensor for quantitative visual colorimetric and fluorescence discrimination for Zn2+ ions. The detection limit (DL) of probe TPESB for Zn2+ ions is calculated to be 38.9 nM, which is lower than those of many reported probes (Table S1, ESI†).45–55
The fluorescence and absorbance spectral changes caused by Zn2+ ions should result from the coordination between TPESB and Zn2+ ions. The coordination with Zn2+ ions can result in formation of a complex, which is responsible for the emergence of a new absorbance band. The chelation effect will restrict the PET effect and prevent the ESIPT and non-radioactive process, and thus induce enhanced fluorescence phenomenon and the emission wavelength change. Job's plot analysis indicates a 1
:
1 stoichiometric binding between TPESB and Zn2+ ions (Fig. S15, ESI†). The binding mode is also proved by the mass and NMR spectra. The observed peak at m/z 886.2551 is attributed to the complex [TPESB + Zn−2H]+ (Fig. S16, ESI†). The H proton located at about 13.05 and 8.65 ppm should be attributed to hydroxy protons and –CH
N– protons, respectively (Fig. S17, ESI†). After addition of 1.0 equiv. of Zn2+ ions, the proton signals at 13.05 disappeared indicating the deprotonation of –OH groups and their involvement in coordination. The proton peaks at 8.65 ppm were significantly broadened and downfield-shifted to 10.05 ppm, which indicates the participation of nitrogen atoms in coordination to Zn2+ ions. The FT-IR spectra of free TPESB and TPESB-Zn2+ were investigated. As illustrated in Fig. S18 (ESI†), an obvious shift of the stretch vibration absorption of the C
N bond from 1615 cm−1 (free TPESB) to 1548 cm−1 (TPESB-Zn2+) can be observed.56,57 The NMR and FT-IR data indicated that the nitrogen in the C
N band participated in the coordination with Zn2+. The theoretical calculation indicates a reasonable decrease of the HOMO–LUMO energy gap of the zinc complex of TPESB compared with that of TPESB, which agrees with the absorption spectral changes after addition of Zn2+ ions (Fig. 6).49–51 The proposed mechanism of the complex formation between TPESB and Zn2+ ions is shown in Fig. S19 (ESI†).
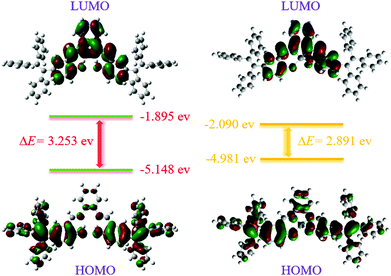 |
| Fig. 6 Energy level diagrams for the frontier molecular orbitals of TPESB (left) and its zinc complex (right) calculated by using the B3LYP/6-31G basis set. | |
The practical application performance of TPESB in detecting Zn2+ was further investigated. Known concentrations of Zn2+ ions were added in real water samples (tap water and Jinghu Lake water, in our university). Then the proposed colorimetric and fluorescence method was used to determine the concentration of Zn2+ ions and the results are illustrated in Table S2 (ESI†). These results revealed a good agreement between the spiked and the detected Zn2+ concentrations indicating the potential application of TPESB for monitoring Zn2+ in real water samples.
Fluorescence imaging of Zn2+ in living cells
In consideration of good sensing properties to Zn2+ ions, the ability of TPESB in detection of Zn2+ in living biologic cells was further investigated by fluorescence imaging experiments. The cytotoxicity of TPESB was first evaluated using the (4,5-dimethyl-2-thiazolyl)-2,5-diphenyltetrazolium bromide (MTT) assay, which indicates a low toxicity for living SiHa cells (Fig. S20, ESI†). As illustrated, almost no fluorescence was observed in the cells in the green channel after SiHa cells were incubated with TPESB (30 μM) for 40 min (Fig. 7). After Zn2+ ions (30 μM) were added and incubated for 40 min, an intense green-yellow emission in the green channel was observed due to the coordination between TPESB and Zn2+ (Fig. 7). These results revealed that TPESB can be used as a potential fluorescent probe for monitoring Zn2+ in living cells.
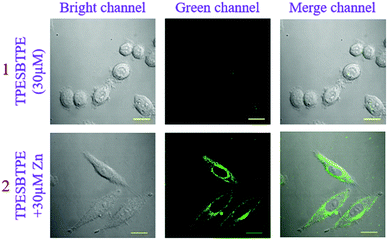 |
| Fig. 7 Fluorescence images of SiHa cells. (1) SiHa cells were incubated with TPESB (30 μM) for 40 min; (2) SiHa cells were pretreated with TPESB (30 μM) for 40 min, and then incubated with Zn2+ ions (30 μM) for another 40 min. | |
Conclusions
In conclusion, we facilely developed an AIE and ESIPT dual mechanism fluorescent material (TPESB) via a one-pot reaction, which exhibited multi-stimulus-responsive properties. The absorption and fluorescence spectra can be significantly changed by water and zinc ions. Taking advantage of the interesting changes of absorbance and fluorescence properties induced by water, a novel inkless rewritable paper was developed exhibiting great prospects in anti-counterfeiting and information security. Moreover, TPESB was successfully employed as a colorimetric/fluorescent dual-channel sensor for Zn2+ ions with high sensitivity, high selectivity, fast response time and low detection limit and was applied for sensing of Zn2+ in living cells. The design strategy presented in this study offers a simple and efficient way of developing multifunctional materials and helping in extending their practical application scope.
Conflicts of interest
There are no conflicts to declare.
Acknowledgements
This work was supported by the Fundamental Research Funds for the Central Universities (2019ZDPY21) and the Priority Academic Program Development of Jiangsu Higher Education Institutions.
References
- J. H. Wang, Y. M. Liu, J. B. Chao, H. Wang, Y. Wang and S. Shuang, A simple but efficient fluorescent sensor for ratiometric sensing of Cd2+ and bio-imaging studies, Sens. Actuators, B, 2020, 303, 127216 CrossRef CAS.
- Z. X. Li, H. X. Liu, H. X. Li, Y. H. Tsou, Y. L. Gao, X. Y. Xu, W. W. Du, L. H. Wei and M. M. Yu, Lysosome-targeting NIR ratiometric luminecent upcoversion nanoprobe toward arginine, Sens. Actuators, B, 2019, 280, 94–101 CrossRef CAS.
- J. M. Wang, Z. D. Teng, T. Cao, J. Qian, L. Zheng, Y. P. Cao, W. W. Qina and H. C. Guo, Turn-on visible and ratiometric near-infrared fluorescent probes for distinction endogenous esterases and chymotrypsins in live cells, Sens. Actuators, B, 2020, 306, 127567 CrossRef CAS.
- G. Huang, Q. Xia, W. Huang, J. Tian, Z. He, B. S. Li and B. Z. Tang, Multiple anti-counterfeiting guarantees from a simple tetraphenylethylene derivative-high-contrasted and multi-state mechanochromism and photochromism, Angew. Chem., Int. Ed., 2019, 58, 17814–17819 CrossRef CAS.
- C. X. Liu, J. Xu, F. Yang, W. Zhou, Z. X. Li, L. H. Wei and M. M. Yu, Nanomolar Cu2+ and F− naked-eye detection with a 1,8-naphthalimide-based colorimetric probe, Sens. Actuators, B, 2015, 212, 364–370 CrossRef CAS.
- A. Forni, E. Lucenti, C. Botta and E. Cariati, Metal free room temperature phosphorescence from molecular self-interactions in the solid state, J. Mater. Chem. C, 2018, 6, 4603–4626 RSC.
- M. Zhang, Y. Li, K. Gao, Z. Li, Y. Liu, Y. Liao, Y. Duan and T. Han, A turn-on mechanochromic luminescent material serving as pressure sensor and rewritable optical data storage, Dyes Pigm., 2020, 173, 107928 CrossRef CAS.
- Q. Zhang, G. Wang, H. Li, F. Zhu, H. Li and J. Lu, Improved molecular stacking and data-storage performance of pyridine- and pyrimidine-substituted small molecules, Adv. Funct. Mater., 2018, 28, 1800568 CrossRef.
- Y. Cao, X. Wang, X. Shi, S. M. Clee, P. L. McGeer, M. O. Wolf and C. Orvig, Biological imaging with medium-sensitive bichromatic flexible fluorescent dyes, Angew. Chem., Int. Ed., 2017, 56, 15603–15606 CrossRef CAS.
- A. C. Sedgwick, L. Wu, H. H. Han, S. D. Bull, X. P. He, T. D. James, J. L. Sessler, B. Z. Tang, H. Tian and J. Yoon, Excited-state intramolecular proton-transfer (ESIPT) based fluorescence sensors and imaging agents, Chem. Soc. Rev., 2018, 47, 8842–8880 RSC.
- Y. Wu, L. H. You, Z. Q. Yu, J. H. Wang, Z. Meng, Y. Liu, X. S. Li, K. Fu, X. K. Ren and B. Z. Tang, 1 Rational Design of Circularly Polarized Luminescent Aggregation-Induced Emission Luminogens (AIEgens): Promoting the Dissymmetry Factor and Emission Efficiency Synchronously, ACS Mater. Lett., 2020, 2, 505–510 CrossRef CAS.
- X. J. Wan, S. Yao, H. Y. Liu and Y. W. Yao, Selective fluorescence sensing of Hg2+ and Zn2+ ions through dual independent channels based on the site-specific functionalization of mesoporous silica nanoparticles, J. Mater. Chem. A, 2013, 1, 10505–10512 RSC.
- Q. Zhao and J. Z. Sun, Red and near infrared emission materials with AIE characteristics, J. Mater. Chem. C, 2016, 4, 10588–10609 RSC.
- M. Huang, R. Yu, K. Xu, S. Ye, S. Kuang, X. Zhu and Y. Wan, An arch-bridge-type fluorophore for bridging the gap between aggregation-caused quenching (ACQ) and aggregation-induced emission (AIE), Chem. Sci., 2016, 7, 4485–4491 RSC.
- J. Mei, N. L. Leung, R. T. Kwok, J. W. Lam and B. Z. Tang, Aggregation-induced emission: together we shine, united we soar, Chem. Rev., 2015, 115, 11718–11940 CrossRef CAS.
- H. B. Cheng, Y. Li, B. Z. Tang and J. Yoon, Assembly strategies of organic-based imaging agents for fluorescence and photoacoustic bioimaging applications, Chem. Soc. Rev., 2020, 49, 21–31 RSC.
- H. Sun, X. X. Tang, B. X. Miao, Y. Yang and Z. Ni, A new AIE and TICT-active tetraphenylethene-based thiazole compound: synthesis, structure, photophysical properties and application for water detection in organic solvents, Sens. Actuators, B, 2018, 267, 448–456 CrossRef CAS.
- Y. Chen, J. W. Y. Lam, R. T. K. Kwok, B. Liu and B. Z. Tang, Aggregation-induced emission: fundamental understanding and future developments, Mater. Horiz., 2019, 6, 428–433 RSC.
- Y. Cheng, J. Wang, Z. Qiu, X. Zheng, N. L. C. Leung, J. W. Y. Lam and B. Z. Tang, Multiscale humidity visualization by vnvironmentally sensitive fluorescent molecular rotors, Adv. Mater., 2017, 29, 1703900 CrossRef.
- H. Sun, X. X. Tang, R. Zhang, W. H. Sun, B. X. Miao, Y. Zhao and Z. H. Ni, Tetraphenylethene-substituted benzothiadiazoles: AIE and TICT properties, tunable intramolecular conjugation and application in detecting trace water in organic solvents, Dyes Pigm., 2020, 174, 108051 CrossRef CAS.
- H. Sun, S. S. Sun, F. F. Han, Z. H. Ni, R. Zhang and M. D. Li, A new tetraphenylethene-based Schiff base: two crystalline polymorphs exhibiting totally different photochromic and fluorescence properties, J. Mater. Chem. C, 2019, 7, 7053–7060 RSC.
- X. Zheng, W. Zhu, C. Zhang, Y. Zhang, C. Zhong, H. Li, G. Xie, X. Wang and C. Yang, Self-assembly of a highly emissive pure organic imine-based stack for electroluminescence and cell imaging, J. Am. Chem. Soc., 2019, 141, 4704–4710 CrossRef CAS.
- R. Isci, E. Tekin, K. Kaya, S. Piravadili Mucur, S. F. Gorkem and T. Ozturk, Tetraphenylethylene substituted thienothiophene and dithienothiophene derivatives: synthesis, optical properties and OLED applications, J. Mater. Chem. C, 2020, 8, 7908–7915 RSC.
- Z. Bao, C. Qin, J. J. Wang, J. Sun, L. Dai, G. Chen and F. Mei, A sensitive and selective probe for visual detection of Cu2+ based on 1, 8-naphthalimidederivative, Sens. Actuators, B, 2018, 265, 234–241 CrossRef CAS.
- C. Xu, Y. Zhou, Y. Cui, X. Liu and X. Peng, A facile AIEgen-based fluorescent probe design strategy and its application in hypochlorite probe construction, Sens. Actuators, B, 2020, 314, 128083 CrossRef CAS.
- X. Feng, Y. Li, X. He, H. Liu, Z. Zhao, R. T. K. Kwok, M. R. J. Elsegood, J. W. Y. Lam and B. Z. Tang, A substitution-dependent light-up fluorescence probe for selectively detecting Fe3+ ions and its cell imaging application, Adv. Funct. Mater., 2018, 28, 1802833 CrossRef.
- L. Wang, Y. Li, X. You, K. Xu, Q. Feng, J. Wang, Y. Liu, K. Li and H. Hou, An erasable photo-patterning material based on a specially designed 4-(1,2,2-triphenylvinyl)aniline salicylaldehyde hydrazone aggregation-induced emission (AIE) molecule, J. Mater. Chem. C, 2017, 5, 65–72 RSC.
- H. Sun, J. Y. Li, F. F. Han, R. Zhang, Y. Zhao, B. X. Miao and Z. H. Ni, Reversible photochromic tetraphenylethene-based Schiff base: design, synthesis, crystal structure and applications as visible light driven rewritable paper and UV sensor, Dyes Pigm., 2019, 167, 143–150 CrossRef CAS.
- G. Liu, M. Yang, L. Wang, J. Zheng, H. Zhou, J. Wu and Y. Tian, Schiff base derivatives containing heterocycles with aggregation-induced emission and recognition ability, J. Mater. Chem. C, 2014, 2, 2684–2691 RSC.
- J. Zhang, L. L. Xu and W. Y. Wong, Energy materials based on metal Schiff base complexes, Coord. Chem. Rev., 2018, 355, 180–198 CrossRef CAS.
- H. Y. Liu, T. Q. Liu, J. Li, Y. M. Zhang, J. H. Li, J. Song, J. L. Qu and W. Y. Wong, A simple Schiff base as dual-responsive fluorescent sensor for bioimaging recognition of Zn2+ and Al3+ in living cells, J. Mater. Chem. B, 2018, 6, 5435–5442 RSC.
- J. E. Kwon and S. Y. Park, Advanced organic optoelectronic materials: harnessing excited-state intramolecular proton transfer (ESIPT) process, Adv. Mater., 2011, 23, 3615–3642 CrossRef CAS.
- P. Zhou and K. Han, Unraveling the detailed mechanism of excited-state proton transfer, Acc. Chem. Res., 2018, 51, 1681–1690 CrossRef CAS.
- W. Sun, M. Li, J. Fan and X. Peng, Activity-based sensing and theranostic probes based on photoinduced electron transfer, Acc. Chem. Res., 2019, 52, 2818–2831 CrossRef CAS.
- D. Zhou, C. Sun, C. Chen, X. Cui and W. Li, Research of a highly selective fluorescent chemosensor for aluminum(III) ions based on photoinduced electron transfer, J. Mol. Struct., 2015, 1079, 315–320 CrossRef CAS.
- X. Yuan, X. Xu, C. Zhao, F. Zhang, Y. Lu, Y. Shen and C. Wang, A novel colorimetric and fluorometric fluoride ion probe based on photoinduced electron transfer signaling mechanism, Sens. Actuators, B, 2017, 253, 1096–1105 CrossRef CAS.
- Y. Qian, S. Li, G. Zhang, Q. Wang, S. Wang, H. Xu, C. Li, Y. Li and G. Yang, Aggregation-induced emission enhancement of 2-(2′-Hydroxyphenyl)benzothiazole-based excited-state intramolecular proton-transfer compounds, J. Phys. Chem. B, 2007, 111, 5861–5868 CrossRef CAS.
- H. Sun, W. H. Sun, Y. Jiang, J. H. Wei, Y. Zhao, R. Zhang and Z. H. Ni, Multi-stimuli-responsive tetraphenylethene-based thiazole compound: time-dependently enhanced blue-shift emission, reversible acidichromism and mechanochromism, Dyes Pigm., 2020, 173, 107938 CrossRef CAS.
- Y. Cao, M. Yang, Y. Wang, H. Zhou, J. Zheng, X. Zhang, J. Wu, Y. Tian and Z. Wu, Aggregation-induced and crystallization-enhanced emissions with time-dependence of a new Schiff-base family based on benzimidazole, J. Mater. Chem. C, 2014, 2, 3686–3694 RSC.
- F. Wang, M. Y. Han, K. Y. Mya, Y. Wang and Y. H. Lai, Aggregation-driven growth of size-tunable organic nanoparticles using electronically altered conjugated polymers, J. Am. Chem. Soc., 2005, 127, 10350–10355 CrossRef CAS.
- B. K. An, S. K. Kwon, S. D. Jung and S. Y. Park, Enhanced emission and its switching in fluorescent organic nanoparticles, J. Am. Chem. Soc., 2002, 124, 14410–14415 CrossRef CAS.
- B. K. An, D. S. Lee, J. S. Lee, Y. S. Park, H. S. Song and S. Y. Park, Strongly fluorescent organogel system comprising fibrillar self-assembly of a trifluoromethyl-based cyanostilbene derivative, J. Am. Chem. Soc., 2004, 126, 10232–10233 CrossRef CAS.
- L. Sheng, M. Li, S. Zhu, H. Li, G. Xi, Y. G. Li, Y. Wang, Q. Li, S. Liang, K. Zhong and S. X. Zhang, Hydrochromic molecular switches for water-jet rewritable paper, Nat. Commun., 2014, 5, 3044 CrossRef.
- G. Xi, L. Sheng, J. Du, J. Zhang, M. Li, H. Wang, Y. Ma and S. X. A. Zhang, Water assisted biomimetic synergistic process and its application in water-jet rewritable paper, Nat. Commun., 2018, 9, 4819 CrossRef.
- S. Jiang, J. Qiu, S. Chen, H. Guo and F. Yang, Double-detecting fluorescent sensor for ATP based on Cu2+ and Zn2+ response of hydrazono-bis-tetraphenylethylene, Spectrochim. Acta, Part A, 2020, 227, 117568 CrossRef CAS.
- W.-K. Dong, S. F. Akogun, Y. Zhang, Y.-X. Sun and X.-Y. Dong, A reversible “turn-on” fluorescent sensor for selective detection of Zn2+, Sens. Actuators, B, 2017, 238, 723–734 CrossRef CAS.
- M. An, B. Y. Kim, H. Seo, A. Helal and H. S. Kim, Fluorescence sensor for sequential detection of zinc and phosphate ions, Spectrochim. Acta, Part A, 2016, 169, 87–94 CrossRef CAS.
- X. L. Jin, X. L. Wu, B. Wang, P. Xie, Y. L. He, H. W. Zhou, B. Yan, J. J. Yang, W. X. Chen and X. H. Zhang, A reversible fluorescent probe for Zn2+ and ATP in living cells and in vivo, Sens. Actuators, B, 2018, 261, 127–134 CrossRef CAS.
- J. M. Jung, S. Y. Lee, E. Nam, M. H. Lim and C. Kim, A highly selective turn-on chemosensor for Zn2+ in aqueous media and living cells, Sens. Actuators, B, 2017, 244, 1045–1053 CrossRef CAS.
- J. M. Jung, D. Yun, H. Lee, K. T. Kim and C. Kim, Selective chemosensor capable of sensing both CN− and Zn2+: its application to zebrafish, Sens. Actuators, B, 2019, 297, 126814 CrossRef CAS.
- J. H. Kang, J. Han, H. Lee, M. H. Lim, K. T. Kim and C. Kim, A water-soluble fluorescence chemosensor for the sequential detection of Zn2+ and pyrophosphate in living cells and zebrafish, Dyes Pigm., 2018, 152, 131–138 CrossRef CAS.
- H. Song and Z. Zhang, A quinoline-based ratiometric fluorescent probe for discriminative detection of Zn2+ and Cd2+ with different binding modes, and its Zn2+ complex for relay sensing of pyrophosphate and adenosine triphosphate, Dyes Pigm., 2019, 165, 172–181 CrossRef CAS.
- R. Lu, S. Cui, S. Li and S. Pu, A highly sensitive and selective fluorescent sensor for Al3+ and Zn2+ based on diarylethene with an aminouracil unit, Tetrahedron, 2017, 73, 915–922 CrossRef CAS.
- Z. Wang, S. Cui, S. Qiu and S. Pu, A dual-functional fluorescent sensor based on diarylethene for Zn2+ and Al3+ in different solvents, J. Photochem. Photobiol., A, 2019, 376, 185–195 CrossRef CAS.
- J. Sun, B. Ye, G. Xia and H. Wang, A multi-responsive squaraine-based “turn on” fluorescent chemosensor for highly sensitive detection of Al3+, Zn2+ and Cd2+ in aqueous media and its biological application, Sens. Actuators, B, 2017, 249, 386–394 CrossRef CAS.
- J. J. Xiong, P. C. Huang, X. Zhou and F. Y. Wu, A highly selective and sensitive “turn-on” fluorescent probe of Cu2+ by p-dimethylaminobenzamide-based derivative and its bioimaging in living cells, Sens. Actuators, B, 2016, 232, 673–679 CrossRef CAS.
- G. G. V. Kumara, M. P. Kesavana, G. Sivaramanc, J. Annarajd, K. Anithae, A. Tamilselvif, S. Athimoolamg, B. Sridharh and J. Rajesh, Reversible NIR fluorescent probes for Cu2+ ions detection and its living cell imaging, Sens. Actuators, B, 2018, 255, 3235–3247 CrossRef.
Footnotes |
† Electronic supplementary information (ESI) available. See DOI: 10.1039/d0qm00623h |
‡ Hao Sun and Yan Jiang contributed equally to this work. |
|
This journal is © the Partner Organisations 2021 |
Click here to see how this site uses Cookies. View our privacy policy here.