DOI:
10.1039/D2QO02058K
(Research Article)
Org. Chem. Front., 2023,
10, 1880-1889
One-step synthesis of polycyclic thianthrenes from unfunctionalized aromatics by thia-APEX reactions†
Received
1st January 2023
, Accepted 13th February 2023
First published on 14th February 2023
Abstract
In this paper, thia-APEX reactions affording π-extended thianthrene derivatives from unfunctionalized aromatics are described. By utilizing S-diimidated 1,2-arenedithiols as benzene-1,2-dithiol dication synthons, new benzodithiine arms were fused to the unfunctionalized aromatic substrates in one step, affording π-extended thianthrenes in 21–87% yields. The present thia-APEX reaction occurs with equimolar amounts of aromatic substrates and S-diimidated 1,2-arenedithiols and a catalytic amount of TfOH, which is advantageous for the efficient creation of novel π-extended thianthrenes. In addition, the unique solid state packing structures and photophysical properties of the synthesized π-extended thianthrenes were elucidated in this study.
Introduction
Thianthrene is a six-membered sulfur-containing heterocyclic compound consisting of a dibenzo-fused 1,4-dithiine ring in which two sulfur atoms are embedded diagonally (Fig. 1A).1 Neutral thianthrene adopts a bent structure whose C–S–C angle is ca. 128°, whereas it reversibly transforms into a planar structure in the radical cation state.1c,2 The redox behavior and cationic-state capability of thianthrene can be utilized for supramolecular chemistry2a,3 and development of organic chemical reactions4 and cathode materials.5 Moreover, the thianthrene structure is favorable for electron-donation and intersystem crossing due to the electron-richness and heavy atom effect of sulfur atoms.6 By utilizing these functionalities, π-extended thianthrenes have been developed in recent years.3–7Fig. 1B shows the representative thianthrene-based materials with unique reactivities and optoelectronic and supramolecular properties: room-temperature phosphorescence (compound A),6d thermally activated delayed fluorescence (TADF) (compound B),6d C–H functionalization of aromatic compounds (compound C),4 semiconductivity (compound D),7l and host–guest capability for fullerenes (compound E).3
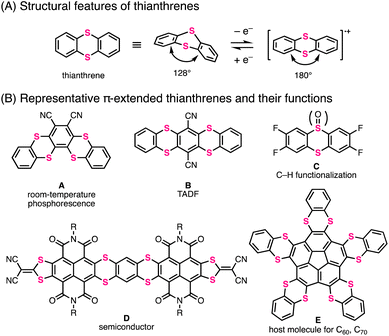 |
| Fig. 1 π-Extended thianthrenes: structures, properties, and applications. | |
To meet the high demands of π-extended thianthrenes as functional molecules, easy and rapid synthetic methods are highly desired. Representative synthetic methods of thianthrene derivatives are shown in Fig. 2A. For example, symmetric thianthrene structures can be constructed utilizing arenethiols as starting materials with fuming H2SO4 and reductants such as zinc and SnCl2 (Fig. 2A-1).8 Besides, using S2Cl2 and Lewis acids such as AlCl3, symmetric thianthrenes can be directly synthesized from unfunctionalized aromatic substrates, while the applicable substrates are limited to simple benzene derivatives.4b,9 Alternatively, SNAr-type homoannulation reactions using ortho-halogenated arenethiols and a base are also useful for the preparation of symmetric thianthrenes.10 Furthermore, cross-annulation reactions using aryldithiols and 1,2-dihaloarenes are frequently employed to construct unsymmetric thianthrene structures and large organic frameworks consisting of multiple thianthrene bridges (Fig. 2A-2).2c,5a,7c,e,h–k,11 In this method, the characteristics of multistep transformations and the low availability of arene dithiols and dihaloarenes in larger aromatic systems would diminish the synthetic utility and efficiency of the whole synthetic process. One early example of one-step synthesis of thianthrenes was reported by Sato and coworkers in 1988 (Fig. 2B).12 They used benzopentathiepin (BPT) and AlCl3 for cross-annulation of unfunctionalized arenes with a dithiine framework, achieving the one-step synthesis of various thianthrenes. However, this reaction requires excess amounts of unfunctionalized aromatics to ensure a high yield. Due to this limitation, it seems difficult to apply it to the synthesis of larger aromatics. Therefore, the development of a more efficient and rapid synthetic method with a broad scope and applicability of substrates would be needed to advance the synthetic chemistry of thianthrenes.
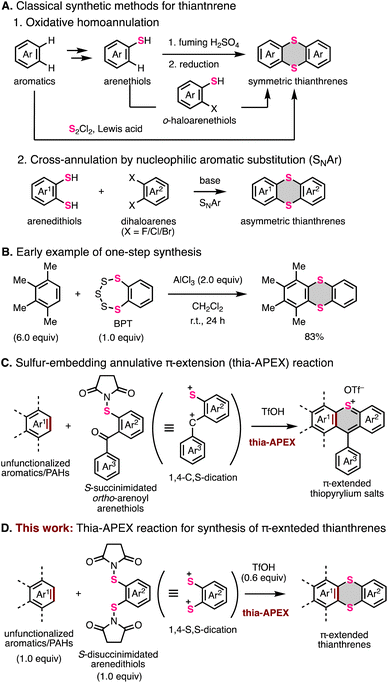 |
| Fig. 2 Conventional synthetic methods for thianthrenes and one-step synthesis using sulfur-embedding annulative π-extension (thia-APEX). | |
Previously, we have devoted our efforts toward the development of a one-step annulative π-extension (APEX)13,14 reaction and a heteroatom-embedding APEX (hetero-APEX)15 reaction for efficient and rapid access to large polycyclic aromatic hydrocarbons (PAHs), polycyclic heteroaromatics and nanographenes from readily available unfunctionalized aromatics. Recently, we also developed a sulfur-embedding APEX (thia-APEX) reaction for the one-step synthesis of π-extended thiopyrylium salts (Fig. 2C).15o In this reaction, S-succinimidated ortho-arenoyl arenethiols work as 1,4-C,S-dication π-extending agents in the presence of TfOH, and thus a one-step sequence of annulative C–S/C–C bond formation and dehydrative aromatization of unfunctionalized aromatics occurs with high regioselectivity. To further explore the potential of the thia-APEX strategy, we tested whether 1,2-arenedithiols having two succinimide groups will work as 1,4-S,S-dications, which would enable the one-step fusion of a benzodithiine ring onto unfunctionalized aromatics. Herein, we report a new thia-APEX reaction using S-diimidated 1,2-arenedithiols for the efficient one-step synthesis of π-extended thianthrenes under mild reaction conditions. Furthermore, some characteristic photophysical properties and structural features of the newly synthesized π-extended thianthrenes were elucidated by X-ray crystallographic analysis, measurements of absorption and emission, and density functional theory (DFT) calculations.
Results and discussion
First, we newly prepared S-diimidated 1,2-benzenedithiol from 1,2-benzenedithiol (2a) (see the ESI† for details) and investigated the thia-APEX reaction of 1,2-dimethoxybenzene (1a) with 2a as a π-extending agent (Table 1). When a mixture containing 1a (0.20 mmol, 1.0 equiv.), 2a (1.1 equiv.), and trifluoromethanesulfonic acid (TfOH, 2.3 equiv.) in 1,1,1,3,3,3,-hexafluoroisopropyl alcohol (HFIP, 1.0 mL) was stirred at 80 °C under air for 13 h, the desired thia-APEX reaction proceeded to afford 2,3-dimethoxythianthrene (3aa) in 38% yield along with the demethylated by-product 4 in 25% yield (entry 1). The use of an excess amount of 2a or a mixed solvent of 1,2-dichloroethane (DCE) and HFIP (v/v = 1
:
1) increased the yields of 3aa with decreased formation of 4 (entries 2 and 3). The use of DCE alone as the solvent resulted in a higher yield than the use of HFIP as the solvent (entries 4–7). In this regard, the HFIP molecule or H2O in HFIP might be involved in the deprotection of OMe to give 4. To our delight, the use of a smaller amount of TfOH (ca. 0.6 equiv.) and a stoichiometric amount of 2a was suitable for increasing the yield of product 3aa (99% NMR yield, entry 7). The use of trifluoroacetic acid (TFA) instead of TfOH dramatically decreased the yield of product 3aa, implying the need for a strong acid for activating the S–N bonds (entry 8). Elevating the temperature to 100 °C resulted in an increased yield of 4 along with the decomposition of 3a (entry 9). Finally, we found that the use of an equimolar amount of 1a and 2a in the presence of 0.6 equiv. of TfOH in DCE at room temperature (23 °C) are the best reaction conditions for the present thia-APEX reaction, and thus succeeded in exclusively obtaining 3aa in 83% isolated yield (entry 10). Compared to the early report on one-step thianthrene synthesis by Sato,12 the present thia-APEX reaction realized a 1
:
1 cross-annulation between the unfunctionalized arene and the π-extending agent with catalytic amounts of an acid even at room temperature.
Table 1 Optimization of the reaction conditions for the thia-APEX reaction of 1a with 2a
With the optimized conditions in hand, the scope of thia-APEX reactions using other aromatic substrates was examined (Fig. 3). When using S-diimidated 3,4,5,6-tetrafluorobenzene-1,2-dithiol 2b with 1a, the thia-APEX reaction was less efficient, but afforded dimethoxytetrafluorothianthrene 3ab in 32% yield. In the reaction with 2a, 1,2,3,4-tetramethylbenzene (1b) was transformed to the corresponding thianthrene 3ba in 70% yield. Unfortunately, the thia-APEX reactions of electron-deficient aromatic substrates such as 1,2-difluorobenzene and ortho-phthalodinitrile did not proceed, probably due to their lower nucleophilicities. Thianthrene 3ca was also obtained in 21% yield from phenanthrene (1c) and 2a. Although this reaction preferentially occurred at the K-region (C9, C10-position of phenanthrene: concave armchair edges in PAH), as same as the previous report,15a,o minor thia-APEX reactions at other regions and/or multi-thia-APEX reactions could result in lower yields of 3ca. In contrast, the thia-APEX reaction using 2,7-di-tert-butylphenanthrene (1d) with 2a efficiently proceeded to afford dibenzothianthrene 3da in 87% yield. Besides, by employing the methylated π-extending agent 2c instead of compound 2a, di-tert-butylphenanthrene 1d was transformed to the corresponding dibenzothianthrene 3dc in 71% yield. Thanks to the bulky tert-butyl groups, the π-extensions on other regions such as the C1–C2, C2–C3 and C3–C4 positions are considered to be prevented. Moreover, the thia-APEX reaction of C5v-symmetric corannulene (1e) with 2a mainly afforded benzodithiine-fused corannulene 3ea in 35% yield, although multi-thia-APEX products were also observed in the reaction mixture to some extent. Besides, benzodithiine-fused triphenylene 3fa was obtained from pristine triphenylene (1f) in 39% yield. The structure of 3fa was elucidated by X-ray crystallographic analysis (see the ESI†). In this reaction, there is a possibility of the formation of other regioisomers and multi-thia-APEX products, which can decrease the yield of 3fa. Furthermore, benzo[b]thiophene (1g) and 1-tosyl-1H-indole (1h) were used as heteroaromatic templates for thia-APEX reactions, and dibenzodithiinothiophene 3ga and dibenzodithiinopyrrole 3ha were obtained in 53% and 86% yields, respectively. Thia-APEX reactions also proceeded on unfunctionalized heteroaromatic rings. For example, the thia-APEX reaction of N-methyldibenzoindole 1i, which was previously synthesized by us using the APEX reaction of N-methylpyrrole,14g with 2a took place to afford 3ia in 42% yield. Finally, guaiazulene (1j) was subjected to the thia-APEX conditions, and compound 3ja was obtained in 25% yield (an average yield of four runs, see the ESI† for details) as a relatively unstable dark-green oil. In this reaction, the thia-APEX reaction selectively proceeded on the five-membered ring moiety.
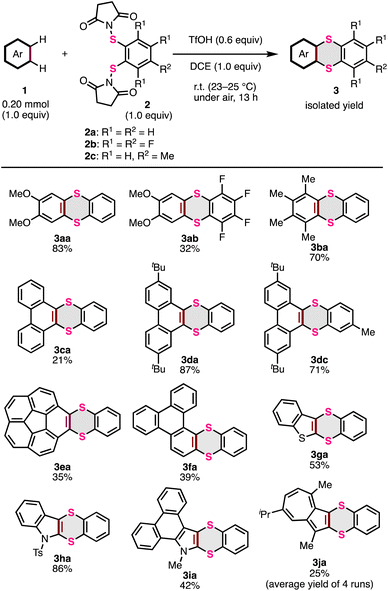 |
| Fig. 3 Scope of substrates in the thia-APEX reaction. | |
Because new π-extended thianthrenes such as 3ca, 3ea and 3ja were easily accessed by the thia-APEX reaction for the first time, the photophysical properties as well as the electronic structures of 3ca, 3ea and 3ja were also investigated. First, dibenzothianthrene 3ca showed an absorption band in the UV region, and the longest wavelength absorption maximum appeared at 331 nm in CH2Cl2 (Fig. 4, green line). Thianthrene 3ca emits strong blue-green fluorescence (fluorescence quantum yield (ΦF) = 24%) upon excitation with 330 nm light, and the emission maximum was observed at 497 nm in CH2Cl2 (Fig. 4, green dashed line). In the case of corannulene-fused thianthrene 3ea, an intense absorption maximum and a weak shoulder peak were observed at 304 nm and at 350–450 nm, respectively (Fig. 4, orange line). Moreover, thianthrene 3ea showed a broad fluorescence spectrum having a peak top at 560 nm (Fig. 4, orange dashed line) and an orange emission in CH2Cl2. Both thianthrene 3ca and 3ea showed Stokes shifts of ca. 160 nm, whose relatively large values were considered to be derived from large dynamic structural relaxations at their excited states.1,7j Furthermore, the fluorescence quantum yields of thianthrenes 3ca and 3ea were measured as 24% and 4%, respectively. Notably, the 24% quantum yield of 3ca was even larger than those of pristine phenanthrene and triphenylene (ca. 10%),16 which can be rationalized by the heavy atom effect of sulfur atoms and the prevention of quenching of fluorescence by its bent structure.17 Finally, we found that guaiazulene-fused benzodithiine 3ja shows a green color in CH2Cl2 and a weak and broad absorption band between 450–800 nm (Fig. S5†). This compound did not show fluorescence, which is strongly reflective of the nature of the guaiazulene core.
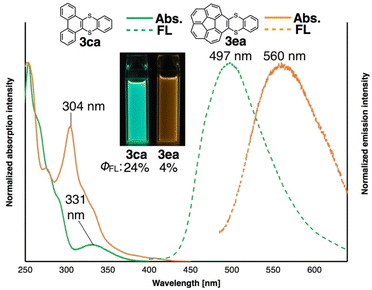 |
| Fig. 4 Absorption and emission spectra of π-extended thianthrenes 3ca and 3ea in CH2Cl2. Excitation wavelengths for fluorescence (FL) measurements: 330 nm (3ca) and 405 nm (3ea). The pictures of emission color were taken using the concentrated solutions of each compound under 365 nm of UV light irradiation. | |
Next, the absorption properties and electronic structures of π-extended thianthrenes 3ca and 3ea were evaluated by DFT and time-dependent DFT (TD-DFT) calculations using the Gaussian 16 program18 at the B3LYP/6-31+G(d,p) level of theory19 and by consideration of the solvent effect using the integral equation formalism-polarizable continuum model (IEF-PCM)20 in CH2Cl2. As shown in Fig. 5, the energy levels of the HOMO and LUMO of 3ca were calculated to be −5.90 eV and −1.74 eV, respectively. While the HOMO of 3ca is mainly localized on the 1,4-dithiine moiety, the LUMO of 3ca is localized to some extent on the phenanthrene moiety. A similar HOMO and LUMO localization tendency was also found in 3ea, and the energy levels of the HOMO and LUMO of 3ea were calculated to be −5.96 eV and −2.20 eV, respectively. Compared with the HOMO and LUMO energy levels of pristine phenanthrene (HOMO: −6.12 eV; LUMO: −1.43 eV) and corannulene (HOMO: −6.35 eV; LUMO: −2.01 eV), the effect of benzodithiine-fusion is slightly more predominant in increasing the energy levels of the HOMO (Δ = +0.22 and +0.39 eV) rather than in decreasing those of the LUMO (Δ = −0.31 and −0.19 eV). As a result, the HOMO–LUMO energy gaps of 3ca and 3ea are reduced by 0.53–0.58 eV compared with phenanthrene and corannulene. As shown in Table 2, the calculated excitation wavelength (λTD-DFT) of the HOMO → LUMO transition of thianthrene 3ca was 347.21 nm (f = 0.0993), which is consistent with the experimental longest wavelength absorption of 3ca as a weak peak (λabs = 331 nm). Besides, the second and third excitation attributed to HOMO → LUMO+1 and HOMO−1 → LUMO transitions was calculated to be 334.84 nm (f = 0.0023) and 299.47 nm (f = 0.2015), respectively. The latter allowed excitation is considered to be the shoulder absorption peak around 290 nm. With regard to 3ea, the electron transitions in three lowest energies were also estimated to be 395.93 nm (f = 0.0162, HOMO → LUMO), 388.71 nm (f = 0.0229, HOMO → LUMO+1) and 356.73 nm (f = 0.0000, HOMO−1 → LUMO+1), whose small or zero values of f show a good agreement with the weak shoulder absorptions between 350 to 450 nm. Moreover, the HOMO−1 → LUMO+1 transition (λTD-DFT = 356.73 nm, f = 0.0000) of 3ea was determined as a forbidden transition.
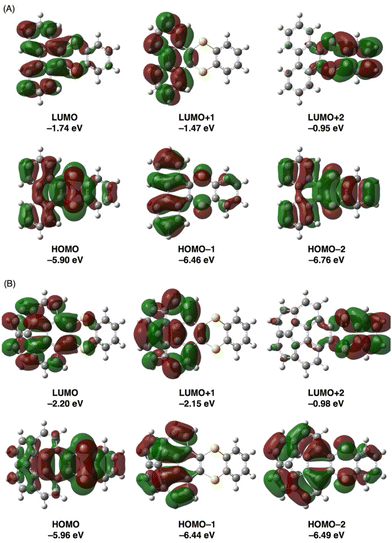 |
| Fig. 5 Representative frontier orbitals of 3ca (A) and 3ea (B) (isovalue = 0.02). Geometry optimization and energy calculations were conducted at the B3LYP/6-31+G(d,p) level of theory. | |
Table 2 Results of TD-DFT calculations at the B3LYP/6-31+G(d,p) level of theory in CH2Cl2 (IEF-PCM). λTD-DFT: estimated excitation wavelength; f: oscillator strength; λabs: the experimental observed longest wavelength absorption in CH2Cl2
|
λ
TD-DFT
|
f
|
Electronic transition (coefficient) |
λ
abs
|
3ca
|
347.21 nm |
0.0993 |
HOMO → LUMO (0.67256) |
331 nm |
334.84 nm |
0.0023 |
HOMO → LUMO+1 (0.61319) |
— |
299.47 nm |
0.2015 |
HOMO−1 → LUMO (0.56362) |
— |
3ea
|
395.93 nm |
0.0162 |
HOMO → LUMO (0.68456) |
350–450 nm |
388.71 nm |
0.0229 |
HOMO → LUMO+1 (0.65994) |
— |
356.73 nm |
0.0000 |
HOMO−1 → LUMO+1 (0.61773) |
— |
Next, we performed the structural analyses of 3ca and 3ea by X-ray crystallographic analysis. Single crystals of 3ca and 3ea were obtained by recrystallization from chloroform/pentane by a vapor diffusion method. In the X-ray crystallographic analysis of 3ca, two pairs of two molecules were observed in a unit cell, and both pairs consist of two molecules arranged in a pseudo-C2-symmetry, whose dithiine cores are directed in the same axis with ca. 127° bent angles (Fig. 6A). Each pair is directed in the opposite direction and positioned in a Ci symmetry, forming the two pseudo-enantiomeric pairs of two racemic molecules (Fig. 6A–C). Furthermore, each molecule is aligned in the direction of the b axis, forming columnar stacks, maintaining ca. 3.6 Å of intermolecular distance, which indicates weak π–π interactions of 3ca molecules.21 This characteristic columnar packing is identical to that of unsubstituted thianthrene.22 In the X-ray crystallographic analysis of 3ea, the bent angle of the thianthrene moiety in 3ea was found to be 126°, which is also closely identical to those of 3ca and unsubstituted thianthrene (Fig. 6D).1,22 Besides, the dangling benzodithiine arm is bent toward the concave face of the corannulene core, which forms a ladle-like shape. The one-dimensional columnar packings of 3ea are aligned in an antiparallel manner to the neighboring molecular columns, counteracting the dipole moments of each column (Fig. 6E). Focusing on the distances between two neighboring molecules, each corannulene core is longitudinally aligned at 3.3 Å intervals, which are smaller than the sum of the van der Waals radii of two carbon atoms, indicative of the existence of the π–π interaction.21 Furthermore, the distances between the peripheral C–H bond in the corannulene core and the carbon atoms in the dangling benzene ring are less, and ranged from 2.7 to 2.9 Å. These values are within the sum of the van der Waals radii of one hydrogen and one carbon atom, showing the existence of CH/π interactions (Fig. 6F).23 Depicting isosurfaces of non-covalent interaction (NCI) plot analysis using the NCIPLOT 4.0 program,24 green isosurfaces, which indicate weak non-covalent interactions, are visually and clearly confirmed between the corannulene cores (π–π) and between the corannulene core and the benzene ring (CH/π) (Fig. 7). Because unsubstituted corannulene in the solid state shows a disordered arrangement derived from CH/π interactions, the thianthrene arms are considered to contribute to the formation of the columnar packing structure. In addition, the columnar packing structure of thianthrene 3ea is the first example of mono-benzodithiine-fused corannulene,3,7e whereas there are a lot of other rim-region-fused or rim-region-substituted corannulene derivatives showing a similar columnar stacking ability.25 We expect that the columnar stacking achieved by the simple structural motif of benzodithiinocorannulene can provide a fruitful insight into the application of corannulene-based functional materials in organic electronics26 and supramolecular chemistry27 as well as host–guest chemistry.3
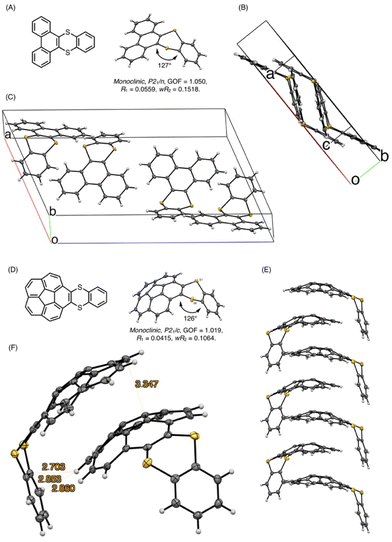 |
| Fig. 6 Structures of π-extended thianthrene 3ca: (A) structure and ORTEP drawing of 3ac with 50% probability, (B) side view of a unit cell, (C) top view of a unit cell, (D) structure and ORTEP drawing of 3ea with 50% probability, (E) ORTEP drawing of the columnar packing structure of 3ea, and (F) an extracted dimer structure with distances of π–π and CH/π interaction in units of Å. | |
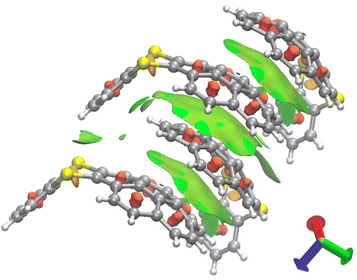 |
| Fig. 7 NCI analysis and reduced density gradient isosurface (isosurface value = 0.3) using the NCIPLOT4 program for π-extended thianthrene 3ea. Color code based on sign (λ2) ρ was: −0.07 a.u. (blue) < 0.0 a.u. (green) < 0.07 a.u. (red). Blue and red isosurfaces show regions having attractive and repulsive interactions, respectively, and green isosurfaces show weak van der Waals interactions such as π–π interaction. The structure of 3ea was extracted from the data of X-ray crystallographic analysis. | |
To obtain insights into the bent structures of thianthrenes 3ca and 3ea in crystalline and solution states, their inversion barriers were examined using DFT calculations. The structures of thianthrenes 3ca and 3ea in the ground and transition states were optimized at the B3LYP/6-31+G(d,p) level of theory. 3ca has only one stable bent structure (see the ESI†), whereas 3ea has two stable structures, a ladle-shaped one (3ea-ladle) and a spoon-shaped one (3ea-spoon), because of the inversion of the corannulene core and the benzodithiine arm (Fig. 8). 3ea-ladle was the most stable conformer in 3ea, whose structure was also observed by X-ray crystallographic analysis (Fig. 6). The inversion barriers corresponding to the inversion of benzodithiine arms were calculated to be 7.31 kcal mol−1 for TS13ca and 7.47 kcal mol−1 for TS13ea. The values of TS13ca and TS13ea are larger than that of pristine thianthrene (5.1 kcal mol−1, at the B3LYP/6-31+G(d,p) level),28 indicating that the PAH structures of phenanthrene and corannulene make the inversion barriers of benzodithiine arms higher. Furthermore, the transition state of corannulene core flipping in 3ea (TS23ea) was optimized and its barrier was calculated to be 9.72 kcal mol−1. This value is also closely identical to that of pristine corannulene.29 In conclusion, newly synthesized 3ca and 3ea were found to be hybrid molecules of thianthrene and PAHs. Their bent structures and conformational changes reflect the nature of both thianthrene and PAH cores well.
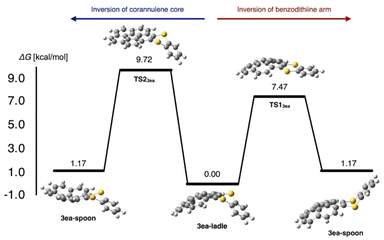 |
| Fig. 8 Two possible pathways of the structural inversion of thianthrene 3ea and their calculated barrier at the B3LYP/6-31+G(d,p) level of theory. | |
Conclusions
By using S-diimidated 1,2-benzenedithiol derivatives as new π-extending agents, thianthrenes and π-extended thianthrenes were efficiently obtained by the thia-APEX reaction of unfunctionalized aromatic substrates in one step. In addition, the characteristic photophysical and electronic properties and structural features of thia-APEX products were elucidated by UV-vis absorption and emission spectroscopy, DFT calculations and X-ray crystallographic analysis. In particular, the π-extended thianthrenes having phenanthrene and corannulene cores (3ca and 3ea) showed emission properties with larger Stokes shifts. 3ca showed higher fluorescence quantum yields than pristine phenanthrene and triphenylene. Regarding corannulene-fused thianthrene 3ea, 1D-columnar packing in the solid state was observed, which is in stark contrast to pristine corannulene. The newly developed thia-APEX reaction is expected to contribute to the rapid and efficient creation of unique π-extended thianthrenes having various aromatic cores.
Conflicts of interest
There are no conflicts to declare.
Acknowledgements
This work was supported by the JST-CREST program (JPMJCR19R1 to H. I.), the JSPS KAKENHI (JP19H05463 to K. I., JP20K21192 and JP21H01931 to H. I.), and the Noguchi Research Foundation and the Foundation of Public Interest of Tatematsu (to H. I.). K. P. K. gratefully acknowledges the JSPS Research Fellowship for Young Scientists (DC1). We thank Mr. Hiroki Shudo for helping with the X-ray crystallographic analysis and taking pictures of sample solutions. NMR spectroscopy and MS measurements were conducted using resources from the Chemical Instrumentation Facility (CIF), Research Center for Materials Science (RCMS), and Institute of Transformative Bio-Molecules (ITbM), Nagoya University. Calculations were performed using the resources of the Research Center for Computational Science, Okazaki, Japan (Projects: 21-IMS-C070 and 22-IMS-C069) and the SuperComputer System, Institute for Chemical Research, Kyoto University.
References
-
(a) K. Kobayashi and C. L. J. Gajurel, The Chemistry of 1, 4-Dithiins, Sulphur Chem., 1986, 7, 123 CAS;
(b) S. I. Etkind and T. M. Swager, The Properties, Synthesis, and Materials Applications of 1,4-Dithiins and Thianthrenes, Synthesis, 2022, 54, 4843 CrossRef CAS;
(c) K. L. Gallaher and S. H. Bauer, Structure and inversion potential of thianthrene, J. Chem. Soc., Faraday Trans. 2, 1975, 71, 1173 RSC;
(d) K. Kobayashi, Chemistry of 1, 4-dithiins, J. Synth. Org. Chem., Jpn., 1982, 40, 642 CrossRef CAS;
(e) A. Ito, H. Ino, H. Ichiki and K. Tanaka, Tuning Spin-State Preference by Substituents: A Case Study of Thianthrene Dication, J. Phys. Chem. A, 2002, 106, 8716 CrossRef CAS.
-
(a) J. Yuan, W. Lv, A. Li and K. Zhu, A self-assembled M2L2 truncated square and its application as a container for fullerenes, Chem. Commun., 2021, 57, 12848 RSC;
(b) M. Yamashita, H. Hayashi, M. Suzuki, D. Kuzuhara, J. Yuasa, T. Kawai, N. Aratani and H. Yamada, Bisanthra-thianthrene: synthesis, structure and oxidation properties, RSC Adv., 2016, 6, 70700 RSC;
(c) S. Tang, L. Zhang, H. Ruan, Y. Zhao and X. Wang, A Magnetically Robust Triplet Ground State Sulfur-Hydrocarbon Diradical Dication, J. Am. Chem. Soc., 2020, 142, 7340 CrossRef CAS PubMed;
(d) D. Casarini, C. Coluccini, L. Lunazzi and A. Mazzanti, J. Org. Chem., 2006, 71, 6248 CrossRef CAS PubMed;
(e) W. J. Ong, F. Bertani, E. Dalcanale and T. M. Swager, Redox Switchable Thianthrene Cavitands, Synthesis, 2017, 49, 358 CAS.
-
(a) M. Bancu, A. K. Rai, P. Cheng, R. D. Gilardi and L. T. Scott, Corannulene Polysulfides: Molecular Bowls with Multiple Arms and Flaps, Synlett, 2004, 173 CAS;
(b) P. E. Georghiou, A. H. Tran, S. Mizyed, M. Bancu and L. T. Scott, Concave polyarenes with sulfide-linked flaps and tentacles: new electron-rich hosts for fullerenes, J. Org. Chem., 2005, 70, 6158 CrossRef CAS PubMed.
-
(a) H. Meng, M.-S. Liu and W. Shu, Organothianthrenium salts: synthesis and utilization, Chem. Sci., 2022, 13, 13690 RSC;
(b) F. Berger, M. B. Plutschack, J. Riegger, W. Yu, S. Speicher, M. Ho, N. Frank and T. Ritter, Site-selective and versatile aromatic C–H functionalization by thianthrenation, Nature, 2019, 567, 223 CrossRef CAS PubMed; J. Chen, J. Li, M. B. Plutschack, F. Berger and T. Ritter, Regio- and Stereoselective Thianthrenation of Olefins To Access Versatile Alkenyl Electrophiles, Angew. Chem., Int. Ed., 2020, 59, 5616 Search PubMed;
(c) H. Jia and T. Ritter, α-Thianthrenium Carbonyl Species: The Equivalent of an α-Carbonyl Carbocation, Angew. Chem., Int. Ed., 2022, 61, e202208978 CAS;
(d) H. Jia, A. P. Häring, F. Berger, L. Zhang and T. Ritter, Trifluoromethyl Thianthrenium Triflate: A Readily Available Trifluoromethylating Reagent with Formal CF3+, CF3˙, and CF3− Reactivity, J. Am. Chem. Soc., 2021, 143, 7623 CrossRef CAS PubMed;
(e) F. Juliá, Q. Shao, M. Duan, M. B. Plutschack, F. Berger, J. Mateos, C. Lu, X.-S. Xue, K. N. Houk and T. Ritter, High Site Selectivity in Electrophilic Aromatic Substitutions: Mechanism of C–H Thianthrenation, J. Am. Chem. Soc., 2021, 143, 16041 CrossRef PubMed;
(f) F. Juliá, J. Yan, F. Paulus and T. Ritter, Vinyl Thianthrenium Tetrafluoroborate: A Practical and Versatile Vinylating Reagent Made from Ethylene, J. Am. Chem. Soc., 2021, 143, 12992 CrossRef PubMed;
(g) D. E. Holst, D. J. Wang, M. J. Kim, I. A. Guzei and Z. K. Wickens, Aziridine synthesis by coupling amines and alkenes via an electrogenerated dication, Nature, 2021, 596, 74 CrossRef CAS PubMed;
(h) D. J. Wang, K. Targos and Z. K. Wickens, J. Am. Chem. Soc., 2021, 143, 21503 CrossRef CAS PubMed;
(i) Y. Xiong, X. Zhang, H.-M. Guo and X. Wu, Photoredox/persistent radical cation dual catalysis for alkoxy radical generation from alcohols, Org. Chem. Front., 2022, 9, 3532 RSC.
-
(a) S. Haldar, M. Wang, P. Bhauriyal, A. Hazra, A. H. Khan, V. Bon, M. A. Isaacs, A. De, L. Shupletsov, T. Boenke, J. Grothe, T. Heine, E. Brunner, X. Feng, R. Dong, A. Schneemann and S. Kaskel, Porous Dithiine-Linked Covalent Organic Framework as a Dynamic Platform for Covalent Polysulfide Anchoring in Lithium–Sulfur Battery Cathodes, J. Am. Chem. Soc., 2022, 144, 9101 CrossRef CAS PubMed;
(b) M. Fu, C. Zhang, Y. Chen, K. Fan, G. Zhang, J. Zou, Y. Gao, H. Dai, X. Wang and C. Wang, A thianthrene-based small molecule as a high-potential cathode for lithium–organic batteries, Chem. Commun., 2022, 58, 11993 RSC;
(c) M. Yao, N. Taguchi, H. Ando, N. Takeichi and T. Kiyobayashi, Improved gravimetric energy density and cycle life in organic lithium-ion batteries with naphthazarin-based electrode materials, Commun. Mater., 2020, 1, 70 CrossRef.
-
(a) P. Pander, A. Swist, R. Turczyn, S. Pouget, D. Djurado, A. Lazauskas, R. Pashazadeh, J. V. Grazulevicius, R. Motyka, A. Klimash, P. J. Skabara, P. Data, J. Soloducho and F. B. Dias, Observation of Dual Room Temperature Fluorescence–Phosphorescence in Air, in the Crystal Form of a Thianthrene Derivative, J. Phys. Chem. C, 2018, 122, 24958 CrossRef CAS;
(b) Y. Wen, H. Liu, S.-T. Zhang, G. Pan, Z. Yang, T. Lu, B. Li, J. Cao and B. Yang, Modulating Room Temperature Phosphorescence by Oxidation of Thianthrene to Achieve Pure Organic Single-Molecule White-Light Emission, CCS Chem., 2020, 2, 1940 Search PubMed;
(c) Z. Yang, S. Zhao, X. Zhang, M. Liu, H. Liu and B. Yang, Efficient Room-Temperature Phosphorescence from Discrete Molecules Based on Thianthrene Derivatives for Oxygen Sensing and Detection, Front. Chem., 2022, 9, 810304 CrossRef PubMed;
(d) M. Li, W. Xie, X. Cai, X. Peng, K. Liu, Q. Gu, J. Zhou, W. Qiu, Z. Chen, Y. Gan and S.-J. Su, Molecular Engineering of Sulfur-Bridged Polycyclic Emitters Towards Tunable TADF and RTP Electroluminescence, Angew. Chem., Int. Ed., 2022, 61, e202209343 CAS;
(e) J. Chen, H. Tian, Z. Yang, J. Zhao, Z. Yang, Y. Zhang, M. P. Aldred and Z. Chi, A Multi-Stimuli-Responsive Molecule with Responses to Light, Oxygen, and Mechanical Stress through Flexible Tuning of Triplet Excitons, Adv. Opt. Mater., 2021, 9, 2001550 CrossRef CAS;
(f) A. Tomkeviciene, T. Matulaitis, M. Guzauskas, V. Andruleviciene, D. Volyniuk and J. V. Grazulevicius, Thianthrene and acridan-substituted benzophenone or diphenylsulfone: Effect of triplet harvesting via TADF and phosphorescence on efficiency of all-organic OLEDS, Org. Electron., 2019, 70, 227 CrossRef CAS.
- Representative applications of (π-extended) thianthrenes:
(a) A. Bartl and J. Fröhner, New charge-transfer complexes with polyconjugated sulfur compounds, Synth. Met., 1992, 51, 115 CrossRef CAS;
(b) B. Fiedler, E. Rojo-Wiechel, J. Klassen, J. Simon, J. Beck and M. Sokolowski, Ordered structures of two sulfur containing donor molecules on the Au(111) surface, Surf. Sci., 2012, 606, 1855 CrossRef CAS;
(c) Z. Liu, W. Wang, W. Xu, H. Chen, X. Zhang, T. Ren, X. Wang, J. Zhao and J. Xiao, Synthesis, characterization and photocurrent behavior of asymmetrical heterotwistacenes, Dyes Pigm., 2015, 115, 143 CrossRef CAS;
(d) P. A. Denis, Theoretical characterization of existing and new fullerene receptors, RSC Adv., 2013, 3, 25296 RSC;
(e) V. Barát, M. Budanović, D. Halilovic, J. Huh, R. D. Webster, S. H. Mahadevegowda and M. C. Stuparu, A general approach to non-fullerene electron acceptors based on the corannulene motif, Chem. Commun., 2019, 55, 3113 RSC;
(f) T. Ren, J. Xiao, W. Wang, W. Xu, S. Wang, X. Zhang, X. Wang, H. Chen, J. Zhao and L. Jiang, Synthesis, Crystal Structures, Optical Properties, and Photocurrent Response of Heteroacene Derivatives, Chem. – Asian J., 2014, 9, 1943 CrossRef CAS PubMed;
(g) S. Riebe, S. Adam, B. Roy, I. Maisuls, C. G. Daniliuc, J. Dubbert, C. A. Strassert, I. Schapiro and J. Voskuhl, Bridged Aromatic Oxo- and Thioethers with Intense Emission in Solution and the Solid State, Chem. – Asian J., 2021, 16, 2307 CrossRef CAS PubMed;
(h) S. I. Etkind, J. Lopez, Y. G. Zhu, J.-H. Fang, W. J. Ong, Y. Shao-Horn and T. M. Swager, Thianthrene-Based Bipolar Redox-Active Molecules Toward Symmetric All-Organic Batteries, ACS Sustainable Chem. Eng., 2022, 10, 11739 CrossRef CAS;
(i) Z. Nelson, L. Delage-Laurin, M. D. Peeks and T. M. Swager, Large Faraday Rotation in Optical-Quality Phthalocyanine and Porphyrin Thin Films, J. Am. Chem. Soc., 2021, 143, 7096 CrossRef CAS PubMed;
(j) W. J. Ong and T. M. Swager, Dynamic self-correcting nucleophilic aromatic substitution, Nat. Chem., 2018, 10, 1023 CrossRef CAS PubMed;
(k) T. Jin, L. Kunze, S. Breimaier, M. Bolte, H.-W. Lerner, F. Jäkle, R. F. Winter, M. Braun, J.-M. Mewes and M. Wagner, Exploring Structure–Property Relations of B,S-Doped Polycyclic Aromatic Hydrocarbons through the Trinity of Synthesis, Spectroscopy, and Theory, J. Am. Chem. Soc., 2022, 144, 13704 CrossRef CAS PubMed;
(l) H. Luo, D. He, Y. Zhang, S. Wang, H. Gao, J. Yan, Y. Cao, Z. Cai, L. Tan, S. Wu, L. Wang and Z. Liu, Synthesis of Heterocyclic Core-Expanded Bis-Naphthalene Tetracarboxylic Diimides, Org. Lett., 2019, 21, 9734 CrossRef CAS PubMed.
-
(a) J. B. Edson and D. M. Knauss, Thianthrene as an activating group for the synthesis of poly(aryl ether thianthrene)s by nucleophilic aromatic substitution, Polym. Chem., 2004, 42, 6353 CrossRef CAS;
(b) K. Fries, H. Koch, H. Slukenbrock and Z. K. des Thianthrens, Justus Liebigs Ann. Chem., 1929, 468, 162 CrossRef CAS.
-
(a) O. A. Rakitin and L. S. Konstantinova, Chapter 4 Sulfur Monochloride in the Synthesis of Heterocyclic Compounds, Adv. Heterocycl. Chem., 2008, 96, 175 CrossRef CAS;
(b) T. Link, M. Oberjat and G. Klar, Dithiines Annulated by heterocycles. Part 2. Reaction of Disulfur Dichloride with Various Quinolines, J. Chem. Res., Synop., 1997, 435 RSC;
(c) F. Mostaghimi, E. Lork, I. Hong, T. L. Roemmele, R. T. Boeré, S. Mebs and J. Beckmann, The reaction
of phenoxatellurine with single-electron oxidizers revisited, New J. Chem., 2019, 43, 12754 RSC;
(d) A. Spurg, G. Schnakenburg and S. R. Waldvogel, Oxidative Coupling of Diaryldisulfides by MoCl5 to Thianthrenes, Chem. – Eur. J., 2009, 15, 13313 CrossRef CAS PubMed;
(e) Z. S. Ariyan and R. L. Martin, Novel macrocyclic polysulphur compounds. 7,15,17,19-Tetra-alkoxy-2,3,4,5,10,11,12,13-octathiatricyclo-[12,2,2,2]eicosa-6,8,14,16,17,19-hexaenes and 2,3,7,8-tetra-alkoxythianthrens; products of the catalysed reaction of aromatic ethers and sulphur monochloride, J. Chem. Soc., Perkin Trans. 1, 1972, 1687 RSC;
(f) S. Wang, J. Yuan, J. Xie, Z. Lu, L. Jiang, Y. Mu, Y. Huo, Y. Tsuchido and K. Zhu, Sulphur-Embedded Hydrocarbon Belts: Synthesis, Structure and Redox Chemistry of Cyclothianthrenes, Angew. Chem., Int. Ed., 2021, 60, 18443 CrossRef CAS PubMed.
- I. W. J. Still and V. A. Sayeed, A Versatile Synthetic Route to Substituted Thianthrenes, Synth. Commun., 1983, 13, 1181 CrossRef CAS.
-
(a) P. Preedasuriyachai, P. Charoonniyomporn, O. Karoonnirun, T. Thongpanchang and Y. Thebtaranonth, A novel one-pot synthesis of derivatives of aryldioxins and aryldithiins, Tetrahedron Lett., 2004, 45, 1343 CrossRef CAS;
(b) C. Marti, J. Irurre, A. Alvarez-Larena, J. F. Piniella, E. Brillas, L. Fajarí, C. Alemán and L. Juliá, Synthesis, Properties, and X-ray Structure of 6-Aza-5,7,12,14-tetrathiapentacene as a Novel Polyheterocyclic Electron Donor, and Related Compounds, J. Org. Chem., 1994, 59, 6200 CrossRef CAS.
- R. Sato, A. Onodera, T. Goto and M. Saito, Chemistry on Benzopentathiepin. Reactios of Benzopentathiepin with Aromatic Compounds in the Presence of Lewis Acid, Heterocycles, 1988, 27, 2563 CrossRef CAS.
-
(a) H. Ito, K. Ozaki and K. Itami, Annulative π-Extension (APEX): Rapid Access to Fused Arenes, Heteroarenes, and Nanographenes, Angew. Chem., Int. Ed., 2017, 56, 11144 CrossRef CAS PubMed;
(b) H. Ito, Y. Segawa, K. Murakami and K. Itami, Polycyclic Arene Synthesis by Annulative π-Extension, J. Am. Chem. Soc., 2019, 141, 3 CrossRef CAS PubMed.
- Our previous examples of APEX reactions:
(a) K. Ozaki, K. Kawasumi, M. Shibata, H. Ito and K. Itami, One-shot K-region-selective annulative π-extension for nanographene synthesis and functionalization, Nat. Commun., 2015, 6, 6251 CrossRef CAS PubMed;
(b) W. Matsuoka, H. Ito and K. Itami, Rapid Access to Nanographenes and Fused Heteroaromatics by Palladium-Catalyzed Annulative π-Extension Reaction of Unfunctionalized Aromatics with Diiodobiaryls, Angew. Chem., Int. Ed., 2017, 56, 12224 CrossRef CAS PubMed;
(c) W. Matsuoka, H. Ito, D. Sarlah and K. Itami, Diversity-oriented synthesis of nanographenes enabled by dearomative annulative π-extension, Nat. Commun., 2021, 12, 3940 CrossRef CAS PubMed;
(d) T. Nakamuro, K. Kumazawa, H. Ito and K. Itami, Bay-Region-Selective Annulative π-Extension (APEX) of Perylene Diimides with Arynes, Synlett, 2019, 30, 423 CrossRef CAS;
(e) K. Ozaki, K. Murai, W. Matsuoka, K. Kawasumi, H. Ito and K. Itami, One-Step Annulative π-Extension of Alkynes with Dibenzosiloles Dibenzogermoles by Palladium/o-chloranil Catalysis, Angew. Chem., Int. Ed., 2017, 56, 1361 CrossRef CAS PubMed;
(f) K. Ozaki, W. Matsuoka, H. Ito and K. Itami, Annulative π-Extension (APEX) of Heteroarenes with Dibenzosiloles and Dibenzogermoles by Palladium/o-Chloranil Catalysis, Org. Lett., 2017, 19, 1930 CrossRef CAS PubMed;
(g) H. Kitano, W. Matsuoka, H. Ito and K. Itami, Annulative π-extension of indoles and pyrroles with diiodobiaryls by Pd catalysis: rapid synthesis of nitrogen-containing polycyclic aromatic compounds, Chem. Sci., 2018, 9, 7556 RSC;
(h) W. Matsuoka, K. P. Kawahara, H. Ito, D. Sarlah and K. Itami, J. Am. Chem. Soc., 2023, 145, 658 CrossRef CAS PubMed.
- Representative examples of the hetero-APEX reaction:
(a) K. P. Kawahara, W. Matsuoka, H. Ito and K. Itami, Synthesis of Nitrogen-Containing Polyaromatics by Aza-Annulative π-Extension of Unfunctionalized Aromatics, Angew. Chem., Int. Ed., 2020, 59, 6383 CrossRef CAS PubMed;
(b) J. Yan, A. P. Pulis, G. J. P. Perry and D. J. Procter, Metal-Free Synthesis of Benzothiophenes by Twofold C–H Functionalization: Direct Access to Materials-Oriented Heteroaromatics, Angew. Chem., Int. Ed., 2019, 58, 15675 CrossRef CAS PubMed;
(c) E. Schendera, L.-N. Unkel, P. P. H. Quyen, G. Salkewitz, F. Hoffmann, A. Villinger and M. Brasholz, Visible-Light-Mediated Aerobic Tandem Dehydrogenative Povarov/Aromatization Reaction: Synthesis of Isocryptolepines, Chem. – Eur. J., 2020, 26, 269 CrossRef CAS PubMed;
(d) Z. Yu, Y. Zhang, J. Tang, L. Zhang, Q. Liu, Q. Li, G. Gao and J. You, Ir-Catalyzed Cascade C–H Fusion of Aldoxime Ethers and Heteroarenes: Scope and Mechanisms, ACS Catal., 2020, 10, 203 CrossRef CAS;
(e) S. Tokita, K. Hiruta, K. Kitahara and H. Nishi, Diels-Alder Reaction of Dialkyl Diazenedicarboxylates with Perylenes; A New Synthesis of Polycyclic Aromatic Pyridazines, Synthesis, 1982, 229 CrossRef CAS;
(f) S. Maiti, T. K. Achar and P. Mal, An Organic Intermolecular Dehydrogenative Annulation Reaction, Org. Lett., 2017, 19, 2006 CrossRef CAS PubMed;
(g) R. Regar, R. Mishra, P. K. Mondal and J. Sankar, Metal-free Annulation at the Ortho- and Bay-Positions of Perylene Bisimide Leading to Lateral π-Extension with Strong NIR Absorption, J. Org. Chem., 2018, 83, 9547 CrossRef CAS PubMed;
(h) Y. Tokimaru, S. Ito and K. Nozaki, Synthesis of Pyrrole-Fused Corannulenes: 1,3-Dipolar Cycloaddition of Azomethine Ylides to Corannulene, Angew. Chem., Int. Ed., 2017, 56, 15560 CrossRef CAS PubMed;
(i) A. Qarah, M. Gasonoo, D. Do and D. A. Klumpp, Superacid-promoted synthesis of polychlorinated dibenzofurans, Tetrahedron Lett., 2016, 57, 3711 CrossRef CAS;
(j) C. Chen, Y. Wang, X. Shi, W. Sun, J. Zhao, Y.-P. Zhu, L. Liu and B. Zhu, Palladium-Catalyzed C-2 and C-3 Dual C–H Functionalization of Indoles: Synthesis of Fluorinated Isocryptolepine Analogues, Org. Lett., 2020, 22, 4097 CrossRef CAS PubMed;
(k) M. Wang, L. Kong, F. Wang and X. Li, Rhodium-Catalyzed Amination and Annulation of Arenes with Anthranils: C-H Activation Assisted by Weakly Coordinating Amides, Adv. Synth. Catal., 2017, 359, 4411 CrossRef CAS;
(l) A. V. Aksenov, N. A. Aksenov, A. S. Lyakhovnenko and I. V. Aksenova, Regioselectivity Change in the Reaction of Naphthalene and 2-Naphthyl Ethers with 1,3,5-Triazines Depending on Reagent Quantities, Synthesis, 2009, 3439 CrossRef CAS;
(m) M. A. Bashir, Y. Zhang, H. Yu, B. Wang, W. Zhao and F. Zhong, Bimetallic copper/cobalt-cocatalyzed double aerobic phenol oxidation/cyclization toward π-extended benzofuro[2,3-b]indoles as electron donors for electroluminescence, Green Chem., 2021, 23, 5031 RSC;
(n) S. C. Benson, J. L. Gross and J. K. Snyder, Indole as a dienophile in inverse electron demand Diels-Alder reactions: reactions with 1,2,4-triazines and 1,2-diazines, J. Org. Chem., 1990, 55, 3257 CrossRef CAS;
(o) K. P. Kawahara, W. Matsuoka, H. Ito and K. Itami, Rapid access to polycyclic thiopyrylium compounds from unfunctionalized aromatics by thia-APEX reaction, Chem. Commun., 2023, 59, 1157 RSC.
-
(a) F. Masetti and U. Mazzucato, Heavy atom effect on the luminescence of phenanthrene, J. Lumin., 1971, 4, 8 CrossRef CAS;
(b) C. A. Parker and C. G. Hatchard, Analyst, 1962, 87, 664 RSC;
(c) S. Kyushin, H. Fujii, K. Negishi and H. Matsumoto, Improvement of the fluorescence quantum yield of triphenylene by the rotational effect of 4-(trimethylsilyl)phenyl groups, Mendeleev Commun., 2022, 32, 87 CrossRef CAS.
- The fluorescent quantum yields of the commercially available
pristine phenanthrene and corannulene in CH2Cl2 were measured using a spectrofluorophotometer in our laboratory (see the ESI†), and determined to be 3% and 1%, respectively. These values were lower than those reported in the literature (14% and 7%): ref. 16a and b and J. Mack, P. Vogel, D. Jones, N. Kavala and A. Suttona, The development of corannulene-based blue emitters, Org. Biomol. Chem., 2007, 5, 2448 RSC.
-
M. J. Frisch, G. W. Trucks, H. B. Schlegel, G. E. Scuseria, M. A. Robb, J. R. Cheeseman, G. Scalmani, V. Barone, G. A. Petersson, H. Nakatsuji, X. Li, M. Caricato, A. V. Marenich, J. Bloino, B. G. Janesko, R. Gomperts, B. Mennucci, H. P. Hratchian, J. V. Ortiz, A. F. Izmaylov, J. L. Sonnenberg, D. Williams-Young, F. Ding, F. Lipparini, F. Egidi, J. Goings, B. Peng, A. Petrone, T. Henderson, D. Ranasinghe, V. G. Zakrzewski, J. Gao, N. Rega, G. Zheng, W. Liang, M. Hada, M. Ehara, K. Toyota, R. Fukuda, J. Hasegawa, M. Ishida, T. Nakajima, Y. Honda, O. Kitao, H. Nakai, T. Vreven, K. Throssell, J. A. Montgomery Jr., J. E. Peralta, F. Ogliaro, M. J. Bearpark, J. J. Heyd, E. N. Brothers, K. N. Kudin, V. N. Staroverov, T. A. Keith, R. Kobayashi, J. Normand, K. Raghavachari, A. P. Rendell, J. C. Burant, S. S. Iyengar, J. Tomasi, M. Cossi, J. M. Millam, M. Klene, C. Adamo, R. Cammi, J. W. Ochterski, R. L. Martin, K. Morokuma, O. Farkas, J. B. Foresman and D. J. Fox, Gaussian 16, RevisionC.01, Gaussian, Inc., Wallingford, CT, 2016 Search PubMed.
-
(a) A. D. Becke, Density-functional thermochemistry. III. The role of exact exchange, J. Chem. Phys., 1993, 98, 5648 CrossRef CAS;
(b) C. Lee, W. Yang and R. G. Parr, Development of the Colle-Salvetti correlation-energy formula into a functional of the electron density, Phys. Rev. B: Condens. Matter Mater. Phys., 1988, 37, 785 CrossRef CAS PubMed.
- B. Mennucci, E. Cancès and J. Tomasi, Evaluation of Solvent Effects in Isotropic and Anisotropic Dielectrics and in Ionic Solutions with a Unified Integral Equation Method: Theoretical Bases, Computational Implementation, and Numerical Applications, J. Phys. Chem. B, 1997, 101, 10506 CrossRef CAS.
- The commonly known range of interplanar distances by π–π stacking is 3.3–3.8 Å according to a reference: C. J. Janiak, A critical account on π–π stacking in metal complexes with aromatic nitrogen-containing ligands, Chem. Soc., Dalton Trans., 2000, 3885 RSC.
-
(a) I. Rowe and B. Post, The crystal structure of thianthrene, Acta Crystallogr., 1958, 11, 372 CrossRef CAS;
(b) H. Lynton and E. G. Cox, The crystal and molecular structure of thianthrene, J. Chem. Soc., 1956, 4886 RSC.
-
(a) M. Nishio, CrystEngComm, 2004, 6, 130 RSC;
(b) G. Aragay, D. Hernández, B. Verdejo, E. C. Escudero-Adán, M. Martínez and P. Ballester, Quantification of CH-π Interactions Using Calix[4]pyrrole Receptors as Model Systems, Molecules, 2015, 20, 16672 CrossRef CAS PubMed.
-
(a) E. R. Johnson, S. Keinan, P. Mori-Sánchez, J. Contreras-García, A. J. Cohen and W. Yang, Revealing Noncovalent Interactions, J. Am. Chem. Soc., 2010, 132, 6498 CrossRef CAS PubMed;
(b) J. Contreras-García, E. R. Johnson, S. Keinan, R. Chaudret, J.-P. Piquemal, D. N. Beratan and W. Yang, NCIPLOT: A Program for Plotting Noncovalent Interaction Regions, J. Chem. Theory Comput., 2011, 7, 625 CrossRef PubMed;
(c) R. A. Boto, F. Peccati, R. Laplaza, C. Quan, A. Carbone, J.-P. Piquemal, Y. Maday and J. Contreras-García, NCIPLOT4: Fast, Robust, and Quantitative Analysis of Noncovalent Interactions, J. Chem. Theory Comput., 2020, 16, 4150 CrossRef CAS PubMed.
-
(a) S. Sanyal, A. K. Manna and S. K. Pati, Functional Corannulene: Diverse Structures, Enhanced Charge Transport, and Tunable Optoelectronic Properties, ChemPhysChem, 2014, 15, 885 CrossRef CAS PubMed;
(b) T. Guo, A. Li, J. Xu, K. K. Baldridge and J. Siegel, Enantiopure C5 Pentaindenocorannulenes: Chiral Graphenoid Materials, Angew. Chem., Int. Ed., 2021, 60, 25809 CrossRef CAS PubMed;
(c) B. M. Schmidt, B. Topolinski, P. Roesch and D. Lentz, Electron-poor N-substituted imide-fused corannulenes, Chem. Commun., 2012, 48, 6520 RSC;
(d) A. Steinauer, A. M. Butterfield, A. Linden, A. Molina-Ontario, D. C. Buck, R. W. Cotta, L. Echegoyen, K. K. Baldridge and J. S. Siegel, Tunable Photochemical/Redox Properties of (Phenylthio)ncorannulenes: Application to a Photovoltaic Device, J. Braz. Chem. Soc., 2016, 27, 1866 CAS;
(e) Non-substituted corannulene is favored to form a disordered close-packed structure according to this paper: R. L. Parc, P. Hermet, S. Rols, D. Maurin, L. Alvarez, A. Ivanov, J. M. Quimby, C. G. Hanley, L. T. Scott and J.-L. Bantignies, New Insights on Vibrational Dynamics of Corannulene, J. Phys. Chem. C, 2012, 116, 25089 CrossRef.
-
(a) R. Chen, R.-Q. Lu, P.-C. Shi and X.-Y. Cao, Corannulene derivatives for organic electronics: From molecular engineering to applications, Chin. Chem. Lett., 2016, 27, 1175 CrossRef CAS;
(b) X. Chena, H. Wanga, B. Wanga, Y. Wanga, X. Jinb and F.-Q. Bai, Charge transport properties in organic D-A mixed-stack complexes based on corannulene and sumanene derivatives-a theoretical study, Org. Electron., 2019, 68, 35 CrossRef;
(c) K. Menekse, R. Renner, B. Mahlmeistera, M. Stoltea and F. Würthner, Bowl-Shaped Naphthalimide-Annulated Corannulene as Nonfullerene Acceptor in Organic Solar Cells, Org. Mater., 2020, 2, 229 CrossRef CAS.
-
(a) J. Kang, D. Miyajima, T. Mori, Y. Inoue, Y. Itoh and T. Aida, Noncovalent assembly. A rational strategy for the realization of chain-growth supramolecular polymerization, Science, 2015, 347, 646 CrossRef CAS PubMed;
(b) D. Miyajima, K. Tashiro, F. Araoka, H. Takezoe, J. Kim, K. Kato, M. Takata and T. Aida, Liquid Crystalline Corannulene Responsive to Electric Field, J. Am. Chem. Soc., 2009, 131, 44 CrossRef CAS PubMed.
-
(a) S. Kim, Y. Kwon, J.-P. Lee, S.-Y. Choi and J. Choo, A theoretical investigation into the conformational changes of dibenzo-p-dioxin, thianthrene, and selenanthrene, J. Mol. Struct., 2003, 655, 451 CrossRef CAS;
(b) J. Chickos and K. Mislow, Conformational Flexibility of Thianthrene and Its Oxides, J. Am. Chem. Soc., 1967, 89, 4815 CrossRef CAS.
- P. U. Biedermann, S. Pogodin and I. Agranat, Inversion Barrier of Corannulene. A Benchmark for Bowl-to-Bowl Inversions in Fullerene Fragments, J. Org. Chem., 1999, 64, 3655 CrossRef CAS PubMed.
Footnote |
† Electronic supplementary information (ESI) available: Syntheses, NMR, UV-vis absorption, emission, DFT calculations and crystallographic table. CCDC 2223785 (3ea), 2223786 (3ca) and 2241075 (3fa). For ESI and crystallographic data in CIF or other electronic format see DOI: https://doi.org/10.1039/d2qo02058k |
|
This journal is © the Partner Organisations 2023 |
Click here to see how this site uses Cookies. View our privacy policy here.