DOI:
10.1039/C4QO00032C
(Research Article)
Org. Chem. Front., 2014,
1, 391-394
A concise method to prepare novel fused heteroaromatic diones through double Friedel–Crafts acylation†
Received
4th February 2014
, Accepted 26th February 2014
First published on 18th March 2014
Abstract
This paper explored the intramolecular double Friedel–Crafts acylation strategy in approaching different types of novel fused heteroaromatic diones (12 samples using 2,2′-bithiophene, 2,2′-bifuran, 2,2′-biselenophene, and 2,2′-bipyrrole as cores) with 16%–85% yield.
Fused aromatic or heteroaromatic diones (Scheme 1) have been demonstrated as powerful building blocks to construct versatile π-conjugated materials ranging from small molecules1–6 to conducting polymers,7 which can be used in organic solar cells,7 organic field-effect transistors,5,6b,7d,e,8 lithium-ion batteries,3e sensors,2b,3c,6c phototransistor devices,3d optical waveguides,6aetc. Especially, fused heteroaromatic diones are more attractive because the type, position, and valence of heteroatoms could offer more opportunities to tune the properties and characteristics of new materials constructed from them.9 In fact, fused heteroaromatic diones could offer us more opportunities to vary the properties of as-prepared materials through post-modification.10 Thus, it is a very urgent task to develop the corresponding method for preparing these fused heteroaromatic diones.
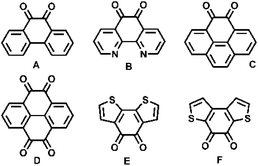 |
| Scheme 1 The reported structures of fused aromatic and heteroaromatic diones. | |
There are three main types of methods in the literature to prepare heteroaromatic-fused diones (using the benzo[2,1-b:3,4-b′]-dithiophene-4,5-dione (BDTD) core as an example, Scheme 2): (a) dialdehyde undergoes benzoin condensation in the presence of cyanide anions or 3,4-dimethyl-5-(2-hydroxyethyl)thiazolium iodide, followed by air oxidation (Scheme 2a);1c,10a (b) substituted 3,3′-dibromo-2,2′-bithiophene can be converted into diones via double lithiation with n-butyllithium, followed by reaction with an electrophile (diethyl oxalate or N,N′-dimethylpiperazine-2,3-dione) or CuBr/LiBr then oxalyl chloride (Scheme 2b);9,10b (c) diones are formed via lithiation of 3-bromo-thiophene with n-butyllithium, then reaction with N,N′-dimethylpiperazine-2,3-dione or CuBr/LiBr then oxalyl chloride, followed by intramolecular thiophene–thiophene coupling with FeCl3 (Scheme 2c).10b,11 However, these methods might not become a general method to prepare other important fused heteroaromatic diones such as 2,2′-bifuran, 2,2′-biselenophene, and N,N′-dimethyl-2,2′-bipyrrole for the following reasons: (1) some intermediates are not commercially available and need more steps to prepare; (2) some commercial sources are expensive or highly-flammable; and (3) some chemical agents such as cyanide are very toxic and are not environment-friendly. Thus, it should be highly desirable to develop a general, concise and efficient method to address these molecules. In this work, we developed a direct and concise method to synthesize fused heteroaromatic diones (X = S, Se, O, N) through intramolecular double Friedel–Crafts acylation (Scheme 2).
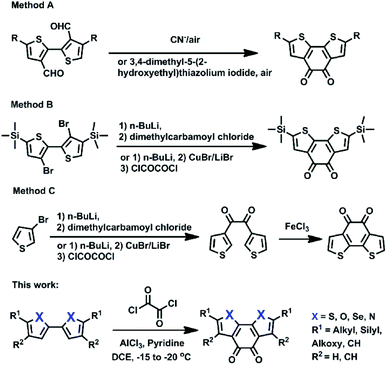 |
| Scheme 2 The synthetic methods to prepare fused heteroaromatic diones. | |
Although it was in 1911 that Liebermann et al.11b demonstrated the possibility of intramolecular double Friedel–Crafts acylation on 4,4′-dimethylbiphenyl, only one recent paper suggested that Friedel–Crafts acylation could be realized on heteroaromatic rings to produce heteroaromatic diones.12 Nevertheless, in this work,12 the Friedel–Crafts acylation happened on 2,2′-positions rather than 3,3′-positions, where 2,2′-positions are well known to be more reactive than 3,3′-positions. To the best of our knowledge, there is no report regarding the Friedel–Crafts acylation happening on 3,3′-positions of heteroaromatic rings. Hence, we became interested in examining the possibility of Friedel–Crafts acylation on 3,3′-positions of heteroaromatic rings including 2,2′-bithiophene, 2,2′-bifuran, 2,2′-biselenophene, and N,N′-dimethyl-2,2′-bipyrrole.
In order to test our ideas, we used 2,2′-bithiophene as a core and functionalized it with different substituent groups in 5 and 5′-positions (Table 1). The ratios of oxalyl chloride and aluminium chloride to reactants are 1.2 and 3.0, respectively. The effects of different substituent groups (e.g., alkyl groups, electron-donating groups, and electron-withdrawing groups) have been investigated. As shown in Table 1, both alkyl groups and silyl groups underwent intramolecular double Friedel–Crafts acylation in medium yields (25–48%). When the R1 group becomes more bulky (e.g., using t-butyl to replace methyl), the yield increases simultaneously (see entries 1, 2, 4 and 5). The reason for the low yield of compound 1d (trimethylsilyl group) might be due to the breakage of the C–Si bond, and accordingly, the intermolecular Friedel–Crafts acylation happens (see ESI: S5†). The intramolecular double Friedel–Crafts acylation reaction proceeded very well if a long chain was used (entry 3). Interestingly, when the strong electron-donating group methoxyl was employed, a relatively low yield (25%) was observed. Furthermore, when the electron-withdrawing group (e.g. CH3CO) was introduced in 5 and 5′-positions, no reaction happened due to the lower reactivity of compound 1g (acetyl group). If R1 was Br, no target molecule was observed and complex by-products formed. Thus, our results suggest that electron-rich substituents favor the intramolecular double Friedel–Crafts acylation.
Table 1 Intramolecular double Friedel–Crafts acylation with various bithiophene substrates
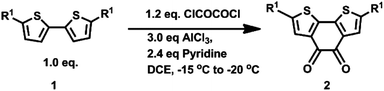
|
Entry |
Substrate |
R1 |
Product |
Yield (%) |
1 |
1a
|
Methyl |
2a
|
32 |
2 |
1b
|
t-Butyl |
2b
|
48 |
3 |
1c
|
n-Hexyl |
2c
|
37 |
4 |
1d
|
Trimethylsilyl |
2d
|
28 |
5 |
1e
|
Tri-i-propylsilyl |
2e
|
45 |
6 |
1f
|
Methoxyl |
2f
|
25 |
7 |
1g
|
Acetyl |
2g
|
No reaction |
8 |
1h
|
Br |
2h
|
No target molecule |
Given our success with intramolecular double Friedel–Crafts acylation of 2,2′-bithiophene derivatives, we decided to extend our method to systems containing 2,2′-bifuran, 2,2′-biselenophene, and N,N′-dimethyl-2,2′-bipyrrole as cores (Table 2). As shown in Table 2, due to its high reactivity, the target compound (4a) for bifuran (3a) can only be obtained in 16% yield when the reaction temperature decreases to −50 °C. As to 2,2′-biselenophene (3b) and N,N′-dimethyl-2,2′-bipyrrole (3c), the reaction can proceed smoothly with medium yield. It is worth noting that this is the first report of performing intramolecular double Friedel–Crafts acylation on these systems containing 2,2′-bifuran, 2,2′-biselenophene or N,N′-dimethyl-2,2′-bipyrrole as the cores.
Table 2 Intramolecular double Friedel–Crafts acylation with bifuran, biselenophene and bipyrrole
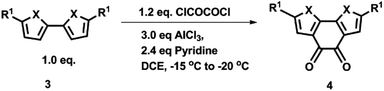
|
Entry |
Substrate |
X |
R1 |
Product |
Yield (%) |
Reaction temperature is −50 °C.
|
1a |
3a
|
O |
Trimethylsilyl |
4a
|
16 |
2 |
3b
|
Se |
Trimethylsilyl |
4b
|
37 |
3 |
3c
|
N–CH3 |
Methyl |
4c
|
32 |
Further explorations of intramolecular double Friedel–Crafts acylation on more complicated systems (e.g. 2,2′-bibenzofuran, 2,2′-bibenzothiophene, and 1,1′-dihexyl-1H,1′H′-2,2′-biindole) have also been demonstrated (Table 3). All reactions gave good yields (35–85%) under similar reaction conditions.
Table 3 Intramolecular double Friedel–Crafts acylation with bibenzofuran, bibenzothiophene and biindole substrates
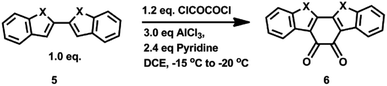
|
Entry |
Substrate |
X |
Product |
Yield (%) |
1 |
5a
|
O |
6a
|
35 |
2 |
5b
|
S |
6b
|
58 |
3 |
5c
|
N-n-hexyl |
6c
|
85 |
To demonstrate the usefulness of these intermediates, we carried out two derivative reactions (Scheme 3) and our results show that both reactions performed well with high yields. Moreover, the as-prepared products show very good solubility in organic solvents (>40 mg mL−1 in chloroform), which are desirable for device fabrication through spin coating.
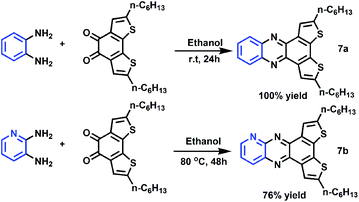 |
| Scheme 3 The derivative reactions based on the 2c. | |
Conclusions
In conclusion, this communication described our recent progress in the reinvestigation of intramolecular double Friedel–Crafts acylation for preparing fused heteroaromatic diones using 2,2′-bithiophene, 2,2′-bifuran, 2,2′-biselenophene, N,N′-dimethyl-2,2′-bipyrrole, 2,2′-bibenzofuran, 2,2′-bibenzothiophene, and 1,1′-dimethyl-1H,1′H′-2,2′-biindole as starting materials. Our success could offer a more convenient and concise method to create high-performance materials with versatile properties.
Acknowledgements
Q.Z. thanks Prof. Bendikov greatly for his generous gift of bifuran and biselenophene and acknowledges the financial support from AcRF Tier 1 (RG 16/12) from MOE, MOE Tier 2 (ARC 20/12), the CREATE program (Nanomaterials for Energy and Water Management) from NRF, and New Initiative Fund from NTU, Singapore.
Notes and references
-
(a) J. E. Kwon, S. Park and S. Y. Park, J. Am. Chem. Soc., 2013, 135, 11239 CrossRef CAS PubMed;
(b) K. Benelhadj, J. Massue, P. Retailleau, G. Ulrich and R. Ziessel, Org. Lett., 2013, 15, 2918 CrossRef CAS PubMed;
(c) J. A. Letizia, S. Cronin, R. P. Ortiz, A. Facchetti, M. A. Ratner and T. J. Marks, Chem.–Eur. J., 2010, 16, 1911 CrossRef CAS PubMed;
(d) R. A. Pascal, W. D. Mcmillan and D. Vanengen, J. Am. Chem. Soc., 1986, 108, 5652 CrossRef CAS;
(e) R. A. Pascal, W. D. Mcmillan, D. Vanengen and R. G. Eason, J. Am. Chem. Soc., 1987, 109, 4660 CrossRef CAS;
(f) J. Lu, D. M. Ho, N. J. Vogelaar, C. M. Kraml and R. A. Pascal, J. Am. Chem. Soc., 2004, 126, 11168 CrossRef CAS PubMed;
(g) J. C. Li, G. F. Hu, N. N. Wang, T. Hu, Q. D. Wen, P. Lu and Y. G. Wang, J. Org. Chem., 2013, 78, 3001 CrossRef CAS PubMed;
(h) N. Crosta, S. Müller, D. Gradl, K. S. Masters and S. Bräse, Synlett, 2013, 951 CAS.
-
(a) J. D. Spence, A. C. Rios, M. A. Frost, C. M. McCutcheon, C. D. Cox, S. Chavez, R. Fernandez and B. F. Gherman, J. Org. Chem., 2012, 77, 10329 CrossRef CAS PubMed;
(b) G. Li, Y. C. Wu, J. K. Gao, J. B. Li, Y. Zhao and Q. Zhang, Chem.–Asian J., 2013, 8, 1574 CrossRef CAS PubMed;
(c) D. Maity, C. Bhaumik, S. Karmakar and S. Baitalik, Inorg. Chem., 2013, 52, 7933 CrossRef CAS PubMed;
(d) J. P. Nietfeld, R. L. Schwiderski, T. P. Gonnella and S. C. Rasmussen, J. Org. Chem., 2011, 76, 6383 CrossRef CAS PubMed.
-
(a) M. Kastler, J. Schmidt, W. Pisula, D. Sebastiani and K. Müllen, J. Am. Chem. Soc., 2006, 128, 9526 CrossRef CAS PubMed;
(b) Q. Zhang, Y. Divayana, J. C. Xiao, Z. J. Wang, E. R. T. Tiekink, H. M. Doung, H. Zhang, F. Boey, X. W. Sun and F. Wudl, Chem.–Eur. J., 2010, 16, 7422 CrossRef CAS PubMed;
(c) M. Alfonso, A. Espinosa, A. Tarraga and P. Molina, Org. Lett., 2011, 13, 2078 CrossRef CAS PubMed;
(d) Y. C. Wu, Z. Y. Yin, J. C. Xiao, Y. Liu, F. X. Wei, K. J. Tan, C. Kloc, L. Huang, Q. Y. Yan, F. Z. Hu, H. Zhang and Q. Zhang, ACS Appl. Mater. Interfaces, 2012, 4, 1883 CrossRef CAS PubMed;
(e) T. Nokami, T. Matsuo, Y. Inatomi, N. Hojo, T. Tsukagoshi, H. Yoshizawa, A. Shimizu, H. Kuramoto, K. Komae, H. Tsuyama and J. Yoshida, J. Am. Chem. Soc., 2012, 134, 19694 CrossRef CAS PubMed;
(f) J. C. Xiao, B. Yang, J. I. Wong, Y. Liu, F. X. Wei, K. J. Tan, X. Teng, Y. C. Wu, L. Huang, C. Kloc, F. Boey, J. Ma, H. Zhang, H. Y. Yang and Q. Zhang, Org. Lett., 2011, 13, 3004 CrossRef CAS PubMed;
(g) E. T. Mack, A. B. Carle, J. T. M. Liang, W. Coyle and R. M. Wilson, J. Am. Chem. Soc., 2004, 126, 15324 CrossRef CAS PubMed;
(h) Y. Yamauchi, M. Yoshizawa, M. Akita and M. Fujita, J. Am. Chem. Soc., 2010, 132, 960 CrossRef CAS PubMed;
(i) S. N. Keller, N. L. Veltri and T. C. Sutherland, Org. Lett., 2013, 15, 4798 CrossRef CAS PubMed;
(j) J. C. Xiao, Y. Divayana, Q. C. Zhang, H. M. Doung, H. Zhang, F. Boey, X. W. Sun and F. Wudl, J. Mater. Chem., 2010, 20, 8167 RSC;
(k) G. Li, H. M. Duong, Z. H. Zhang, J. C. Xiao, L. Liu, Y. L. Zhao, H. Zhang, F. W. Huo, S. Z. Li, J. Ma, F. Wudl and Q. C. Zhang, Chem. Commun., 2012, 48, 1359 Search PubMed;
(l) J. C. Xiao, H. M. Duong, Y. Liu, W. X. Shi, L. Ji, G. Li, S. Z. Li, X. W. Liu, J. Ma, F. Wudl and Q. C. Zhang, Angew. Chem., Int. Ed., 2012, 51, 6094 CrossRef CAS PubMed;
(m) J. Xiao, C. D. Malliakas, Y. Liu, F. Zhou, G. Li, H. Su, M. G. Kanatzidis, F. Wudl and Q. C. Zhang, Chem.–Asian J., 2012, 4, 672 CrossRef PubMed;
(n) J. Xiao, S. Liu, Y. Liu, L. Ji, X. Liu, H. Zhang, X. W. Sun and Q. C. Zhang, Chem.–Asian J., 2012, 7, 561 CrossRef CAS PubMed;
(o) H. B. Liu, J. L. Xu, Y. J. Li and Y. L. Li, Acc. Chem. Res., 2010, 43, 1496 CrossRef CAS PubMed.
-
(a) B. X. Gao, M. Wang, Y. X. Cheng, L. X. Wang, X. B. Jing and F. S. Wang, J. Am. Chem. Soc., 2008, 130, 8297 CrossRef CAS PubMed;
(b) C. H. Tong, W. G. Zhao, J. Luo, H. Y. Mao, W. Chen, H. S. O. Chan and C. Y. Chi, Org. Lett., 2012, 14, 494 CrossRef CAS PubMed;
(c) L. Zophel, V. Enkelmann and K. Müllen, Org. Lett., 2013, 15, 804 CrossRef PubMed;
(d) C. H. Tong, W. G. Zhao, J. Luo, H. Y. Mao, W. Chen, H. S. O. Chan and C. Y. Chi, Org. Lett., 2012, 14, 494 CrossRef CAS PubMed;
(e) X. D. Yin, Y. J. Li, Y. L. Zhu, Y. H. Kan, Y. L. Li and D. B. Zhu, Org. Lett., 2011, 13, 1520 CrossRef CAS PubMed;
(f) Y. J. Li, L. Xu, T. F. Liu, Y. W. Yu, H. B. Liu, Y. L. Li and D. B. Zhu, Org. Lett., 2011, 13, 5692 CrossRef CAS PubMed.
- Y. A. Getmanenko, M. Fonari, C. Risko, B. Sandhu, E. Galan, L. Y. Zhu, P. Tongwa, D. K. Hwang, S. Singh, H. Wang, S. P. Tiwari, Y. L. Loo, J. L. Bredas, B. Kippelen, T. Timofeeva and S. R. Marder, J. Mater. Chem. C., 2013, 1, 1467 RSC.
-
(a) Z. H. Guo, T. Lei, Z. X. Jin, J. Y. Wang and J. Pei, Org. Lett., 2013, 15, 3530 CrossRef CAS PubMed;
(b) Y. F. Xie, T. Fujimoto, S. Dalgleish, Y. Shuku, M. M. Matsushita and K. Awaga, J. Mater. Chem. C, 2013, 1, 3467 RSC;
(c) R. Satapathy, Y. H. Wu and H. C. Lin, Chem. Commun., 2012, 48, 5668 RSC.
-
(a) Y. Zhang, J. Y. Zou, H. L. Yip, K. S. Chen, J. A. Davies, Y. Sun and A. K. Y. Jen, Macromolecules, 2011, 44, 4752 CrossRef CAS;
(b) Y. Zhang, J. Y. Zou, H. L. Yip, K. S. Chen, D. F. Zeigler, Y. Sun and A. K. Y. Jen, Chem. Mater., 2011, 23, 2289 CrossRef CAS;
(c) R. Mondal, N. Miyaki, H. A. Becerril, J. E. Norton, J. Parmer, A. C. Mayer, M. L. Tang, J. L. Bredas, M. D. McGehee and Z. A. Bao, Chem. Mater., 2009, 21, 3618 CrossRef CAS;
(d) R. Mondal, H. A. Becerril, E. Verploegen, D. Kim, J. E. Norton, S. Ko, N. Miyaki, S. Lee, M. F. Toney, J. L. Bredas, M. D. McGehee and Z. N. Bao, J. Mater. Chem., 2010, 20, 5823 RSC;
(e) S. Li, Z. C. He, J. Yu, S. A. Chen, A. S. Zhong, R. L. Tang, H. B. Wu, J. G. Qin and Z. Li, J. Mater. Chem., 2012, 22, 12523 RSC;
(f) Y. Lee, Y. M. Nam and W. H. Jo, J. Mater. Chem., 2011, 21, 8583 RSC;
(g) C. Y. Mei, L. Liang, F. G. Zhao, J. T. Wang, L. F. Yu, Y. X. Li and W. S. Li, Macromolecules, 2013, 46, 7920 CrossRef CAS.
- R. Mondal, S. W. Ko, E. Verploegen, H. A. Becerril, M. F. Toney and Z. A. Bao, J. Mater. Chem., 2011, 21, 1537 RSC.
- Y. A. Getmanenko, C. Risko, P. Tongwa, E. G. Kim, H. Li, B. Sandhu, T. Timofeeva, J. L. Bredas and S. R. Marder, J. Org. Chem., 2011, 76, 2660 CrossRef CAS PubMed.
-
(a) Y. Didane, A. Kumagai, N. Yoshimoto, C. Videlot-Ackermann and H. Brisset, Tetrahedron, 2011, 67, 1628 CrossRef CAS PubMed;
(b) F. A. Arroyave, C. A. Richard and J. R. Reynolds, Org. Lett., 2012, 14, 6138 CrossRef CAS PubMed.
-
(a) J. E. Zhang, W. Z. Cai, F. Huang, E. G. Wang, C. M. Zhong, S. J. Liu, M. Wang, C. H. Duan, T. B. Yang and Y. Cao, Macromolecules, 2011, 44, 894 CrossRef CAS;
(b) C. Liebermann and M. Zsuffa, Ber. Dtsch. Chem. Ges., 1911, 44, 852 CrossRef CAS.
- K. E. S. Phillips, T. J. Katz, S. Jockusch, A. J. Lovinger and N. J. Turro, J. Am. Chem. Soc., 2001, 123, 11899 CrossRef CAS PubMed.
Footnote |
† Electronic supplementary information (ESI) available: Details of experimental procedures, 1H NMR, 13C NMR, HRMS, etc. See DOI: 10.1039/c4qo00032c |
|
This journal is © the Partner Organisations 2014 |
Click here to see how this site uses Cookies. View our privacy policy here.