DOI:
10.1039/C4QO00072B
(Research Article)
Org. Chem. Front., 2014,
1, 351-354
Singlet carbenes as mimics for transition metals: synthesis of an air stable organic mixed valence compound [M2(C2)+˙; M = cyclic(alkyl)(amino)carbene]†
Received
13th March 2014
, Accepted 24th March 2014
First published on 25th March 2014
Abstract
A cyclic C-bromo-iminium bromide reacts with lithium trimethylsilyl acetylide to afford the title compound 2+˙ in 40% yield. In contrast to other organic mixed valence compounds, this radical cation can be stored in air. Oxidation and reduction of 2+˙ afford the corresponding dication 2++ and neutral cumulene 2, respectively, in good yields.
During the last few years, it has been shown that due to the presence of a lone pair and an accessible vacant orbital, singlet carbenes could mimic the chemical behaviour of transition metal centers.1 Indeed, stable and rather electrophilic carbenes such as cyclic(alkyl)(amino)carbenes (CAACs)2 and diamidocarbenes3 can activate small molecules (H2, CO and NH3),4 and enthalpically strong bonds such as Si–H, P–H, B–H, P–P, and even C–H bonds.5 Moreover, it has been found that these carbenes can stabilize a variety of main-group compounds featuring unusual bonding situations,6 as well as paramagnetic species.7,8 Since the pioneering work by Creutz and Taube,9 bimetallic mixed-valence systems have attracted much attention due to their potential applications in electronic conductors and non-linear optical materials, among others.10 Although organic mixed-valence compounds have been known for decades,11 their applications have been hampered due to their sensitivity to oxygen and their lack of thermal stability.12 Here we report that the peculiar electronic properties of CAACs13 allow for the preparation of a thermally and air stable purely organic mixed-valence derivative.14
Cyclic C-bromo-iminium bromide 1 readily reacts at −78 °C with a freshly prepared THF solution of lithium trimethylsilyl acetylide in THF (Scheme 1). Within a few minutes at room temperature the pale yellow suspension darkens to a deep red colour. After removal of all volatiles and washing of the crude mixture with diethyl ether, compound 2+˙ was extracted with dichloromethane and recovered as a red solid (40% yield).
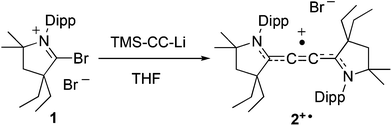 |
| Scheme 1 Synthesis of radical cation 2+˙ (Dipp: 2,6-diisopropylphenyl). | |
The paramagnetic nature of 2+˙ was clearly demonstrated by the X-band EPR spectrum shown in Fig. 1 (bottom left). Red single crystals were obtained by slow diffusion of diethyl ether into a THF solution of 2+˙ in air, and were subjected to an X-ray diffraction study. Compound 2+˙ crystallizes in a monoclinic P21/n space group with an inversion center in the middle of the central CC bond. The 5-membered rings are coplanar in a trans configuration. The NC1 [1.338(3) Å], C1C2 [1.376(3) Å] and C2C2′ [1.230(5) Å] bond distances are in agreement with the presence of a delocalized unpaired electron across the NC1C2C2′C1′N′ fragment (Table 1). This delocalization is substantiated by DFT calculations [B3LYP/6-311 g(d,p)], the spin density being distributed over the N (44%), C1 (24%) and C2 (31%) centres (Fig. 1, top right inset). Very interestingly, the radical cation 2+˙ is thermally very stable [m.p. > 250 °C(dec.)], and even moisture and air stable for several months in the solid state.
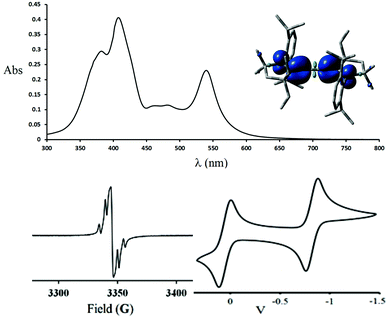 |
| Fig. 1 UV-Vis spectrum (5.5 × 10−4 mol L−1 in methylene chloride) (top), spin density map [hydrogen atoms omitted for clarity, B3LYP/6-311 g(d,p)] (top right inset), X-Band EPR spectrum (bottom left), and cyclic voltammogram (nBu4NPF6 0.1 M, 100 mV S−1, versus Fc/Fc+) (bottom right) of 2+˙. | |
Table 1 Solid-state structure and selected bond lengths (Å) and angles (°) of compounds 2++, 2+˙, and 2 (H atoms and anions are omitted for clarity)
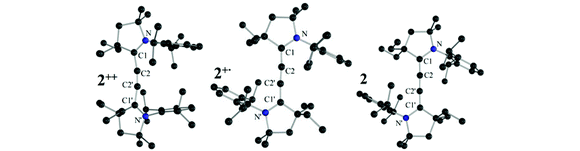 |
|
NC1 |
C1C2 |
C2C2′ |
NC1C2 |
C1C2C1′ |
NC1C1′N′ |
2++ |
1.289(2) |
1.438(2) |
1.195(4) |
123.63(16) |
171.04(11) |
45.59 |
2+˙ |
1.338(3) |
1.376(3) |
1.230(5) |
123.74(19) |
175.5(3) |
180 |
2 |
1.3904(15) |
1.3332(17) |
1.266(2) |
124.45(11) |
177.73(16) |
180 |
The cyclic voltammogram of 2+˙ shows two reversible redox processes at E1/2 = +0.05 V and −0.82 V (versus Fc+/Fc) (Fig. 1, bottom right). The large coupling of about 0.87 V is another indication of the delocalization of the unpaired electron in the extended π-system.
The UV-Vis spectrum of a methylene chloride solution of the radical cation 2+˙ shows strong absorption bands at 382, 408 and 540 nm, along with weak bands at 464 and 482 nm (Fig. 1, top).
Encouraged by these results, we engaged in the synthesis of the oxidized and reduced derivatives of 2+˙, namely the dication 2++ and cumulene 2 (Scheme 2). An excess of bromine was added at room temperature to a chloroform solution of the radical cation 2+˙. An immediate yellow precipitate appeared, and after filtration and washing with diethyl ether, 2++ was isolated as a pale yellow, air stable, solid in 53% yield. Similarly to radical cation 2+˙, the dication 2++ proved to be highly thermally stable [m.p. = 200 °C (dec.)]. The diamagnetic nature of 2++ allows for its characterization by NMR spectroscopy. In the 13C NMR spectrum, the four quaternary carbons C1, C1′, and C2, C2′ give signals at +178.8 and +96.7 ppm, respectively, in the usual range of iminium and alkyne carbons.
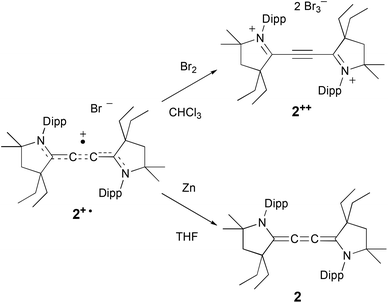 |
| Scheme 2 Oxidation and reduction of radical cation 2+˙. | |
Treatment of a THF solution of 2+˙ with an excess of zinc powder, in the absence of air, led to a rapid fading of the red colour. The solvent was removed under vacuum, and the residue was extracted with diethyl ether. After evaporation of the solvent, 2 was isolated as a yellow solid in 56% yield. Although 2 is highly air sensitive, it is stable under an inert atmosphere for weeks both in the solid state and in solution. The cumulenic carbons give signals at δ = 140.8 and 127.9 ppm, in the range observed for other cumulenes.15
Single crystals of 2++ and 2 were subjected to an X-ray diffraction study, which allows comparing the bond lengths along the series 2++, 2+˙, and 2 (Table 1). As expected, addition of one and two electrons into the extended π-system results in lengthening of the N–C1 and C2–C2′ bonds, with a concomitant shortening of the C1–C2 bond. Also noteworthy is the coplanarity of the two five-membered rings in 2+˙ and 2, whereas they are twisted by 46° in 2++.
Compounds 2++, 2+˙, and 2 can be viewed as a C2++, C2+˙ and C2 fragment, respectively, stabilized by two CAACs.16 The straightforward synthesis of 2+˙, and its surprising air stability, pave the way for the preparation of a variety of organic mixed valence systems featuring electrophilic carbenes as stabilizing groups for different radical cation spacers.
Thanks are due to the National Science Foundation (CHE-1316956), and to the China Scholarship Council for a graduate fellowship (L.L.).
Notes and references
- D. Martin, M. Soleilhavoup and G. Bertrand, Chem. Sci., 2011, 2, 389–399 RSC.
-
(a) V. Lavallo, Y. Canac, C. Prasang, B. Donnadieu and G. Bertrand, Angew. Chem., Int. Ed., 2005, 44, 5705–5709 CrossRef CAS PubMed;
(b) R. Jazzar, R. D. Dewhurst, J. B. Bourg, B. Donnadieu, Y. Canac and G. Bertrand, Angew. Chem., Int. Ed., 2007, 46, 2899–2902 CrossRef CAS PubMed;
(c) R. Jazzar, J. B. Bourg, R. D. Dewhurst, B. Donnadieu and G. Bertrand, J. Org. Chem., 2007, 72, 3492–3499 CrossRef CAS PubMed.
-
(a) T. W. Hudnall and C. W. Bielawski, J. Am. Chem. Soc., 2009, 131, 16039–16041 CrossRef CAS PubMed;
(b) T. W. Hudnall, A. G. Tennyson and C. W. Bielawski, Organometallics, 2010, 29, 4569–4578 CrossRef CAS;
(c) G. A. Blake, J. P. Moerdyk and C. W. Bielawski, Organometallics, 2012, 31, 3373–3378 CrossRef CAS.
-
(a) V. Lavallo, Y. Canac, B. Donnadieu, W. W. Schoeller and G. Bertrand, Angew. Chem., Int. Ed., 2006, 45, 3488–3491 CrossRef CAS PubMed;
(b) G. D. Frey, V. Lavallo, B. Donnadieu, W. W. Schoeller and G. Bertrand, Science, 2007, 316, 439–441 CrossRef CAS PubMed;
(c) T. W. Hudnall, J. P. Moerdyk and C. W. Bielawski, Chem. Commun., 2010, 46, 4288–4290 RSC;
(d) J. P. Moerdyk, G. A. Blake, D. T. Chase and C. W. Bielawski, J. Am. Chem. Soc., 2013, 135, 18798–18801 CrossRef CAS PubMed;
(e) K. M. Wiggins, J. P. Moerdyk and C. W. Bielawski, Chem. Sci., 2012, 3, 2986–2992 RSC.
-
(a) J. D. Masuda, W. W. Schoeller, B. Donnadieu and G. Bertrand, Angew. Chem., Int. Ed., 2007, 46, 7052–7055 CrossRef CAS PubMed;
(b) O. Back, G. Kuchenbeiser, B. Donnadieu and G. Bertrand, Angew. Chem., Int. Ed., 2009, 48, 5530–5533 CrossRef CAS PubMed;
(c) G. D. Frey, J. D. Masuda, B. Donnadieu and G. Bertrand, Angew. Chem., Int. Ed., 2010, 49, 9444–9447 CrossRef CAS PubMed;
(d) J. P. Moerdyk and C. W. Bielawski, Nat. Chem., 2012, 4, 275–280 CrossRef CAS PubMed;
(e) J. P. Moerdyk and C. W. Bielawski, Chem. – Eur. J., 2013, 19, 14773–14776 CrossRef CAS PubMed;
(f) D. T. Chase, J. P. Moerdyk and C. W. Bielawski, Org. Lett., 2014, 16, 812–815 CrossRef CAS PubMed.
-
(a) Y. Wang, Y. Xie, P. Wei, R. B. King, H. F. Schaefer III, P. V. R. Schleyer and G. H. Robinson, Science, 2008, 321, 1069–1071 CrossRef CAS PubMed;
(b) C. A. Dyker and G. Bertrand, Science, 2008, 321, 1050–1051 CrossRef CAS PubMed;
(c) A. Sidiropoulos, C. Jones, A. Stasch, S. Klein and G. Frenking, Angew. Chem., Int. Ed., 2009, 48, 9701–9704 CrossRef CAS PubMed;
(d) M. Y. Abraham, Y. Wang, Y. Xie, R. J. Gilliard Jr., P. Wei, H. F. Schaefer, P. V. R. Schleyer III and G. H. Robinson, Chem. – Eur. J., 2010, 16, 432–435 CrossRef CAS PubMed;
(e) C. Jones, A. Sidiropoulos, N. Holzmann, G. Frenking and A. Stasch, Chem. Commun., 2012, 48, 9855–9857 RSC;
(f) H. Braunschweig, R. D. Dewhurst, K. Hammond, J. Mies, K. Radacki and A. Vargas, Science, 2012, 336, 1420–1422 CrossRef CAS PubMed.
- For a review, see: C. D. Martin, M. Soleilhavoup and G. Bertrand, Chem. Sci., 2013, 4, 3020–3030 RSC.
-
(a) O. Back, M. A. Celik, G. Frenking, M. Melaimi, B. Donnadieu and G. Bertrand, J. Am. Chem. Soc., 2010, 132, 10262–10263 CrossRef CAS PubMed;
(b) R. Kinjo, B. Donnadieu, M. Ali Celik, G. Frenking and G. Bertrand, Science, 2011, 333, 610–613 CrossRef CAS PubMed;
(c) K. C. Mondal, H. W. Roesky, M. C. Schwarzer, G. Frenking, I. Tkach, H. Wolf, D. Kratzert, R. Herbst-Irmer, B. Niepötter and D. Stalke, Angew. Chem. Int. Ed., 2013, 52, 1801–1805 CrossRef CAS PubMed;
(d) K. C. Mondal, H. W. Roesky, M. C. Schwarzer, G. Frenking, B. Niepötter, H. Wolf, R. Herbst-Irmer and D. Stalke, Angew. Chem., Int. Ed., 2013, 52, 2963–2967 CrossRef CAS PubMed;
(e) D. S. Weinberger, M. Melaimi, C. E. Moore, A. L. Rheingold, G. Frenking, P. Jerabek and G. Bertrand, Angew. Chem., Int. Ed., 2013, 52, 8964–8967 CrossRef CAS PubMed;
(f) K. C. Mondal, H. W. Roesky, A. C. Stueckl, F. Ehret, W. Kaim, B. Dittrich, B. Maity and D. Koley, Angew. Chem., Int. Ed., 2013, 52, 11804–11807 CrossRef CAS PubMed;
(g) D. Martin, C. E. Moore, A. L. Rheingold and G. Bertrand, Angew. Chem., Int. Ed., 2013, 52, 7014–7017 CrossRef CAS PubMed;
(h) J. K. Mahoney, D. Martin, C. E. Moore, A. L. Rheingold and G. Bertrand, J. Am. Chem. Soc., 2013, 135, 18766–18769 CrossRef CAS PubMed;
(i) M. Y. Abraham, Y. Wang, Y. Xie, R. J. Gilliard Jr., P. Wei, B. J. Vaccaro, M. K. Johnson, H. F. Schaefer, P. V. R. Schleyer III and G. H. Robinson, J. Am. Chem. Soc., 2013, 135, 2486–2488 CrossRef CAS PubMed.
-
(a) C. Creutz and H. Taube, J. Am. Chem. Soc., 1969, 91, 3988–3989 CrossRef CAS;
(b) C. Creutz and H. Taube, J. Am. Chem. Soc., 1973, 95, 1086–1094 CrossRef CAS.
-
(a) P. Aguirre-Etcheverry and D. O'Hare, Chem. Rev., 2010, 110, 4839–4864 CrossRef CAS PubMed;
(b) K. Costuas and S. Rigaut, Dalton Trans., 2011, 40, 5643 RSC;
(c) P. J. Low, Coord. Chem. Rev., 2013, 257, 1507–1532 CrossRef CAS PubMed;
(d) J.-F. Halet and C. Lapinte, Coord. Chem. Rev., 2013, 257, 1584–1613 CrossRef CAS PubMed.
- D. O. Cowan, C. LeVanda, J. Park and F. Kaufman, Acc. Chem. Res., 1973, 6, 1–7 CrossRef CAS.
-
(a) J. Hankache and O. S. Wenger, Chem. Rev., 2011, 111, 5138–5178 CrossRef CAS PubMed;
(b) A. Heckmann and C. Lambert, Angew. Chem., Int. Ed., 2012, 51, 326–392 CrossRef CAS PubMed;
(c) M. Abe, Chem.
Rev., 2013, 113, 7011–7088 CrossRef CAS PubMed;
(d) Y. Su, X. Wang, X. Zheng, Z. Zhang, Y. Song, Y. Sui, Y. Li and X. Wang, Angew. Chem., Int. Ed., 2014, 53, 2857–2861 CrossRef CAS PubMed.
-
(a) M. Melaimi, M. Soleilhavoup and G. Bertrand, Angew. Chem., Int. Ed., 2010, 49, 8810–8849 CrossRef CAS PubMed;
(b) O. Back, M. Henry-Ellinger, C. D. Martin, D. Martin and G. Bertrand, Angew. Chem., Int. Ed., 2013, 52, 2939–2943 CrossRef CAS PubMed;
(c) T. Droege and F. Glorius, Angew. Chem., Int. Ed., 2010, 49, 6940–6952 CrossRef CAS PubMed.
- During the submission of this work, Roesky et al. published similar results using a gem-dimethyl CAAC scaffold: Y. Li, K. C. Mondal, P. P. Samuel, H. Zhu, C. M. Orben, S. Panneerselvam, B. Dittrich, B. Schwederski, W. Kaim, T. Mondal, D. Koley and H. W. Roesky, Angew. Chem., Int. Ed., 2014, 53 DOI:10.1002/anie.201310975.
-
(a) G. Maas, A. Fronda, D. Mayer and R. Brückmann, J. Organomet. Chem., 1994, 469, 115–123 CrossRef CAS;
(b) J. A. Januszewski, D. Wendinger, C. D. Methfessel, F. Hampel and R. R. Tykwinski, Angew. Chem., Int. Ed., 2013, 52, 1817–1821 CrossRef CAS PubMed.
-
(a) D. Himmel, I. Krossing and A. Schnepf, Angew. Chem., Int. Ed., 2014, 53, 370–374 CrossRef CAS PubMed;
(b) J. L. Dutton and D. J. D. Wilson, Angew. Chem., Int. Ed., 2012, 51, 1477–1480 CrossRef CAS PubMed.
Footnote |
† Electronic supplementary information (ESI) available: Synthetic procedures and analytical data; CIF files for single crystal X-ray structural analysis. CCDC 991467–991469. For ESI and crystallographic data in CIF or other electronic format see DOI: 10.1039/c4qo00072b |
|
This journal is © the Partner Organisations 2014 |
Click here to see how this site uses Cookies. View our privacy policy here.