DOI:
10.1039/C4QO00253A
(Research Article)
Org. Chem. Front., 2014,
1, 1261-1265
Efficient and scalable Pd-catalyzed double aminocarbonylations under atmospheric pressure at low catalyst loadings†‡
Received
23rd September 2014
, Accepted 21st October 2014
First published on 22nd October 2014
Abstract
By using a robust acenaphthoimidazolylidene palladium complex (Pd-NHC 1), a scalable approach to access a variety of chiral, pharmaceutical and structurally intriguing N-substituted phthalimides via double aminocarbonylations has been established under atmospheric carbon monoxide pressure at catalyst loadings as low as 0.05 mol%. In addition, the fluorescent properties of the selected N-substituted phthalimide products were also characterized. In comparison with well-known fluorescent molecules, some of them exhibited enhanced violet emission, especially for the ester analogue of Alrestatin, which further confirmed the applicability of the protocol.
Introduction
As one of the useful motifs, imides widely exist in various bioactive, fluorescent and pharmaceutical molecules.1 Besides pyromellitic diimides applied as high-performance polymeric materials for aerospacecrafts,2 phthalimides and their derivatives also exhibit extensive applicability in drugs, pesticides and dyes.3–5 Therefore, various efforts have been devoted to the syntheses of cyclic imides.6 In contrast with conventional methodologies, including condensation of a phthalic acid anhydride and a primary amine, which show rather limited substrate applicability even at high temperature, transition-metal catalyzed aminocarbonylations of haloarenes with inexpensive carbon monoxide (CO gas) represent an atom-economic, efficient and straightforward protocol.7 Inspired by the seminal work of Heck in 1974, Ban and co-workers realized a nice example of Pd-catalyzed mono-aminocarbonylations.8 A decade later, Perry and co-workers extended it to the double aminocarbonylations under very high CO pressure.9 After developing for decades, there are still various limitations in this less-studied topic. In general, a high amount of catalyst containing air sensitive phosphine ligands is usually required to achieve satisfactory outcomes.10 Besides high CO pressure (up to 39 atm), sterically demanding and heterocyclic substrates are still not well tolerated in the known protocols.10
As robust ligands, N-heterocyclic carbenes (NHCs) have been successfully applied in numerous Pd-catalyzed cross-coupling reactions.11 Furthermore, unlike phosphine ligands, NHCs behave as strong σ-donors almost without metal-to-ligand π-back-bonding ability, which can significantly increase the electron density on the metal center and constitute optional ligands for the carbonylation reactions.12 However, to the best of our knowledge, there is still no example of the synthesis of N-substituted phthalimides by using Pd-NHC catalysts. Moreover, in comparison with imidazole analogues, we found that the less-explored ylidenes derived from acenaphthoimidazolium salts exhibited stronger σ-donor and weaker π-acceptor properties, and the corresponding Pd-NHCs (1–3, Scheme 1) revealed extremely high catalytic activity and broad substrate scope in several Pd-catalyzed cross-coupling reactions as well as aminocarbonylative reactions at extremely low catalyst loadings.13 Encouraged by these promising results and our recent achievements in exploring a series of metal-complexes in the soft materials and catalysis,13–15 herein, we would like to extend the feasibility of these bulky Pd-NHCs (1–3) to fabricate a variety of structurally intriguing functional N-substituted phthalimides under mild reaction conditions, especially, at low catalyst loadings.
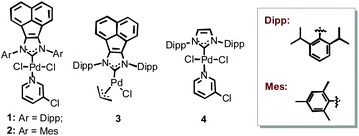 |
| Scheme 1 Representative Pd-NHCs complexes. | |
Results and discussion
Initially, o-diiodobenzene and aniline were selected to explore the catalytic activity of Pd-NHCs 1–4 in the double aminocarbonylative reactions under atmospheric CO pressure. After detailed optimization of the reaction conditions (see ESI‡), a quantitative yield of N-phenylphthalimide 5 was obtained when the reaction was carried out with 1 mol% Pd-NHC 1 and 1.5 equiv. 1,4-diazabicyclo[2.2.2]octane (DABCO) as a base in o-xylene at 110 °C for 30 hours (entry 1, Table 1). To our delight, a full conversion was still observed within 24 hours when the catalyst loading was reduced to 0.5 mol% (entries 2–3, Table 1). When other Pd-NHCs (2–4) were involved, all resulted in good to excellent outcomes (91–>99%, entries 4–6, Table 1). Under the optimal reaction conditions, Pd(OAc)2 and PPh3, however, only gave a 47% isolated yield (entry 7, Table 1), which indicated ligands with strong π-acceptor properties were unfavourable for the transformation. When the catalyst loading of Pd-NHC 1 was reduced to 0.05 mol%, a moderate yield was still observed within 30 hours, which can be further increased to a quantitative yield by extending the reaction time to 48 hours (entry 8, Table 1). Further decreasing the catalyst loading to 0.01 mol%, a 50% yield was still observed (TON: 5000, entry 10, Table 1), which was obviously superior to the result of Pd-NHC 4 (entry 9, Table 1) and further confirmed that ylidenes derived from the π-extended imidazolium salts are better ligands than that from their imidazolium analogues.14 In addition, no desired product was found in the blank test (entry 11, Table 1).
Table 1 Reaction conditions optimizationa
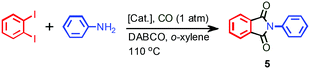
|
Entry |
[Cat.] |
mol% |
Time (h) |
Yieldb (%) |
0.5 mmol scale.
Isolated yield.
|
1 |
1
|
1 |
30 |
>99 |
2 |
1
|
0.5 |
30 |
>99 |
3 |
1
|
0.5 |
24 |
>99 |
4 |
2
|
0.5 |
24 |
91 |
5 |
3
|
0.5 |
24 |
>99 |
6 |
4
|
0.5 |
24 |
96 |
7 |
Pd(OAc)2/PPh3 |
0.5 |
24 |
47 |
8 |
1
|
0.05 |
30/48 |
71/>99 |
9 |
4
|
0.05 |
48 |
6 |
10 |
1
|
0.01 |
48 |
50 |
11 |
— |
— |
24 |
N.D. |
With the optimal reaction conditions in hand, 0.5 mol% catalyst and 24 hours were selected to further evaluate the substrate scope. As shown in Table 2, our protocol well tolerated various primary amines with diverse electronic and steric properties. The relative position of substituents on anilines slightly influenced the process: p-toluidine resulted in a higher yield than its o- and m-analogues (6cvs.6a and 6b). Anilines with electron-donating groups were well tolerated (7a–b, up to 95%). As an efficient anticonvulsant drug without neurotoxicity and mortality,16 to our delight, compound 7a was readily prepared in gram scale at such low catalyst loading (1.14 g, 90%). Anilines containing electron-withdrawing groups (–CF3 and –F) also resulted in up to quantitative yields (8a–b). The halogen atoms such as Cl and Br were well accommodated (9a–b), which provided possibility for further functionalization. Although the previous reports were all inefficient for sterically demanding anilines,10 satisfactory isolated yields (10–13, 76–88%) were achieved by our protocol in all selected cases, which further confirmed our protocol efficiency. Among them, compound 11 (PP-33), approved as an α-tumor necrosis factor (TNF) inhibitor, was also synthesized in a good yield.17 In consideration of the satisfactory results obtained so far, we turned our attention to primary alkylamines. Besides benzyl amine, a chiral amine also resulted in an excellent yield without affecting the chiral center (15, 90%). Other aliphatic amines were also suitable substrates, up to 95% yields were obtained with linear, cyclic, and heterocyclic substituted amines (16–19), and slightly inferior outcomes were observed with low-boiling aliphatic amines (16 and 18). When morpholin-4-amine was involved, a moderate yield was obtained (20, 68%). N-Heterocyclic anilines were usually regarded as worse partners in the transition-metal catalyzed coupling reactions due to their strong coordination ability,10c however, our protocol well tolerated pyridin-3-amine and bulky quinolin-3-amine (21–22), and even up to quantitative yields were observed at 0.5 mol% catalyst loading. In addition, our approach was also suitable for an alkyl diamine and produced di-N-phenylphthalimide 23 in a good yield (70%).
Table 2 Pd-Catalyzed double aminocarbonylation of o-diiodobenzene with various primary aminesa
0.5 mmol scale for 24 h.
Isolated yield.
With 0.05 mol% Pd-NHC 1.
With 0.5 mmol 1,6-diaminohexane, 1.25 mmol diiodobenzene, 3.0 mmol DABCO and 1 mol% Pd-NHC 1.
|
|
Subsequently, our attention was focused on the feasibility of other o-dihaloarenes. As expected, in the presence of 0.5 mol% Pd-NHC 1, the aminocarbonylation of selected o-diiodoarenes with aniline and aliphatic amines all produced corresponding products in good to quantitative yields under the optimized conditions (24–31, Table 3), whereas, a lower yield was encountered with o-bromoiodobenzene (5, Table 3). When bulkier 1,8-diiodonaphthalene was applied, diverse 1,8-naphthalimides were easily accessible in good to quantitative yields (28–31). It has to be noted that compound 30 was readily completely hydrolyzed in aqueous sodium hydroxide to give a well-known aldose reductase inhibitor (Alrestatin, 32, see ESI‡) in a quantitative yield.18 Moreover, in consideration of strongly fluorescent compound 29 having potential applications in molecular sensors,19 several analogues produced by our protocol (27, 28, 30, and 31) were selected to study their fluorescent properties (Fig. 1). In comparison with compound 29, a slight red shift was observed with all tested samples (1 × 10−5 mol L−1, in CH2Cl2). Compounds 27 and 30 exhibited significant enhanced violet emission. However, the intensity of compounds 28 and 31 at the same concentration were slightly low, which may be caused by (hetero)-aryl substituent groups partially quenching the emission. These results not only indicated our protocol was practical to access various fluorescent molecules, but also highlighted another potential functionality of Alrestatin.
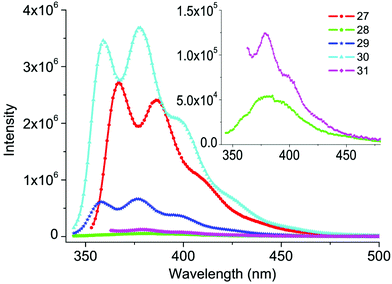 |
| Fig. 1 The fluorescence spectra of compounds 27–31 (measured in CH2Cl2 at 1 × 10−5 mol L−1 at room temperature, excitation wavelength at 334 nm for compounds 27–30 and 346 nm for compound 31). | |
Table 3 Pd-Catalyzed double aminocarbonylation of o-dihaloarenesa
0.5 mmol scale for 24 h.
Isolated yield.
In case of o-bromoiodobenzene.
Glycine methyl ester hydrochloride was used as amine source.
|
|
In light of the high efficiency of our protocol to access a variety of pharmaceutical and fluorescent molecules, which are hard to access by the conventional protocol, we paid attention to the synthesis of well-known thalidomide. Currently, thalidomide has exhibited therapeutic value in the treatment of myeloma and leprosy.20 By using 3-amino-piperidine-2,6-dione hydrochloride instead of the sensitive amine, in the presence of 0.5 mol% Pd-NHC 1, double aminocarbonylation of o-diiodobenzene also successfully produced the desired thalidomide in a good isolated yield (81%, Scheme 2), which further confirmed the broad and practical feasibility of the new developed methodology.
 |
| Scheme 2 Synthesis of thalidomide. | |
Conclusion
In summary, by using a robust acenaphthoimidazolylidene palladium complex (Pd-NHC 1), we have successfully developed a mild, practical and scalable protocol to access a variety of functional and structurally intriguing N-substituted phthalimides via palladium-catalyzed double aminocarbonylation of o-dihaloarenes with diverse primary amines under atmospheric carbon monoxide pressure at catalyst loadings as low as 0.05 mol%. In comparison with previous reports with phosphine ligands, diverse electron-rich, electron-poor and heterocyclic substrates are easily converted to corresponding products even for di-phthalimides in good to excellent yields under the mild reaction conditions at such low catalyst loadings. Meanwhile, sterically hindered (heterocyclic) amines are also applicable under the optimized reaction conditions, which constitute a challenging task for aminocarbonylative reactions and also are hard to access by using the conventional approaches. Notably, several important chiral, pharmaceutical and fluorescent molecules such as thalidomide and Alrestatin are also accessible by our newly developed approach even in gram scale. Additionally, the fluorescent properties of the selected N-substituted phthalimide products were also characterized; among them the enhanced fluorescence of compound 30 which also demonstrated another application of Alrestatin besides pharmaceutical utilization.
Acknowledgements
Financial support from the National Natural Science Foundation of China (no. 21172045 and 91127041), the Changjiang Scholars and Innovative Research Team in University (IRT1117), the doctoral fund of Ministry of Education of China (20130071110032), Shanghai Shuguang and Pujiang Programs and Department of Chemistry, Fudan University is gratefully acknowledged.
Notes and references
-
(a) R. Antunes, H. Batista, R. M. Srivastava, G. Thomas and C. C. Araujo, Bioorg. Med. Chem. Lett., 1998, 8, 3071 CrossRef CAS
;
(b) L. M. Lima, P. Castro, A. L. Machado, C. A. M. Fraga, C. Lugnier, V. L. G. de Moraes and E. J. Barreiro, Bioorg. Med. Chem., 2002, 10, 3067 CrossRef CAS
;
(c) U. Sharma, P. Kumar, N. Kumar and B. Singh, Mini-Rev. Med. Chem., 2010, 10, 678 CrossRef CAS
;
(d) M. A. Bhat and M. A. Al-Omar, Acta Pol. Pharm. Drug Res., 2011, 68, 375 CAS
.
-
(a) K. H. Chae and Y. H. Kim, Adv. Funct. Mater., 2007, 17, 3470 CrossRef CAS
;
(b) G. Chen, X. Zhang, S. Zhang, T. Chen and Y. Wu, J. Appl. Polym. Sci., 2007, 106, 2808 CrossRef CAS
.
-
(a) E. de Almeida Neto, Hansenol. Int., 1981, 6, 114 CAS
;
(b) M. Walchner, M. Meurer, G. Plewig and G. Messer, Int. J. Dermatol., 2000, 39, 383 CrossRef CAS
.
- W. D. Figg, S. Raje, K. S. Bauer, A. Tompkins, D. Venzon, R. Bergan, A. Chen, M. Hamilton, J. Pluda and E. Reed, J. Pharm. Sci., 1999, 88, 121 CrossRef CAS PubMed
.
-
(a) N. S. Pawar, U. R. Kapadi, D. G. Hundiwale and P. P. Kumbhar, J. Sci. Ind. Res., 2002, 61, 454 CAS
;
(b) S. P. Gromov, M. V. Fomina, A. S. Nikiforov, A. I. Vedernikov, L. G. Kuz'mina and J. A. K. Howard, Tetrahedron, 2013, 69, 5898 CrossRef CAS PubMed
.
-
(a) J. C. Sheehan and V. S. Frank, J. Am. Chem. Soc., 1949, 71, 1856 CrossRef CAS
;
(b) J. C. Sheehan, D. W. Chapman and R. W. Roth, J. Am. Chem. Soc., 1952, 74, 3822 CrossRef CAS
;
(c) T. Han and C.-F. Chen, J. Org. Chem., 2007, 72, 7287 CrossRef CAS PubMed
;
(d) M. Sani, G. Fossati, F. Huguenot and M. Zanda, Angew. Chem., Int. Ed., 2007, 46, 3526 CrossRef CAS PubMed
;
(e) J. Zhang, M. Senthilkumar, S. C. Ghosh and S. H. Hong, Angew. Chem., Int. Ed., 2010, 49, 6391 CrossRef CAS PubMed
.
-
(a) R. Skoda-Foldes and L. Kollar, Curr. Org. Chem., 2002, 6, 1097 CrossRef CAS
;
(b) A. Brennführer, H. Neumann and M. Beller, Angew. Chem., Int. Ed., 2009, 48, 4114 CrossRef PubMed
;
(c) W. Fang, H. Zhu, Q. Deng, S. Liu, X. Liu, Y. Shen and T. Tu, Synthesis, 2014, 46, 1689 CrossRef PubMed
;
(d) S. T. Gadge and B. M. Bhanage, RSC Adv., 2014, 4, 10367 RSC
and references therein.
-
(a) A. Schoennberg and R. F. Heck, J. Org. Chem., 1974, 39, 3327 CrossRef
;
(b) M. Mori, K. Chiba, N. Ohta and Y. Ban, Heterocycles, 1979, 13, 329 CrossRef CAS
.
- R. J. Perry and S. R. Turner, J. Org. Chem., 1991, 56, 6573 CrossRef CAS
.
-
(a) A. Takács, P. Ács and L. Kollár, Tetrahedron, 2008, 64, 983 CrossRef PubMed
;
(b) S. Inoue, H. Shiota, Y. Fukumoto and N. Chatani, J. Am. Chem. Soc., 2009, 131, 6898 CrossRef CAS PubMed
;
(c) H. Cao and H. Alper, Org. Lett., 2010, 12, 4126 CrossRef CAS PubMed
;
(d) M. V. Khedkar, S. R. Khan, D. N. Sawant, D. B. Bagal and B. M. Bhanage, Adv. Synth. Catal., 2011, 353, 3415 CrossRef CAS
;
(e) D. Marosvölgyi-Haskó, A. Petz, A. Takács and L. Kollár, Tetrahedron, 2011, 67, 9122 CrossRef PubMed
;
(f) X.-F. Wu, S. Oschatz, M. Sharif, A. Flader, L. Krey, M. Beller and P. Langer, Adv. Synth. Catal., 2013, 355, 3581 CrossRef CAS
;
(g) J. Chen, K. Natte, A. Spannenberg, H. Neumann, M. Beller and X.-F. Wu, Org. Biomol. Chem., 2014, 12, 5578 RSC
.
- N. Marion and S. P. Nolan, Acc. Chem. Res., 2008, 41, 1440 CrossRef CAS PubMed
.
-
(a) S. Würtz and F. Glorius, Acc. Chem. Res., 2008, 41, 1523 CrossRef PubMed
;
(b) S. Díez-González, N. Marion and S. P. Nolan, Chem. Rev., 2009, 109, 3612 CrossRef PubMed
.
- W. Fang, Q. Deng, M. Xu and T. Tu, Org. Lett., 2013, 15, 3678 CrossRef CAS PubMed
.
-
(a) T. Tu, W. Assenmacher, H. Peterlik, R. Weisbarth, M. Nieger and K. H. Dötz, Angew. Chem., Int. Ed., 2007, 46, 6368 CrossRef CAS PubMed
;
(b) T. Tu, W. Assenmacher, H. Peterlik, G. Schnakenburg and K. H. Dötz, Angew. Chem., Int. Ed., 2008, 47, 7127 CrossRef CAS PubMed
;
(c) T. Tu, X. Bao, W. Assenmacher, H. Peterlik, J. Daniels and K. H. Dötz, Chem. – Eur. J., 2009, 15, 1853 CrossRef CAS PubMed
;
(d) T. Tu, W. Fang, X. Bao, X. Li and K. H. Dötz, Angew. Chem., Int. Ed., 2011, 50, 6601 CrossRef CAS PubMed
;
(e) W. Fang, Z. Sun and T. Tu, J. Phys. Chem. C, 2013, 117, 25185 CrossRef CAS
;
(f) W. Fang, X. Liu, Z. Lu and T. Tu, Chem. Commun., 2014, 50, 3313 RSC
.
-
(a) T. Tu, H. Mao, C. Herbert, M. Xu and K. H. Dötz, Chem. Commun., 2010, 46, 7796 RSC
;
(b) T. Tu, X. Feng, Z. Wang and X. Liu, Dalton Trans., 2010, 39, 10598 RSC
;
(c) Z. Wang, X. Feng, W. Fang and T. Tu, Synlett, 2011, 951 CAS
;
(d) T. Tu, W. Fang and J. Jiang, Chem. Commun., 2011, 47, 12358 RSC
;
(e) T. Tu, Z. Sun, W. Fang, M. Xu and Y. Zhou, Org. Lett., 2012, 14, 4250 CrossRef CAS PubMed
;
(f) W. Fang, J. Jiang, Y. Xu, J. Zhou and T. Tu, Tetrahedron, 2013, 69, 673 CrossRef CAS PubMed
;
(g) Z. Liu, N. Dong, M. Xu, Z. Sun and T. Tu, J. Org. Chem., 2013, 78, 7436 CrossRef CAS PubMed
;
(h) M. Xu, X. Li, Z. Sun and T. Tu, Chem. Commun., 2013, 49, 11539 RSC
.
- A. A.-M. AbdeI-Hafez, Arch. Pharmacal Res., 2004, 27, 495 CrossRef
.
- H. Miyachi, A. Azuma, E. Hioki, S. Iwasaki, Y. Kobayashi and Y. Hashimoto, Biochem. Biophys. Res. Commun., 1996, 224, 426 CrossRef CAS PubMed
.
- A. DaSettimo, G. Primofiore, F. Da Settimo, F. Simorini, C. La Motta, A. Martinelli and E. Boldrini, Eur. J. Med. Chem., 1996, 31, 49 CrossRef CAS
.
- S. Erten-Ela, S. Ozcelik and E. Eren, J. Fluoresc., 2011, 21, 1565 CrossRef CAS PubMed
.
- S. M. Capitosti, T. P. Hansen and M. L. Brown, Org. Lett., 2003, 5, 2865 CrossRef CAS PubMed
.
Footnotes |
† Dedicated to Prof. Li-Xin Dai on the occasion of his 90th birthday. |
‡ Electronic supplementary information (ESI) available: Experimental details and mechanism study. See DOI: 10.1039/c4qo00253a |
|
This journal is © the Partner Organisations 2014 |
Click here to see how this site uses Cookies. View our privacy policy here.