DOI:
10.1039/C4QO00339J
(Research Article)
Org. Chem. Front., 2015,
2, 336-339
Phase-transfer-catalyzed asymmetric desymmetrizations of cyclopentanones†‡
Received
20th December 2014
, Accepted 30th January 2015
First published on 3rd February 2015
Abstract
Although various highly enantioselective transformations have been achieved under phase-transfer conditions, asymmetric desymmetrization of cyclic ketones is still a challenging reaction in the phase-transfer chemistry. In this context, we have developed asymmetric desymmetrization of meso-epoxy ketones under phase-transfer conditions. The epoxide-opening reaction was efficiently promoted by chiral bifunctional phase-transfer catalysts to give the corresponding cyclic ketones with high enantioselectivities. Furthermore, this reaction system was applied to asymmetric isomerization of cyclic ketone.
Introduction
Phase-transfer catalysis has long been recognized as a versatile method for organic synthesis in the chemical community,1 and asymmetric phase-transfer reactions catalyzed by chiral quaternary onium salts constitute a topic of great scientific interest, particularly in the last 20 years. Notable achievements have been made recently that have enabled an array of phase-transfer-catalyzed transformations in a highly enantioselective manner under mild conditions.2 However, some reactions, such as the asymmetric desymmetrizations3 of cyclic ketones by using phase-transfer catalysis, remained challenging (Scheme 1). Although several approaches for the asymmetric desymmetrization of cyclohexanones have been reported, the enantioselectivities of the resulting chiral cyclic products were moderate at best (Scheme 1a and b).4 In this context, we are interested in the development of more efficient approaches for the asymmetric desymmetrization of cyclic ketones under phase-transfer conditions. Here we wish to report a valuable example on highly enantioselective desymmetrization of cyclopentanones catalyzed by chiral bifunctional quaternary ammonium bromides (Scheme 1c).5
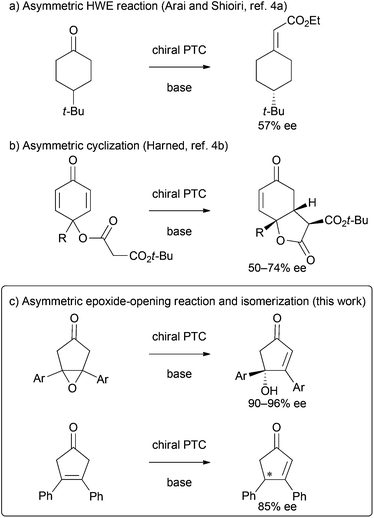 |
| Scheme 1 Phase-transfer-catalyzed asymmetric desymmetrizations of cyclic ketones. | |
Results and discussion
We first examined the asymmetric desymmetrization of meso-epoxy ketone 1a6 promoted by binaphthyl-modified chiral quaternary onium salts 3–5 as promising chiral phase-transfer catalysts (Table 1).2 Attempted reaction of 1a in aqueous K2CO3–toluene biphasic solution under the influence of chiral phase-transfer catalyst (S)-37 at 0 °C for 24 h afforded the corresponding chiral cyclopentenone 2a in high yield with low enantioselectivity (5% ee, entry 1). To improve the enantioselectivity of this reaction, we next examined bifunctional catalysts (S)-4a and 4b8 possessing hydroxy-groups (R, R′ = H, H). Although the reaction with phenyl-substituted catalyst (S)-4a (Ar = Ph) showed poor selectivity (11% ee, entry 2), a dramatic improvement in enantioselectivity was attained by the use of 3,5-bis(trifluoromethyl)phenyl-substituted catalyst (S)-4b (Ar = 3,5-(CF3)2-C6H3), and product 2a was obtained with high enantioselectivity (92% ee, entry 3). It should be noted that the use of mono- and di-hydroxy-protected catalysts (S)-4c (R, R′ = H, Me) and 4d (R, R′ = Me, Me) caused decrease in enantioselectivities (65 and 3% ee, entries 4 and 5). These results clearly indicate that the two hydroxy-groups of catalyst (S)-4b are essential to obtain high enantioselectivity in this reaction. Although the role of hydroxy-groups in catalyst (S)-4b is not clear at this stage, one of the hydroxy-groups may interact with the enolate anion9 derived from epoxy ketone 1, and the other one may supply a proton in the epoxide-opening step (Fig. 1).5 Chiral bifunctional quaternary phosphonium bromides (S)-5a–c,10 which were developed recently by our group, did not give satisfactory results for this desymmetrization reaction (entries 6–8).11
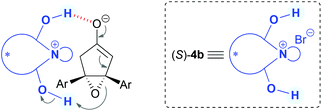 |
| Fig. 1 Proposed working model of bifunctional catalyst (S)-4b. | |
Table 1 Screening of chiral phase-transfer catalystsa
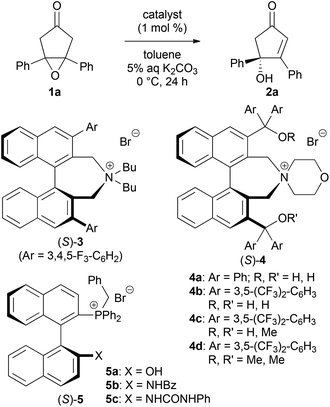
|
Entry |
Catalyst |
Yieldb (%) |
eec (%) |
Reaction conditions: 1a (0.050 mmol), 5% aq K2CO3 (0.35 mL) in the presence of catalyst (1 mol %) in toluene (0.50 mL) at 0 °C for 24 h.
Yield of isolated product.
Determined by chiral HPLC analysis.
|
1 |
(S)-3 |
99 |
−5 |
2 |
(S)-4a |
98 |
−11 |
3 |
(S)-4b |
99 |
92 |
4 |
(S)-4c |
99 |
65 |
5 |
(S)-4d |
94 |
3 |
6 |
(S)-5a |
94 |
−14 |
7 |
(S)-5b |
87 |
−8 |
8 |
(S)-5c |
98 |
10 |
With an effective desymmetrization catalyst in hand, we next studied the substrate generality of this reaction using various meso-epoxy ketones 1 (Table 2). The introduction of electron-donating and electron-withdrawing substituents on the aryl groups in 1 uniformly gave products 2b–e in high yields and enantioselectivities (90–93% ee, entries 1–4). Sterically hindered aryl group-substituted ketones 1f and 1g could also be used in this reaction, thus providing products 2f and 2g with high enantioselectivities (90–96% ee, entries 5 and 6). Unfortunately, alkyl-substituted epoxy ketones 1 gave low enantioselectivities.12
Table 2 Asymmetric desymmetrization of epoxy ketones 1a
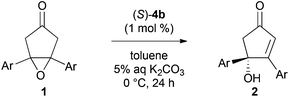
|
Entry |
Ar |
Yieldb (%) |
eec (%) |
Reaction conditions: 1 (0.050 mmol), 5% aq K2CO3 (0.35 mL) in the presence of (S)-4b (1 mol %) in toluene (0.50 mL) at 0 °C for 24 h.
Yield of isolated product.
Determined by chiral HPLC analysis.
Reaction was performed with 3 mol % of (S)-4b.
|
1 |
4-Me-C6H4 (1b) |
99 (2b) |
90 |
2 |
3-Me-C6H4 (1c) |
95 (2c) |
91 |
3 |
4-F-C6H4 (1d) |
96 (2d) |
93 |
4d |
4-Cl-C6H4 (1e) |
93 (2e) |
90 |
5 |
4-Ph-C6H4 (1f) |
93 (2f) |
96 |
6 |
2-Naphthyl (1g) |
90 (2g) |
90 |
To expand the synthetic utility of chiral bifunctional quaternary ammonium bromide (S)-4b catalyzed asymmetric desymmetrization, we examined the asymmetric isomerization13 of cyclic ketone 6 (Scheme 2). Although adjustment of the optimal reaction conditions was required, the reaction in aqueous Cs2CO3/trifluoromethylbenzene biphasic solution under the influence of (S)-4b at −20 °C for 48 h afforded the corresponding chiral cyclopentenone 7 in good yield and enantioselectivity. The reaction with other catalysts (S)-4c, 4d, and 5a gave product 7 with low to moderate yields and enantioselectivities.
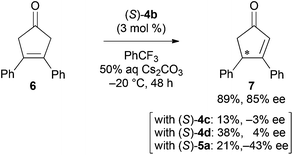 |
| Scheme 2 Asymmetric isomerization of cyclic ketone 6. | |
Conclusions
In summary, we have successfully achieved highly enantioselective desymmetrization of cyclic ketones under phase-transfer conditions. The desymmetrization of epoxy ketones 1 and ketone 6 was efficiently promoted by chiral phase-transfer catalysts. The bifunctional design of chiral quaternary ammonium bromide catalysts was essential to achieve high enantioselectivities. Further studies will be directed toward the expansion of the reaction scope.
Experimental
General procedure for the catalytic asymmetric desymmetrization of epoxy ketones 1 (Table 2)
To a solution of epoxy ketones 1 (0.050 mmol) and chiral phase-transfer catalyst (S)-4b (1 mol %, 0.00050 mmol) in toluene (0.50 mL) was added 5% aqueous K2CO3 (0.35 mL) at 0 °C. The reaction mixture was vigorously stirred for 24 h at 0 °C. The resulting mixture was quenched with saturated aqueous NH4Cl and diluted with ethyl acetate. The organic phase was separated and the aqueous phase was extracted with ethyl acetate. The combined extracts were dried over Na2SO4 and concentrated. The residue was purified by column chromatography on silica gel (hexane–ethyl acetate as the eluent) to give product 2. The enantiomeric excess of product 2 was determined by chiral HPLC analysis.
Procedure for the catalytic asymmetric isomerization of ketone 6 (Scheme 2)
To a solution of ketone 6 (0.050 mmol) and chiral phase-transfer catalyst (S)-4b (3 mol %, 0.0015 mmol) in trifluoromethylbenzene (0.50 mL) was added 50% aqueous Cs2CO3 (0.50 mL) at −20 °C. The reaction mixture was vigorously stirred for 48 h at −20 °C. The resulting mixture was quenched with saturated aqueous NH4Cl and diluted with ethyl acetate. The organic phase was separated and the aqueous phase was extracted with ethyl acetate. The combined extracts were dried over Na2SO4 and concentrated. The residue was purified by column chromatography on silica gel (hexane–ethyl acetate 10
:
1–3
:
1 as the eluent) to give product 7. The enantiomeric excess of product 7 was determined by chiral HPLC analysis.
Acknowledgements
This work was partially supported by a Grant-in-Aid for Scientific Research from JSPS and MEXT (Japan). S.B.J.K. thanks JSPS for a postdoctoral fellowship.
Notes and references
- For reviews on phase-transfer catalysis, see:
(a)
Phase Transfer Catalysis, ed. E. V. Dehmlow and S. S. Dehmlow, VCH, Weinheim, 3rd edn, 1993 Search PubMed;
(b)
Phase-Transfer Catalysis, ed. C. M. Starks, C. L. Liotta and M. Halpern, Chapman & Hall, New York, 1994 Search PubMed;
(c)
Handbook of Phase-Transfer Catalysis, ed. Y. Sasson and R. Neumann, Blackie Academic & Professional, London, 1997 Search PubMed;
(d)
Phase-Transfer Catalysis, ed. M. E. Halpern, American Chemical Society, Washington, DC, 1997 (ACS Symposium Series 659) Search PubMed.
- For recent reviews on asymmetric phase-transfer catalysis, see:
(a) M. J. O'Donnell, Aldrichimica Acta, 2001, 34, 3 Search PubMed;
(b) K. Maruoka and T. Ooi, Chem. Rev., 2003, 103, 3013 CrossRef CAS PubMed;
(c) M. J. O'Donnell, Acc. Chem. Res., 2004, 37, 506 CrossRef PubMed;
(d) B. Lygo and B. I. Andrews, Acc. Chem. Res., 2004, 37, 518 CrossRef CAS PubMed;
(e) J. Vachon and J. Lacour, Chimia, 2006, 60, 266 CrossRef CAS;
(f) T. Ooi and K. Maruoka, Angew. Chem., Int. Ed., 2007, 46, 4222 CrossRef CAS PubMed;
(g) T. Ooi and K. Maruoka, Aldrichimica Acta, 2007, 40, 77 CAS;
(h) T. Hashimoto and K. Maruoka, Chem. Rev., 2007, 107, 5656 CrossRef CAS PubMed;
(i) K. Maruoka, Org. Process Res. Dev., 2008, 12, 679 CrossRef CAS;
(j) S.-S. Jew and H.-G. Park, Chem. Commun., 2009, 7090 RSC;
(k) K. Maruoka, Chem. Rec., 2010, 10, 254 CrossRef CAS PubMed;
(l) S. Shirakawa and K. Maruoka, Angew. Chem., Int. Ed., 2013, 52, 4312 CrossRef CAS PubMed.
- For reviews on asymmetric desymmetrization, see:
(a) M. C. Willis, J. Chem. Soc., Perkin Trans. 1, 1999, 1765 RSC;
(b) E. García-Urdiales, I. Alfonso and V. Gotor, Chem. Rev., 2005, 105, 313 CrossRef PubMed;
(c)
T. Rovis, in New Frontiers in Asymmetric Catalysis, ed. K. Mikami and M. Lautens, John Wiley & Sons, Hoboken, New Jersey, 2007, p. 275 Search PubMed.
-
(a) S. Arai, S. Hamaguchi and T. Shioiri, Tetrahedron Lett., 1998, 39, 2997 CrossRef CAS;
(b) R. Tello-Aburto, K. A. Kalstabakken, K. A. Volp and A. M. Harned, Org. Biomol. Chem., 2011, 9, 7849 RSC; See also:
(c) A. Claraz, S. Oudeyer and V. Levacher, Tetrahedron: Asymmetry, 2013, 24, 764 CrossRef CAS PubMed;
(d) S. Shirakawa, X. Wu and K. Maruoka, Angew. Chem., Int. Ed., 2013, 52, 14200 CrossRef CAS PubMed;
(e) R. J. Armstrong and M. D. Smith, Angew. Chem., Int. Ed., 2014, 53, 12822 CrossRef CAS PubMed.
- For a review on chiral bifunctional quaternary ammonium salts, see: J. Novacek and M. Waser, Eur. J. Org. Chem., 2013, 637 CrossRef CAS.
- G. Dickmeiss, V. De Sio, J. Udmark, T. B. Poulsen, V. Marcos and K. A. Jørgensen, Angew. Chem., Int. Ed., 2009, 48, 6650 CrossRef CAS PubMed.
-
(a) M. Kitamura, S. Shirakawa and K. Maruoka, Angew. Chem., Int. Ed., 2005, 44, 1549 CrossRef CAS PubMed;
(b) M. Kitamura, S. Shirakawa, Y. Arimura, X. Wang and K. Maruoka, Chem. – Asian J., 2008, 3, 1702 CrossRef CAS PubMed.
-
(a) R. He, S. Shirakawa and K. Maruoka, J. Am. Chem. Soc., 2009, 131, 16620 CrossRef CAS PubMed;
(b) X. Wang, Q. Lan, S. Shirakawa and K. Maruoka, Chem. Commun., 2010, 46, 321 RSC;
(c) S. Shirakawa, L. Wang, R. He, S. Arimitsu and K. Maruoka, Chem. – Asian J., 2014, 9, 1586 CrossRef CAS PubMed.
-
(a) L. Wang, S. Shirakawa and K. Maruoka, Angew. Chem., Int. Ed., 2011, 50, 5327 CrossRef PubMed;
(b) S. Shirakawa, S. J. Terao, R. He and K. Maruoka, Chem. Commun., 2011, 47, 10557 RSC.
-
(a) S. Shirakawa, A. Kasai, T. Tokuda and K. Maruoka, Chem. Sci., 2013, 4, 2248 RSC;
(b) S. Shirakawa, T. Tokuda, A. Kasai and K. Maruoka, Org. Lett., 2013, 15, 3350 CrossRef CAS PubMed;
(c) S. Shirakawa, K. Koga, T. Tokuda, K. Yamamoto and K. Maruoka, Angew. Chem., Int. Ed., 2014, 53, 6220 CrossRef CAS PubMed.
- The reaction of 1a in the absence of a phase-transfer catalyst gave product 2a in very low yield (<5%).
- The reaction with methyl-substituted epoxy ketone 1 (Ar = Me) gave the corresponding product 2 in low enantioselectivity (91%, 4% ee).
- For examples of asymmetric isomerization of cyclic carbonyl compounds, see:
(a) Y. Wu, R. P. Singh and L. Deng, J. Am. Chem. Soc., 2011, 133, 12458 CrossRef CAS PubMed;
(b) J. H. Lee and L. Deng, J. Am. Chem. Soc., 2012, 134, 18209 CrossRef CAS PubMed;
(c) X.-S. Xue, X. Li, A. Yu, C. Yang and J.-P. Cheng, J. Am. Chem. Soc., 2013, 135, 7462 CrossRef CAS PubMed.
Footnotes |
† This paper is dedicated to Professor Ei-ichi Negishi on his 80th Birthday. |
‡ Electronic supplementary information (ESI) available: Experimental details and characterization data for new compounds. See DOI: 10.1039/c4qo00339j |
|
This journal is © the Partner Organisations 2015 |
Click here to see how this site uses Cookies. View our privacy policy here.