DOI:
10.1039/D1RA06087B
(Review Article)
RSC Adv., 2021,
11, 29080-29101
Lipid-based nanoparticles for psoriasis treatment: a review on conventional treatments, recent works, and future prospects
Received
11th August 2021
, Accepted 19th August 2021
First published on 1st September 2021
Abstract
Psoriasis is a lingering inflammatory skin disease that attacks the immune system. The abnormal interactions between T cells, immune cells, and inflammatory cytokines causing the epidermal thickening. International guidelines have recommended topical treatments for mild to moderate psoriasis whilst systemic and phototherapy treatments for moderate to severe psoriasis. However, current therapeutic approaches have a wider extent to treat moderate to severe type of psoriasis especially since the emergence of diverse biologic agents. In the meantime, topical delivery of conventional treatments has prompted many unsatisfactory effects to penetrate through the skin (stratum corneum). By understanding the physiology of stratum corneum barrier functions, scientists have developed different types of lipid-based nanoparticles like solid lipid nanoparticles, nanostructured lipid carriers, nanovesicles, and nanoemulsions. These novel drug delivery systems help the poorly solubilised active pharmaceutical ingredient reaches the targeted site seamlessly because of the bioavailability feature of the nanosized molecules. Lipid-based nanoparticles for psoriasis treatments create a paradigm for topical drug delivery due to their lipids' amphiphilic feature to efficiently encapsulate both lipophilic and hydrophilic drugs. This review highlights different types of lipid-based nanoparticles and their recent works of nano formulated psoriasis treatments. The encapsulation of psoriasis drugs through lipid nanocarriers unfold numerous research opportunities in pharmaceutical applications but also draw challenges for the future development of nano drugs.
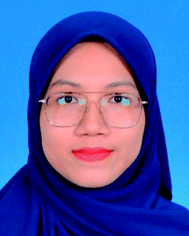 Ummu Umaimah Mohd Nordin | Ummu Umaimah Mohd Nordin received her BSc in Chemistry (Forensic Analysis) from Universiti Teknologi MARA, Shah Alam, Malaysia in 2016. She is currently a Graduate Research Assistant (GRA) while pursuing her master's degree in chemistry under the supervision of Dr Noraini Ahmad and Dr Norazlinaliza Salim at the Department of Chemistry, Faculty of Science, Universiti Malaya. Her current research interests focus on colloid chemistry and nanotechnology. |
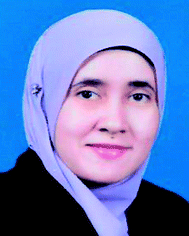 Noraini Ahmad | Noraini Ahmad received her PhD (2012) in Physical Chemistry from the Universiti Malaya. She went for a research attachment at the IQAC-CSIC, Barcelona, Spain in 2010 and 2011 funded by InForm Project, EU-FP7 and Ministry of Higher Education Malaysia, respectively. Presently, Noraini is an Associate Professor at the Department of Chemistry, Faculty of Science and Fellow of UM Community and Sustainability Centre (UMCares). Her research interests focus on colloidal chemistry, liquid crystals, and formulation of nanocarrier systems for cosmeceutical, pharmaceutical and nutraceutical applications. She has been awarded with several research grants and won numerous awards locally and internationally. |
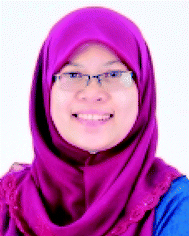 Norazlinaliza Salim | Norazlinaliza Salim received her PhD (2013) in Oleochemistry from the Universiti Putra Malaysia and went for a research attachment at the IQAC-CSIC, Barcelona, Spain (2010). In 2014, she was a post-doctoral research fellow at Centre for Fundamental and Frontier Sciences in Nano-structure Self-Assembly, Universiti Malaya and thereafter, was appointed as a senior lecturer at the Centre of Foundation Studies for Agricultural Science, Universiti Putra Malaysia. Her research interest is focussed on nanodelivery systems such as nanoemulsion, niosome, etc. for cosmeceutical and pharmaceutical applications. She has published over 25 papers in peer-reviewed indexed journals, one book chapter and five patents. |
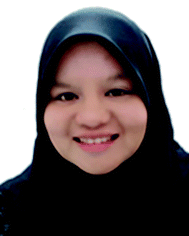 Nor Saadah Mohd Yusof | Nor Saadah Mohd Yusof obtained her PhD from the University of Melbourne in 2015. Presently, she is a senior lecturer at the Department of Chemistry, Universiti Malaya. Her research focuses on the micelle structures in the presence of various additives; and their transitional quantification using kinetics techniques. She is also working on sonochemistry and the effect of ultrasound on the micelle structures. Other than that, she also works on the use of ultrasound on other subjects such as to synthesize gold nanoparticles, on natural rubber, sonoencapsulation, sonoextraction, sonopolymerization and sonodegradation such as on food wastes and organic pollutants. |
1 Introduction
Psoriasis is an immune-mediated inflammatory dermatological disease that is also widely referred to as a systemic inflammatory disorder.1,2 Psoriasis can be indicated by a rapid build-up of cells on the skin's surface, forming erythematous (redness) papules and plaques with silver scales.3 The red, scaly plaques on the skin are itchy and sometimes painful.4 The psoriasis lesions typically formed on joints such as elbows and knees and may develop on any part of the skin such as hands, feet, scalp, neck, and face.5 The inflammation comes from the aberrant interactions between innate immune cells, T cells, and keratinocytes.6 However, psoriasis is non-contagious since it inherently involves innate and adaptive immune systems.7
The prevalence rate differs by region,8 which is epidemiologically attributable to racial factors, genetic background, lifestyle, and diet.9,10 Psoriasis prevalence amongst adults and children is reported to be ranging from 0.91% to 8.5% and 0 to 2.1%, respectively.11,12 Generally, it depicts a lower occurrence in Asian and some African populations but has a higher occurrence amongst Caucasian and Scandinavian people, notably in high-income countries.2,8 Nevertheless, the actual data amongst worldwide occurrence is not precise as Parisi et al. reported that the majority of low-income countries did not report the epidemiology of psoriasis in their countries.2
The disease may be triggered by several known factors or may also develop over the course of time. Infections from bacteria and viruses are reportedly exacerbated psoriasis conditions.13 Besides, certain medications (such as beta-blockers, lithium antimalarials, nonsteroidal anti-inflammatory medications, and tetracyclines) for adult psoriasis patients could also trigger the disease.14,15 They are also associated with numerous psoriasis comorbidities, including psoriatic arthritis, heart disease, hypertension, dyslipidaemia, obesity, type II diabetes, metabolic syndrome, inflammatory bowel disease, anxiety, and depression.14,16 From psychosocial impacts, psoriasis has been an intimidating disease as some studies show that the risk for anxiety and depression has become dominant comorbidities in psoriasis pathophysiology that influences patients' physical appearance and self-esteem.17,18
In determining the type of psoriasis (mild to moderate or moderate to severe), Psoriasis Area and Severity Index (PASI) score acts as the gold standard of severity lesion measurement, with PASI ≥ 10 indicating moderate to severe psoriasis.19,20 For mild to moderate psoriasis conditions, topical therapies are generally recommended over systemic therapies.3 The latter is preferable in cases involving moderate to severe disease conditions.3,21 Conventional topical delivery of antipsoriatic drugs typically utilises dosage methods at high concentrated therapeutics for a certain period.22 Nonetheless, this treatment possesses several limitations, including inadequate and unpredictable delivery to the afflicted skin as well as local toxicity due to skin irritation.22,23
Biologic drugs (biologics or biologic agents) are the recent treatment strategy with superior efficacy to other treatments for moderate to severe type of psoriasis.3,24 This treatment employs recombinant proteins to inhibit inflammatory cytokines at the target site while functions systemically.25 The advent of biologic agents has transformed the psoriasis treatment landscape after the first of its kind, etanercept, was approved by the Food and Drug Administration (FDA) in 2004.26
Currently, the breakthrough of psoriasis treatments emerges from the utilisation of novel drug carrier systems or nanotechnology-based approaches leading to greater drug efficacy and long-term effects.22,27,28 Poorly soluble active pharmaceutical ingredients (API) can be improved through the use of nanotechnology whilst increasing their bioavailability and physicochemical stability.28–32 It is presumed that ineffective APIs have low pharmacokinetic profiles, lack of stability and solubility, or ones with limited dose toxicity.33 Therefore, by employing novel drug delivery systems, these drawbacks can be overcome and thus improve the therapeutic actions.33–35 Nanoparticles have been proven in preclinical and clinical studies to reduce the drug's side effects at highly controlled drug delivery with relevant therapeutic concentrations.36 Nanoparticles through the application of nanomedicine are designed to improve the drug's half-life, thus facilitating the API delivery to its targeted action site through nanocarriers.33 Nano-based drug delivery or known as nanocarriers are nanosized particles that enhance the API's therapeutic effectiveness and reduce adverse effects. This is mainly because of their large surface area to carry the API.37 Nanocarriers are drug delivery systems employed to regulate the pharmacokinetics and pharmacodynamic properties of the drug of interest.38 Widespread attention to utilising topical nanocarriers for skin diseases has multiplied lately because they provide better skin permeation and controlled release at a reduced dosage regimen.21
Different interests in various types of nanoparticles have been widely investigated for topical psoriasis treatments. Polymeric nanoparticles, namely nanospheres39 and nanocapsules40 as well as polymer-based nanoparticles such as dendrimers,41 and polymeric micelles42 are a growing interest in these applications. Generally, many types of polymers are attractive biocompatible materials with proven favourable skin penetration and deposition effects.43–45 Despite so, their constituents are inclined to cytotoxicity while the cost of production is expensive for a scale-up and requires high-end instruments.45,46
Hydrogels, water-insoluble cross-linked hydrophilic polymers, have become a popular trend in psoriasis with/without nanoformulations lately, as they can improve anti-psoriatic activity after drug encapsulation. The gels can solely act as drug vehicles,47 or formulated into nanostructured supramolecules,48 or integrated as excipients in various types of nanocarriers, such as graphene oxide,49 polymeric nanoparticles,43 nanosponge,50 and niosomes.51 With the growing interest and research in hydrogels, it is expected that they would become a significant component of nano-based drug delivery systems in the future to further improve the therapeutic profiles.52
At a more advanced approach than conventional phototherapy treatments, photothermal therapy (PTT) is a new technology combined with nano-agents to kill cancer cells by localised thermal damage,53 which can also be utilised for psoriasis treatments. PTT results in the apoptosis of keratinocytes upon photo absorption of nano-agents that is activated by light.54 Nirmal et al. developed gold nanorods and isatin (an anti-inflammatory agent) loaded into poly(lactic-co-glycolic acid) (PLGA) to form nanocomplexes for PTT, which was found to be able to reduce hyperthermia as a result of near-infrared (NIR) irradiation and also improve psoriasiform hyperproliferation.54 Nevertheless, the use of gold as a nano-agent is associated with low photostability, which is liable to structural deformation.55 Photodynamic therapy (PDT) is also a form of phototherapy, which is illuminated by a laser light beam of a specific wavelength, matching the absorption band of the topical administrated photosensitizer drug in psoriasis treatment. For example, a study reported by Liu et al.56 showed that zinc phthalocyanine (ZnPc) via PDT significantly inhibited the hyperproliferation of mouse vaginal epithelium induced by diethylstilbestrol and improved propranolol- and imiquimod-induced psoriasis-like symptoms. However, the PDT may perform damage to the plasma membrane or DNA of harmful cells, which may lead to cell death due to the highly reactive 1O2 generated in situ. Thus, nanocarriers based on carboxymethyl chitosan loaded with ZnPc has been reported.57
Lipid-based nanoparticles (also known as lipid-based nanocarriers or nano-delivery systems) have been futuristic for topical nanocarriers in the treatments of wound-healing activity of skin diseases such as those found from psoriasis.58–60 Such interest in lipid-based nanoparticles is the result of their low toxicity potential and much easier sample preparation with low-production cost allowing mass industrial production, and better thermal stability when compared to other types of nanoparticles.61,62 On top of that, their constituents are also more biodegradable and biocompatible relative to synthetic polymer constituents like in other types of nanocarriers.58,63
In this review, the pathophysiology of psoriasis is briefly explained such as the interaction of the inflammatory infiltrating cells with specific cytokines and alleles in the dermal layers. Multiple diagnosis strategies and the conventional treatments as recommended by dermatologists depending on the types of psoriasis are presented to provide information on different existing therapies that are regulated worldwide. As psoriasis is a skin disease, the skin penetration barrier of the stratum corneum and the challenges in delivering current therapies are further explained. This includes the discussion on unsatisfactory results from existing approaches. Subsequently, the emerging roles of lipid-based nanocarriers including their pros and cons are to be associated with the conventional strategies of psoriasis treatments, as well as their recent works. Lastly, current opportunities and challenges that arise from this novel therapeutic in treating psoriasis are also discussed comprehensively.
2 Pathogenesis of psoriasis
Psoriasis is a T cell-mediated inflammatory disease originating from the autoimmune system of the body. It is also construed to stem from a combination of genetic, epigenetic, and environmental factors,64 and also attributable to endocrine hormones and immunological factors.10 Human leukocyte antigen (HLA) alleles (also known as major histocompatibility complex (MHC) molecules) that reside in both innate and adaptive immune systems and other susceptibility loci that bear genetic factors lead to the risk of developing psoriasis.65–67 It remains vague whether the inciting antigens are self-antigens, environmental antigens, or some combination of the two to a certain extent.67 This autoimmune expedites the life cycle of skin cells and often be characterised by an excessively rare hyperproliferation of keratinocytes,64 caused by inflammatory cell infiltration that generates into the epidermis and dermis skin layers.11 Inflammatory cells are from many types of leukocyte population including lymphocytes, monocytes, and neutrophils that are accelerated by tumour necrosis factor (TNF)-α, a type of inflammatory cytokine aided by dendritic cell activation.10,68 Hence, the feedback loop between adaptive immune cells (T cells), innate immune cells (e.g., dendritic cells, Langerhans cells, natural killer cells, macrophages, granulocytes), and resident skin cells (e.g., keratinocytes, endothelial cells) will increase the inflammatory response, thus, cause cell hyperproliferation.69
TNF-α has the highest concentration of cytokine in psoriatic lesions since it is the target of immune response that is produced by various skin cells such as activated T cells, natural killer cells, keratinocytes, and Langerhans cells.70 Psoriatic lesions are also indicated by the rich in mature CD4+ helper T (Th1 and Th17) and CD8+ cytotoxic T cells as well as the increase in both Th1 and Th17-related cytokines.67,70 The major pathway of antigens and cytokines in the psoriasis pathophysiology is illustrated in Fig. 1 and summarised below.
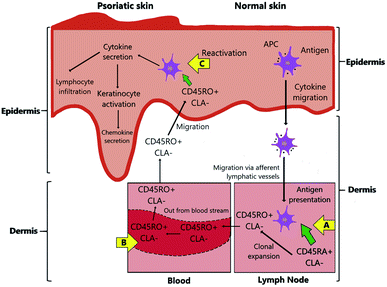 |
| Fig. 1 A schematic pathway diagram of psoriasis pathology. | |
(1) Antigen-presenting cells (APCs), such as Langerhans cells (the specialised form of dendritic cells), act as T-cell recognition. They mediate specific HLA antigens that carry psoriasis alleles to migrate from the epidermis to the lymph nodes via afferent lymphatic vessels. The migration process is promoted by inflammatory cytokines such as TNF-α and interleukin (IL)-1β. The APCs present their antigens (a process called antigen presentation) to naïve T cells, which results in the activation of T-cell proliferation in the dermis to regulate the immune response.70–72
(2) Dermal dendritic cells activate Th1 and Th17 infiltrate by secreting pro-inflammatory cytokines IL-12 and IL-23, respectively. Besides, other APCs (inflammatory dendritic cells) help secrete IL-18, IL-23, and TNF-α cytokines. This process is also accompanied by the change of phenotype of the naïve T cells, from CD45RA that are often expressed as cutaneous lymphocyte-associated antigens (CLA) on their surface (known as CD45RA+ CLA−), to the effector memory T cells, CD45RO+ CLA−.70,72–74
(3) The memory CD45RO+ CLA− T cells are accompanied by the Th1 and Th17 cells to be carried out through the blood vessels from the dermis to the epidermis as the result of the intradermal proliferation of T-cells.74
(4) In the epidermis, the natural killer T cells are responsible for direct antigen presentation due to their proximity to the keratinocytes. The activated Th1 cells are stimulated by IL-18 and IL-23 to secrete interferon-gamma (IFN-γ) cytokine. Meanwhile, Th17 cells are stimulated by IL-23 to secrete IL-17 and IL-22 cytokines.70 Then, the IL-23/IL-17 immune axis induces the infiltration of skin resident innate lymphocytes.75 IFN-γ enhances IL-22 to initiate keratinocyte activation causing epidermal acanthosis (skin thickening) such as found in plaque psoriasis,72,76 thus the activation also induces IL-17 family to promote keratinocyte amplification and further chemokine secretion.77
3 Treatment options and management of psoriasis
Psoriasis is an acute recurrent disease that requires long-term therapy, therefore tailored treatment is normally recommended based on the drug's compatibility with the patient.78 Another pertinent factor is the patient's background in terms of gender, age, and previous medical history since individuals respond to drugs is somehow unique. The quality of life and the effects of the disease on the patient's responsiveness and tolerability are also essential psychosocial assessments to be evaluated by dermatologists before prescriptions.79
In general, there are three different treatment options according to the type of psoriasis, which are topical, systemic, and phototherapy. Milder topical treatments can be recommended for patients with mild to moderate psoriasis. On the other hand, systemic treatments that include oral and biologic drugs are suitable for patients with moderate to severe type of psoriasis. Phototherapy treatment is commonly suggested for patients with moderate to severe type of psoriasis in the event of unsuccessful topical and systemic treatments. A list of treatment options under topical, systemic, and phototherapy are depicted in Fig. 2.21,27
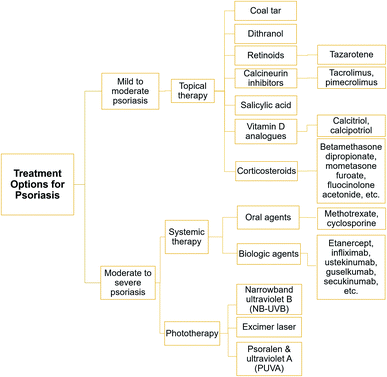 |
| Fig. 2 Currently available treatment options by type of psoriasis. | |
3.1 Topical treatments
The recommended first line of defence is topical treatment, in which the therapeutic is applied onto the afflicted skin. Such topical formulations are typically rendered in cream, solution, ointment, and shampoo (for scalp treatment). A wide range of topical treatments is available to treat psoriasis with different mechanisms of action. Topical drugs are expedient and accessible but usually deliver better efficacy in combination with other topical agents. In this section, the type of topical agents is sorted by older to the latest treatments relevant to the medications.
3.1.1 Coal tar. Since ancient times, coal tar has been part of psoriasis treatment, especially for scalp psoriasis but shows declining popularity in the past decades when compared to other tropicals.80,81 This is probably because of its cosmetically unpleasant nature such as malodorous smell and staining of cloth, especially in patients with non-black hair.80,82 Out of thousand compounds constituted in the tar, polycyclic aryl hydrocarbon is the most analysed compound as it is believed to generate an effect in psoriasis treatment.83 Contrariwise, carbazole of aryl hydrocarbons is suggested to exhibit a potent effect in the coal tar.83 Even so, coal tar has raised concerns among clinicians and patients due to its thousands of unidentified components.84 It is also banned in Canada and the European Union for cosmetic use because of carcinogenic concerns.85
3.1.2 Dithranol. Dithranol (also known as anthralin) is one of the oldest treatments to alleviate plaque psoriasis86 through inhibiting proliferation and inducing keratinocyte apoptosis, as demonstrated through human keratinocyte (HaCaT) cells.87,88 In essence, the direct or indirect effects of dithranol on the inflammatory response of psoriasis are not entirely comprehended, although they have been investigated by multifaceted studies.89,90 Notwithstanding the statement, a recent study showed that topical dithranol leads to a rapid reduction in the PASI score, resultant in a decrease in epidermal hyperproliferation and delayed reduction of inflammatory penetration in psoriatic skin.89 The study also suggested that psoriatic activity can be suppressed by targeting the keratinocytes (residing in the epidermis) rather than the immune cells. Despite the benefits, dithranol may not be powerful enough for skin permeation as it is associated with low solubility and stability, toxicity, staining, and skin irritation.91
3.1.3 Retinoids. Retinoids are the derivatives or vitamers of Vitamin A. The sole FDA-approved retinoids for psoriasis treatments are tazarotene and acitretin.92 Tazarotene is a topical drug, whilst acitretin is an oral agent. The retinoids work on retinoic acid receptors and retinoid-X-receptors, initiating gene expression's modification of inflammatory cytokines and thus, inhibits the keratinocyte proliferation. However, topical tazarotene can cause localised toxicity such as skin irritation and photosensitivity.93,94 Tazarotene's efficacy has been widely studied through combination with topical corticosteroids as they optimally enhance the post-treatment effects of psoriasis, in which tazarotene reduces the irritation whilst corticosteroids provide anti-inflammatory effects.95,96
3.1.4 Calcineurin inhibitors. Tacrolimus and pimecrolimus are two different types of topical calcineurin inhibitors (immunosuppressants) that act as the first-line therapeutic options for inverse psoriasis (body fold area) for long-time use.97,98 They are functional in anti-inflammatory and immunomodulatory activities to inhibit calcineurin phosphatase; hence, suppressing T-cell activation and cytokine production.99 Tacrolimus is also used to treat genital psoriasis, is well-tolerated amongst adults, and is suitable for patients who are sensitive to steroids but with certain side effects like mild pruritus and burning sensation onset the treatment.100–102 Similarly, pimecrolimus has also been used for such psoriasis conditions and facial psoriasis with equivalent potency in children but has less therapeutic efficacy than tacrolimus in adults.98,101,102
3.1.5 Salicylic acid. Salicylic acid is a potent keratolytic agent, often used in combination with other topical agents such as corticosteroids and calcineurin inhibitors. Its combination with calcineurin inhibitors is believed to help in the excellent drug absorption into psoriasis plaques.103 The use of salicylic acid alone for scalp psoriasis may result in temporary shedding of telogen hair, but the cessation of use may resolve the issue.104 Besides, it is not recommended to use salicylic acid with a concentration greater than 10% on large body surface areas. This is mainly to avoid possible side effects such as oral mucosal burn, frontal headache, and nausea. Some previous research has shown the potent effects of salicylic acid as a penetration enhancer through combination with a calcineurin inhibitor, vitamin D analogue, and corticosteroid for better skin permeation and sustained release profile.105–107
3.1.6 Vitamin D analogues. Calcipotriol and calcitriol are two types of vitamin D analogues derived from sunlight. Presumably, vitamin D deficiency is associated with psoriasis as its presence at an adequate amount helps suppress the production of inflammation's potent cytokine mediators, namely IL-2, IL-6, and IFN-γ; thus, keep the body healthy. Moreover, there is a correlation between Th17 cells (that work for a healthy immune system) and vitamin D in psoriasis patients.108 Vitamin D evidently stimulates suppressor T cells that regulate other cells in the immune system and inhibits cytotoxicity and, thus, is a natural killer of cell hyperproliferation. Nevertheless, their association remains debatable, albeit well-documented,109 and vitamin D analogues are often recommended with other topical agents. Calcipotriol and betamethasone dipropionate (a type of corticosteroid) has been studied widely to treat trunk and limb psoriasis as they both work synergistically to reduce inflammation. The combination therapy of the two helps control the imbalance between regulatory CD8+ or CD4+ T cells and pro-inflammatory CCR6+ gamma delta (γδ) T cells for antipsoriatic activity.110
3.1.7 Corticosteroids. Corticosteroids are the mainstay for topical therapies of psoriasis. They work by inhibiting the release of phospholipase A2 and act directly on deoxyribonucleic acid (DNA) and inflammatory cytokines.111 The use of corticosteroids alone acts as first-line topical therapy by reducing inflammation and slowing down cell hyperproliferation, especially in genital psoriasis.112 As discussed earlier, the effects of corticosteroids are more powerful through combination with other topical agents, namely retinoids, salicylic acid, and vitamin D analogues, as evidenced by multiple studies. However, local side effects like perioral dermatitis, striae, hypertrichosis, and infections are reportedly developed from the long-time use of topical corticosteroids.113 On a side note, studies showed that there is a moderately high perception of steroid phobia among our current generation, especially in women.114,115 In general, the studies indicate that the fear of using steroid-related drugs are due to skin deterioration conditions, weight gain, asthma, and stunted growth after obtaining sources from dermatologists and media.
3.2 Systemic treatments
The systemic treatment utilises drug that circulates through the whole body. It can be divided into two types: (1) oral agent; and (2) biologic agent that is administered into the system by injection or intravenous (IV) infusion. International guidelines for psoriasis treatments have recommended that if topical and phototherapy treatments are deemed unsuccessful, systemic nonbiologic (oral agent) and biologic therapies may be pursued to achieve skin clearance. Since monotherapy of topical treatments to moderate to severe psoriasis causes many dissatisfactions, patients can also benefit from systemic therapies that provide better efficiency and favourable tolerability to reduce clinical symptoms, accomplish treatment efficacy targets, and improve their quality of life.116
3.2.1 Oral drugs. Systemic nonbiologic therapies work by suppressing inflammatory responses in the form of oral consumption. Methotrexate and cyclosporine are the two famous oral, systemic therapies used for decades to treat psoriasis, often recommended as monotherapy or other systemic therapies. Although biologics have widespread attention in psoriasis treatments recently, systemic nonbiologic therapies are with many advantages. Oral systemic generally curb pervasive psoriasis, is lower cost than older medications and biologics, and has the convenience of use and availability.117 Besides can be administered orally, the active ingredients are also loaded in nanoformulations to treat psoriasis topically.
3.2.1.1 Methotrexate. Methotrexate is a comprehensive treatment for all types of psoriasis conditions. It normalises psoriasis conditions by inciting the apoptosis process of keratinocyte proliferation.118 It is usually administered weekly at a low single dose to reduce side effects before the dose can be gradually increased to achieve its optimal efficacy.119 However, the continuous use of methotrexate would cause liver damage and pulmonary toxicity (lung damage). Besides, myelosuppression (low blood cell production) and immunosuppression (weakened immune system) are other problems associated with methotrexate's long-term use. It is also reported that methotrexate-receiving patients have a higher cardiovascular event risk than TNF inhibitor users.120
3.2.1.2 Cyclosporine. Cyclosporine (sometimes spelt as cyclosporin and known as cyclosporine A) is another type of calcineurin inhibitor that has been one of the most effective systemic psoriasis treatments. Both cyclosporine and methotrexate's rotational use are found to help reduce long-term kidney toxicity (nephrotoxicity) whilst minimising the adverse impacts of plaque psoriasis.121 The use of cyclosporine drug is reportedly equally safe and effective for severe paediatric and adult plaque psoriasis patients122 but with mild side effects such as pain abdomen and an increase in serum creatinine (a chemical waste product in the blood).123,124 However, liver toxicity (hepatotoxicity) has been subject to the most affected organ for prolonged use of cyclosporine.125
3.2.2 Biologic drugs. After the successful case of treating a patient that suffered from Crohn's disease and moderate to severe psoriasis with a TNF-α blocker in 2000,126 the last decade witnessed the discovery of IL-17 and IL-23 pathways responsible for the pathogenesis of psoriasis.127,128 Biologics have pragmatic strength of recommendations for psoriasis treatments, typically recommended based on the patient's case evidence and the drug's performance. The American Academy of Dermatology (AAD) and the National Psoriasis Foundation define biologic agents as “engineered monoclonal antibodies and fusion proteins that exert their therapeutic actions by blocking specific cytokines or cytokine receptors critical to psoriatic inflammation”.26 Presently, there are 11 types of FDA-approved biologic agents for adult psoriasis treatments, classified by their cytokine classes (TNF, IL-12, IL-23, IL-17A) (Fig. 3). Secukinumab, as the first-line systemic biological treatment129 is discussed to represent the overview of this type of advanced drug.
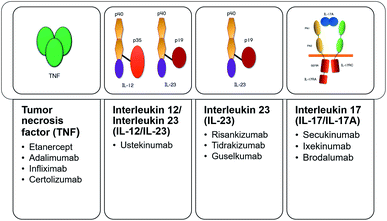 |
| Fig. 3 FDA-approved biologic agents by their classes. | |
Secukinumab (Cosentyx®) inhibits IL-17A from binding to its cytokine receptor for inflammation.130 It is highly favourable in safety profile as its efficacy demonstrates comparable with the commonly used psoriasis treatments (e.g., methotrexate and TNF-α blockers).131 Its efficacy is widely reported in treating moderate to severe plaque psoriasis132 and psoriatic arthritis.133 Other than that, those with a past treatment failure, difficult-to-treat patterns of psoriasis (nail, scalp, and palmoplantar psoriasis),134 and have clear skin desire135 can also be benefited from this biologics. Having said that, secukinumab is also perceived to have the most frequent side effects, mainly infections, compared with other biologic agents.136 It has been reported that nasopharyngitis, upper respiratory tract infections, and headaches are the expected side effects of secukinumab.133
Overall, biologic agents are considered expensive or as high-investment treatment based on the type of biologics and individual's PASI score.137 In the wake of the globally impacted pandemic era of COVID-19 (novel coronavirus disease) that severely affects the human respiratory system, the continuation of biologic treatments is controversial. This is due to the high respiratory rate before the pandemic emerged.138 That raises concern if it could increase the consumers' susceptibility to the virus. On the contrary, treatment discontinuation would cause loss of response when treatments are reintroduced or further exacerbated by antibody formation to the biologics.138
3.3 Phototherapy treatments
Photo-based therapy has been developed for psoriasis treatments since the 1920s, and presently serves as a mainstay for the moderate to severe type of psoriasis.139 In general, phototherapy is deemed an effective and comparatively affordable treatment. For instance, it is estimated that a 3 year phototherapy treatment would cost about $5000 as compared to the 3 year duration cost of secukinumab at about $182
718.140 Phototherapy treatments also have relatively rare side effects, particularly immunosuppression as compared to systemic therapies.141,142 Presently, narrowband ultraviolet B (NB-UVB), excimer laser/lamp (targeted phototherapy), and psoralen plus ultraviolet A (PUVA) are three different types of phototherapy treatments to treat psoriasis as recommended by international guidelines.
Nevertheless, phototherapy might be unpopular among psoriasis patients due to concerns about its carcinogenicity and is therefore not recommended for long-term treatment.141 The declining usage of phototherapy is also contributed after the advent of biological agents.3,143 Moreover, NB-UVB is also not suitable for psoriasis patients with photosensitive disorders (such as those suffering from xeroderma pigmentosa).141,143 Other than that, the literature reveals that PUVA undergoes a higher adverse risk than UVB and is not recommended for maintenance therapy due to cutaneous malignancy risks.144
3.3.1 Narrowband ultraviolet B (NB-UVB) and excimer laser. NB-UVB light (311 nm) and excimer lamp/laser (308 nm) are two effective phototherapies that currently act as the first-line treatment for stable plaque psoriasis.145 Both therapies are equally useful for psoriasis' skin clearance.141,145 The choice of NB-UVB is usually made after the failure of a series of topical agents.146 Phototherapy requires repetitive skin exposure to UV light. The generation of phototherapy works where the UV radiation from the treatments induce apoptosis in T lymphocytes and keratinocytes in the epidermis to control the psoriatic lesions.147 The trend of the highly cost-effective UVB treatment is the exclusive option for a particular group of patients, such as human immunodeficiency virus (HIV), internal malignancy, and pregnancy, where contraindication of their systemic immune reaction might happen (if modified while using other psoriasis treatments).148The excimer laser is remarked to be more efficacious than the excimer lamp and is recommended by the AAD for PASI < 10.141 Whilst other parts of the body can be induced by the NB-UVB phototherapy, the nose, ear, and palpebral region represent difficult-to-treat areas of psoriasis. Therefore, the excimer laser is a better phototherapy alternative to target those mentioned psoriasis areas.149 Besides being a powerful and effective treatment, it is preferable amongst psoriasis patients as the laser offers long-term remission of psoriasis.150
3.3.2 Psoralen plus ultraviolet A (PUVA). PUVA is a photochemotherapy treatment considered another effective treatment of chronic plaque psoriasis.151 The combination of psoralens and UVA has been shown to reduce PASI by 75% to 80% amongst the users, making this phototherapy useful as a second-line treatment after unresponsive topical and NB-UVB treatments.152 Instances of specific failure treatment indications of NB-UVB relapse are when the patients develop pustular psoriasis and pityriasis rubra pilaris conditions. Consequently, to introduce PUVA phototherapy to the patients after such psoriasis deterioration, psoralen needs to be sensitised upon them as a form to enhance the therapeutic procedure.146 Psoralen is a group of light-sensitive compounds derived from natural plants, commonly found in Psoralea corylifolia (Babchi) that exhibit therapeutic properties.153 They are likely to instigate skin-related conditions namely burning, itching, and pigmentation, and also nausea after this therapeutic action.154
4 Overcoming skin barrier challenges for nano-based drug delivery
A thorough analysis and understanding of the various skin's structures and physiology is required to design drugs with improved permeability and skin penetration. Skin is the largest organ in the human body and is also responsible for protecting one's system against exogenous pathogens and environmental factors and preventing water and electrolyte loss from the internal.155,156 As a result, the stratum corneum prevents the penetration of larger molecules (>500 Da)157 across the outermost layer of the skin epidermis. The barrier is referred to as a ‘brick-and-mortar system’ and comprises approximately 15 layers of flattened corneocytes (anucleated keratinocytes) within a multilamellar, lipid-rich extracellular matrix,158 The three essential components of stratum corneum are: (1) natural moisturising factor (NMF)-laden and lipid-bound corneocytes (terminally differentiated keratinocytes); (2) corneodesmosomes (proteinaceous rivets holding corneocytes together); and (3) lipids as shown in Fig. 4.
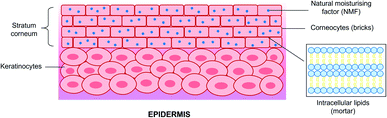 |
| Fig. 4 The ‘brick-and-mortar’ system of the stratum corneum within the epidermis. | |
Optimal skin barrier function can be achieved by the correct stratum corneum lipid lamellar structure with well-structured lipid bilayers, a massive amount of phytosphingosine-containing ceramides, and a high stratum corneum lipid/protein ratio.159 Other than that, the lipid matrix is also vital in the skin barrier function. Ceramides, fatty acids, and cholesterol are the principal components of the skin barrier lipids present in an equimolar ratio.160 Headgroup chemistry (hydroxyl group's number and position) and tail structure (unsaturation of sphingoid moiety) play parts in the ceramide bilayers' structural and transport properties.161
As a result of the complicated skin barrier lipids, nanoparticles may act as a tool to overcome the complexity of the stratum corneum in which chemical enhancers are unable to. In this way, nanoparticles stimulate drugs' residence time by channelling them to the target size while improving their penetration.162 As conventional topical treatments possess low drug permeation across the scaly psoriasis lesions, nanoparticles' microscopic morphology ensures that drugs can penetrate through the skin layers effectively.
It is widely regarded that topical drug delivery is the ideal route for skin diseases owing to its ability to deliver a high concentration of drugs whilst reducing systemic toxicity,163 as illustrated in Fig. 5. Its mechanism allows the API or drug to pass through the skin to the local therapy or across the skin by systemic treatment. Topical drug formulations like cream, gel, and ointment have been studied for local effect rather than systemic action. Thus, chemical enhancers like surfactants, alcohols, and amines are employed to deliver active therapeutics by overcoming the skin barrier (topical delivery).164 Besides topical, transdermal drug delivery can also target local skin disease and reach the bloodstream (systemic treatment). In transdermal delivery, the drug's systemic circulation (distribution process) is deemed successful upon skin absorption to the body's tissues via capillary action at a specific rate.165 In a common occurrence, the release of drugs are influenced by environmental parameters such as pH, redox reaction, and enzymes.37
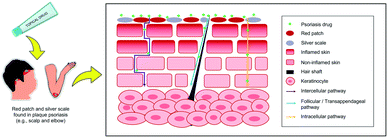 |
| Fig. 5 The pathways of topical drug delivery for psoriasis treatments. | |
5 Types and properties of lipid-based nanoparticles for psoriasis treatments
Lipids (Fig. 6) are amphipathic since they possess both the hydrophilic (polar head group) and the hydrophobic (non-polar tail group) properties.166 They self-assemble in aqueous solutions to minimise contact of their non-polar part with the water molecules. This characteristic allows them to spontaneously organise themselves into ordered patterns without human interference.167 This usually results in a micelle formation or micellization process when the surfactant concentration is above the critical micelle concentration (CMC).167,168 Such a phenomenon is one of the most important physicochemical properties since many types of lipid-based are micelles and act as surface-active agents (surfactants). Micelles act as carriers for the majority of hydrophobic (lipophilic) drugs upon their incorporation at nanoscale size whereby their architecture has a resemblance to lipid-based nanoparticles.169 The micellar resemblance of drug carriers has benefited nearly all possible drug administrations to increase bioavailability whilst reducing adverse effects.170
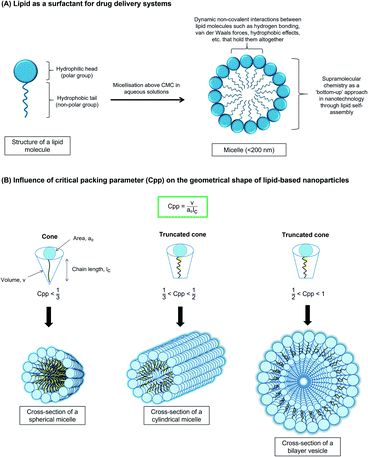 |
| Fig. 6 Properties of lipids as nanocarriers. | |
In addition to CMC, the critical packing parameter, Cpp of the lipid surfactants also governs the geometrical shape of the aggregates,171–173 as shown in Fig. 6. Their self-assembly morphology in an aqueous medium generally can be predicted to be either spherical micelles, cylindrical micelles or vesicles, as proposed by Israelachvili.171–173 For example, spherical (Cpp < 1/3), cylindrical, (1/3 < Cpp < 1/2), and vesicles (1/2 < Cpp < 1). Traditionally, the self-assembly properties and supramolecular chemistry are important for drug delivery systems through the bottom-up approach in nanotechnology.174,175 Supramolecular chemistry is generally referred to as the soft and dynamic non-covalent interactions of the assemblies, such as hydrogen bonding and van der Waals forces, but the interactions are capable of holding the amphiphiles together.174,176,177 This bottom-up approach has been an exciting route before the aggregates are formed into their shapes that are mainly influenced by their Cpp prediction.171,174 Therefore, the stable colloidal lipid systems in nanoscale supramolecular structures help overcome skin physiological barriers for optimised targeting and controlled release of drug delivery.168,178,179
Solid lipid nanoparticles (SLNs), nanostructured lipid carriers (NLCs), liposomes, niosomes, transfersomes, ethosomes, and nanoemulsions are classified as lipid-based nanoparticles because of their lipid arrangements. This section focuses on these different types of lipid-based nanoparticles because of their significant applications in psoriasis treatments lately. Their structural representations are illustrated in Fig. 7. This section also describes their overall concept, roles, components, preparation methods, advantages, and disadvantages for topical and transdermal psoriasis delivery. Recent up-to-date works involving psoriasis drugs loaded in the lipid-based nano delivery systems (nanoformulations) are compiled in Table 1.
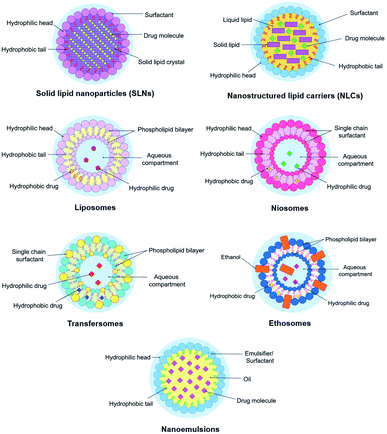 |
| Fig. 7 Structural diagrams of lipid-based nanoparticles. | |
Table 1 Recent developments of lipid-based nanoparticles for psoriasis treatments (2016–2020)
No. |
Lipid carrier |
Antipsoriatic agent |
Route of drug administration |
Method |
Remark |
Reference |
1 |
Solid lipid nanocarriers (SLNs) |
Methotrexate and etanercept |
Topical |
Hot ultrasonication |
Prolonged drug release in vitro and nontoxic towards human keratinocytes and fibroblasts |
272 |
Cyclosporine |
Topical |
Microemulsion |
Exhibited systemic absorption and reduced side effects whilst increased its concentration in the skin layers in vitro |
273 |
Thymoquinone (Nigella sativa extract) |
Topical |
Melt-emulsification and ultrasonication |
Low skin irritation score and reduced symptoms in erythema, oedema, and thickening in PASI score |
274 |
Tacrolimus |
Topical |
Emulsification and low-temperature solidification |
High ex vivo skin penetration value and deeper in vivo skin layer penetration |
275 |
2 |
Nanostructured lipid carriers (NLCs) |
Methotrexate |
Topical |
Solvent diffusion |
Significant entrapment efficiency and drug deposition whilst no erythema was indicated at primary skin irritation index |
276 |
Mometasone furoate |
Topical |
Microemulsion |
High skin deposition and low primary skin irritation index with complete clearance of parakeratosis in vivo |
277 |
Dithranol |
Topical |
Hot melt homogenisation |
Reduced the symptoms by PASI score and enzyme-linked immunosorbent assay with depletion in disease severity and cytokines like ILs-17, 22, 23, and TNF-α |
278 |
Methotrexate |
Topical |
Solvent diffusion |
Improved therapeutic response and reduced local side effects in vivo with a decline of PASI score, oxidative stress, inflammatory cytokines like TNF-α, IL-1β, and IL-6, and IMQ-induced histopathological alterations in mouse ear models |
279 |
3 |
Liposomes |
Cyclosporine |
Topical |
Thin-film hydration |
Showed acceptable safety profile in the remedy of chronic plaque psoriasis |
280 |
Psoralen |
Topical PUVA |
Cationic liposomes by thin-film hydration method |
Multiple fold increase in skin permeation study with reduced psoriasis plaque symptoms and levels of psoriatic cytokines (TNF-α, IL-17, and IL-22) |
281 |
Cyclosporine |
Topical |
Cationic liposomes by ethanol injection method |
Had shear-thinning behaviour and reduced the symptoms of psoriasis plaque and levels of psoriatic cytokines (TNF-α, IL-17, and IL-22) |
282 |
Zedoary turmeric oil and tretinoin |
Topical |
Ethanol injection |
High drug penetration and drug retention in vitro and significant psoriasis drug-dependent in vivo |
283 |
trans-Retinoic acid and betamethasone |
Topical |
Thin-film hydration |
Nontoxic and time-dependent cellular uptake on HaCaT cells whilst reducing the epidermal thickness and cytokine level (TNF-α and IL-6) in vivo |
284 |
Liposomal spherical nucleic acids (L-SNA) |
Topical |
IL-17A receptor targeting |
Reduced significantly in the PASI score and epidermal thickness on imiquimod (IMQ)-remedied mouse skin, and in the expressions of IL17RA, IL-17C, defensin, beta 4 (DEFB4), TNF-α, and phosphoinositide 3-kinase (PI3K) on psoriatic 3D rafts |
285 |
Bexarotene (retinoid X receptor) |
Topical |
Thin-film hydration |
A significant reversal of psoriasis (reduced scaling, inflammation without any toxicity) on IMQ-induced psoriatic plaque model mice whilst reducing the expression of cytokines (IL-17, IL-23, and IL-2) |
286 |
4 |
Niosomes |
Diacerein |
Topical |
Thin-film hydration |
Prominent skin penetration in epidermal and dermal layers in vitro and found stable at low temperature |
287 |
Acitretin |
Topical |
Thin-film hydration |
Improved ex vivo permeability assay and drug deposition in HaCaT cells |
288 |
Celastrol |
Topical |
Thin-film hydration |
Alleviated erythema and scaling psoriasis symptoms in vivo |
51 |
5 |
Transfersomes |
Tacrolimus |
Transdermal |
Rotary evaporation-sonication |
Higher mean residence time and skin permeation in vivo compared to that of liposomal formulation |
289 |
Methotrexate |
Topical |
The fusion method |
Reduced the thickness score in dose-dependent IMQ-induced psoriasis mouse model with no organ toxicity observed |
290 |
6 |
Ethosomes |
Methotrexate and salicylic acid (MTX-SA) |
Topical |
The cold method |
Decreased in PASI score whilst MTX-SA had more prolonged release than MTX alone |
291 |
Anthralin (dithranol) |
Topical |
Thin-film hydration |
High permeation rate ex vivo with minimised adverse effects after treatment in clinical studies |
292 |
7 |
Nanoemulsions |
Cyclosporine |
Topical |
Phase inversion composition |
High efficacy rate in vitro and improvement in skin hydration in vivo |
293 |
Tacrolimus |
Topical |
Spontaneous emulsification |
Prolonged-release pattern and dermal bioavailability improvement in vitro whilst showed depletion in serum cytokines and improvement in psoriasis symptoms in vivo |
294 |
Methotrexate |
Topical |
Low energy emulsification |
Improved skin permeation and retention in deep skin layers ex vivo with an increase in antipsoriatic efficacy, effective skin retention, and lesser serum and tissue accumulation in vivo |
295 |
Curcumin (natural compound) |
Topical |
Low-energy emulsification |
Multiple fold increase in skin permeation and fast healing in psoriatic activity |
296 |
Imiquimod and curcumin |
Topical |
Low energy emulsification |
Prevented the appearance of psoriasis-like symptoms and slowing down the psoriatic activity |
297 |
5.1 Solid lipid nanoparticles (SLNs)
SLNs is the first generation of lipid nanocarrier systems introduced in the 1990s. It is an advanced drug delivery carrier where the submicron particles (40 to 1000 nm) are lipids that are dispersed in an aqueous surfactant solution.180 The surfactant used for SLNs acts as an emulsifier while the lipids (e.g., fatty acids, steroids, glycerides) are solid at the body and ambient temperature.181 SLNs are commonly formulated by a cold or hot homogenisation method, depending on the drug's thermal stability.182,183 SLNs can also be formulated by high-pressure homogenisation and ultrasonication methods.182,184 SLNs are site-specific, developed to overcome the drawbacks of polymeric nanoparticles and liposomes, such as polymer degradation and cytotoxicity, and drug leakage, and also liposome's phospholipid degradation.185 Their dynamic system also allows modification in formulating the components compared to the liposomes, such as the surfactant can either be ionic or nonionic and is often used with a co-surfactant to reduce the particle size.186 Nevertheless, established studies show that there is a particular type of surfactant and lipid components in SLN formulation which might have influenced their high cytotoxicity level and thus, have less biocompatibility feature such as sodium dodecyl sulphate (surfactant) and stearic acid (lipid).187,188
Within SLN systems, there exists a perfect crystal structure that resembles ‘a wall of brick’ in which drug molecules are encompassed between similar lipid components.189 For this reason, the nanosized particles remain in the solid-state even after administration, which offers a controlled release profile.190 Because of the same reason, SLNs can provide drug protection from external factors by their rigid core matrix.186 They are also possible to be produced on large scale by the high-pressure homogenisation method.62 Despite the basis of SLNs' core matrix that is better suited for hydrophobic-loaded drugs, research has reported for SLNs' capability to entrap and release hydrophilic drugs.191,192 However, since the solid core encapsulated in the lipid system has a perfect crystalline structure, this would lead to inefficient drug loading and possible drug repulsion due to the crystallisation process. Other inevitable factors may also affect SLNs' drug encapsulation in the lipid matrix, such as the melting of lipids, and the miscibility or dispersion of the drug with the lipids.62
5.2 Nanostructured lipid carriers (NLCs)
About two decades ago, researchers observed some limitations in SLNs, such as drug leakage through the matrix during storage and the less efficient drug loading as mentioned previously.62 The limitations led to the invention of NLCs, second-generation lipid carrier systems that are capable of better drug loading efficiency. Within NLCs' system, the drug is encapsulated in the mixture of unsaturated, amorphous, or liquid lipids (oils) to the solid lipids.193 The premise of NLCs' design was also improved to obtain a solidified core with a little-to-no crystalline matrix.183
NLCs also have similar preparation methods to SLNs, namely cold homogenisation, hot homogenisation, and hot emulsification-ultrasonication.183 However, unlike SLNs, the oils in NLCs formulation ensure the expulsion of the loaded drug is minimised during the storage period as they obstruct the recrystallisation process.190 The incorporation of drugs is also improved due to lipid imperfections within their matrix systems.194 Thus, higher drug solubility and a controlled release profile can be achieved by NLCs.62 Currently, NLCs are regarded as a more superior drug carrier than SLNs due to their more excellent formulation and biocompatibility properties. NLCs are also site-specific, and their topical applications improve skin irritation and occlusive properties, as well as skin permeation and retention.195–197
5.3 Nanovesicular systems
Nanovesicular systems (also known as nanovesicles) can typically be distinguished by their unilamellar structures, which have become up-and-coming drug delivery and targeting systems.198 The original vesicles are conventional liposomes before they are adapted into niosomes, transfersomes, and ethosomes, named after their characteristics and compositions.199 They can be assembled per need of their membrane components, and the type of the drug (hydrophobic or hydrophilic),199 which are useful for topical delivery of psoriasis.27
5.3.1 Liposomes. The liposomes are nano-, and micro-sized colloidal multilayer vesicles (50–1000 nm in diameter) consist of an aqueous compartment enclosed by natural or synthetic lipid bilayers.37,200 Liposomes are permeable to water and osmotically sensitive.201 Liposomes may present in multilamellar, small unilamellar, large unilamellar, or multivesicular shapes. Regardless of that, liposomes' size and homogeneity are more essential for drug encapsulation compared to the lamellar numbers.202,203 Liposomes can be prepared conventionally by thin-film hydration, reverse-phase evaporation, detergent dialysis, or solvent injection method.199,204 Further formation steps namely sonication, high-pressure homogenisation or extrusion technique is required to obtain smaller unilamellar vesicles.199,204Several drawbacks associated with phospholipid bilayers of conventional liposomes are revealed in literature such as high-cost production, short half-life, and low solubility.199,200 Besides, the encapsulated drug molecules within liposomes may leak out and fuse because of their less stable surfactants (phospholipids).200 Phospholipids that make up the membrane components of liposomes have a negatively charged phosphate hydrophilic head group with two adjacent fatty acid chains on their tail. Phospholipids are disposed to undergo hydrolysis–oxidation reactions due to several factors: (1) small liposome size and low concentration; (2) the presence of oxidant agents (chemical oxidants and enzymatic oxidants); and (3) modification on fatty acyl chain. The literature also stated that liposome size is an essential parameter for a stable liposome, whilst phospholipids' starting material could cause free radical oxidation.205
Accordingly, some characteristics are modified to improve liposomal formulations for gene delivery application and are pertinent to antipsoriatic activity. The recent shift towards cationic liposomes by adding cationic lipids on the surface of liposomes to form stable complexes has gained a lot of interest for select nucleic acid delivery.206,207 In this regard, liposomes provide some protection to the genes from degradation reactions. The cationic liposomal structures will get entrapped to the large negatively charged DNA fragments, possibly about chromosome size, and, most importantly, can target specific cells or tissues.200
5.3.2 Niosomes. Niosomes are the best alternative to liposomes. They are developed to overcome the low physical stability and expensive production of liposomes.208,209 Niosomes obtained the name because they consist of nonionic surfactants, thus are less toxic to the human's body since they carry no charge.208,210 However, the earliest reported use of niosomes (known as synthetic liposomes) was in cosmetical application, coined by cosmetic giant L’Oréal in the 1970s and 1980s,211,212 before being explored in pharmaceutical applications. Since they are made up of nonionic surfactants, they are less haemolytic and disrupt the cellular surfaces, thus, exhibiting biocompatible properties.213–215 The formation of niosomes is usually with or without cholesterol, in which the latter affords the membrane rigidity and stability due to its charge presence.216,217Different preparation methods of niosomes have been reported including ether injection method, thin-film hydration method, emulsion method, reverse phase evaporation method, and micro fluidisation method, which each could yield different vesicles sizes.209,218,219 It is signified that a favourable niosome of amphiphilic bilayers for high entrapment efficiency can be produced by taking into consideration of the hydrophilic-lipophilic balance (HLB) value of nonionic surfactants between 4 and 8.218,220 The choice of nonionic surfactants that falls within the range is typically amongst Tween, Span, and Brij. Besides, a stable niosome formation for better drug loading can be achieved had long hydrocarbon chains used without a double bond.220 It is also ubiquitous for nonionic surfactants with a high HLB value, notably Tween 20, Tween 60, and Tween 80 to integrate cholesterol for stabilisation due to their imbalance hefty hydrophilic heads and small hydrophobic tails.220
In general, niosomes exhibit similar physicochemical properties, drug delivery studies, and in vivo behaviour with liposomes.210,221 Even so, they have different structural composition bilayer as niosomes exhibit higher chemical, physical, and biological stabilities of the vesicles.221 As a result, niosomal formulations may offer several advantages over liposomes in respect of higher drug entrapment and cheaper industrial manufacturing.209,210,221 However, this interesting nanovesicle is relatively new and young in pharmaceutical applications compared to liposomes.221 The lack of generally recognised as safe (GRAS) components in niosomes (like phospholipids in liposomes) might be one of their drawbacks,212 whilst the aforementioned commercial surfactants (Tween and Span) reportedly exhibit polydispersity feature, which is held as another shortcoming from niosomes.210
5.3.3 Transfersomes. Transfersomes (also known as ultra-deformable liposomes or elastic liposomes),222,223 was designed by Cevc and Blume in 1992.224,225 The main components of transfersomes are phospholipids (similar to liposomes) and edge activators (EA), a single chain surfactant that is also a membrane-softening agent (e.g., Tween 80, Span 80, and sodium cholate).224,226 Novel approaches have been introduced to provide better deformability, but there is no general recipe in formulating the vesicles since their medium suspending composition must be tailored individually to each type of drug and the specific payload.222,224 Besides, the high bilayer adaptability and vesicle stability are the two vital factors in the process of forming transfersomes.224In essence, transfersomes are more deformable and elastic to efficiently penetrate through the small channels in the skin compared to conventional liposomes.226,227 As a result of their self-optimising deformability, the membrane changing flexibility upon reaching and passing through the skin pores can be attained spontaneously as they open up extracellular pathways of the cells.227 EA acts as a permeation enhancer to enable transdermal penetration by destabilising lipid bilayers of the vesicles while enhancing the fluidity of stratum corneum lipid.163,225 Thus, transdermal delivery can be enhanced by avoiding the first-pass effect and be controlled and prolonged the duration of the drug activity. These pharmacokinetic effects allow the efficiency of short half-life drugs and increase the physiological and pharmacological response.228 Amongst the common preparation methods of transfersomes are either thin-film hydration, reverse-phase evaporation, ethanol injection, or freeze–thaw method.226,229 It is generally regarded that transfersomes are superior to liposomes in terms of skin penetration226,227,230 but relatively inferior to ethosomes in terms of deformability.231 However, transfersomes and ethosomes have equivalent skin deposition ability had ethosomes not incorporated another type of alcohol as will be discussed in the next subsection.232
5.3.4 Ethosomes. Ethosomes were developed by Touitou et al. in 1996, acting as another enhanced delivery of active agents.233–235 Ethosomes are composed of phospholipids (similar to liposomes) and ethanol, in which ethanol plays an essential role in efficient topical drug delivery.236,237 Ethosomes have a better penetration rate through the skin, probably due to their high ethanolic content (20–50%). The soft and malleable vesicles are obtained as a result of the coexistence of both phospholipids and ethanol as proved by Touitou et al.238,239 Similar to transfersomes, therapeutic delivery can be administered via transdermal route by ethosomal systems.240 Thus, the vesicles have a higher therapeutic efficacy over liposomes in terms of the greater permeation of the drug's ability as they can squeeze themselves through the skin pores as established by several studies.241–243 A study by Yang et al. showed that ethosomal formulation had a higher quantity and depth of lipophilic drugs to be delivered across the skin (transdermal delivery) for a longer period when compared to liposomes due to their interdependent composition.244The proposed mechanism of ethosome transportation across the skin is well-described by the literature; however, the exact method of how they act as vehicles is not fully understood.237 The underlying mechanism is upon ethanol's polar head group interaction with the lipid regions of the skin to allow the increase of cell membrane lipid fluidity, which results in improved skin penetrability of the ethosomes.237 These ethosomes then permeate the skin, merge with cell membrane lipids, and release the system's drug.245 A recent investigation by Niu and co-workers reported that that the ethanol solution influenced the swelling of the stratum corneum and the increase in the size of keratinocytes, except that the ethosomes were not responsible for the results. The findings also concluded that the ethosomes had better transdermal permeation effects since some of the ethosomes fused to the upper stratum corneum whilst some others travelled and released the drug to the intercellular space during their penetration into the deeper skin layers.246
Amongst popular ethosomal formulations are the classical cold method, the hot method, ethanol injection-sonication, thin-film hydration, and reverse phase-evaporation methods.233 Ethosomal systems can be further classified into their composition as (1) classical ethosomes; (2) binary ethosomes (added with another type of alcohol like isopropyl alcohol); and (3) transethosomes (added with a chemical surfactant or edge activator).233 Several studies published comparative studies between these different types of ethosomes and transfersomes. For instance, upon the comparison between classical ethosomes, binary ethosomes, and transfersomes, it was reported that binary ethosomes have the highest penetration depth and fluorescence intensity but both types of ethosomes have lesser skin deposition than transfersomes.231 Similarly, Zhang et al. concluded that the significant amount of ethanol had influenced the transdermal drug delivery rather than the skin deposition (topical delivery) of ethosomes. Hence, the ethosomal formulations could be adjusted with propylene glycol to prolong the movement of the vesicles and accumulate in the skin.241 On top of that, transethosomes showed the highest drug's incorporation efficiency as opposed to ethosomes and transfersomes, and as such, were more deformable than the two because of the presence of both ethanol and surfactant compositions.232
5.4 Nanoemulsions
Nanoemulsions are isotropic, heterogeneous system, consisting of two immiscible liquids of a sufficient drug's dispersion in nanodroplet sizes.247 The two liquids usually are either water in oil (W/O) or oil in water (O/W) or double emulsion (W/O/W and O/W/O)248 stabilised by amphiphilic surfactants, with mean droplets of <200 nm depending on the phase media.249 The systems have been proven to virtually encapsulate lipophilic active compounds for enhanced skin delivery topically.250 Besides, nanoemulsions are also excellent vehicles to encapsulate essential oils and natural bioactive compounds as stated in the literature and proven in recent studies.251–254 The emulsion technique is versatile, and as such, it can be rendered in spray, gel, cream, and aerosol. Several other reasons also lead to the overwhelming number of research that utilises nanoemulsion methods as drug delivery, such as they are feasible, non-invasive, and cost-effective, especially for high metabolic active drugs.255,256
The choice of surfactants is vital in reducing the surface and interfacial tension between oil and water, which can either be ionic or nonionic.249 Amongst common surfactants or emulsifying agents are Tween® 80, amphiphilic proteins (caseinate), phospholipids (soy lecithin), polysaccharides, and polymers (PEG).257 Generally, nonionic surfactants are regarded as safer than anionic surfactants because they have less local irritation, which is suitable for pharmaceutical applications.258 In addition, they also have a lower CMC value than their ionic counterparts of the same alkyl chain length for a more stable drug delivery system.259,260 Concurrently, a co-surfactant is often needed whereby alcohol groups with C3–C8 chain length like ethylene glycol, propylene glycol, ethanol, and propanol are added to enhance the entropy and stabilise the colloidal system.247 It is interesting to note that, nanoemulsion structure has the closest resemblance of a micelle, out of other lipid nanocarriers, except that the innermost part of nanoemulsions is the oil phase that solubilises hydrophobic drugs. In choosing the oils, different types of triglycerides from long-chain triglycerides (LCT), medium-chain triglycerides (MCT), and short-chain triglycerides (SCT) with potent therapeutic benefits have been utilised as excipients and act as determinants in the bioavailability of the encapsulated API.261 Nevertheless, it is assumed that a more stable O/W formulation could be achieved through a less polar oil base.262
The preparation methods of nanoemulsions can generally be divided into high energy and low energy methods.263 The high-energy method, such as high-pressure homogenisation, produces a robust way of synthesising nanoemulsions by applying excess shear or mechanical device, to break large droplets into nanosized particles.263,264 However, the high-energy method merely produces 1% nanoemulsion whilst 99% of its heat is dissipated off, thus, a repeated series of cycles is required, which is more applicable in large scale production.265 On the other hand, two different types of low-energy methods, namely phase inversion composition (PIC) and phase inversion temperature (PIT) are known to be energy-saving since nanoemulsions are produced by exploiting the system's low interfacial property.264,266,267 The PIC and PIT methods have gained special attention lately and become more favourable methods in which temperature and composition are kept constant, respectively.266 Given the requirements of the low-energy methods, composition variables act as determinants in producing nanodroplets rather than the preparation variables as in the high-energy method.267
Nanoemulsions are non-equilibrium systems with thermodynamically unstable and kinetically stable systems that are sensitive to environmental parameters like pH and temperature.268,269 Consequently, they have a propensity to undergo flocculation, coalescence, and/or Ostwald ripening phenomena that are associated with unstable emulsions.270,271 However, with appropriate optimisation in the selection of their components as well as during formulating preparation, a stable nanoemulsion system can be achieved for drug delivery.
6 Future prospects of lipid-based nanoparticles in pharmaceutical applications
In line with nanotechnology evolution, we witness an overwhelming number of nanotechnology uses in pharmaceutical applications. As a result, the utilisation of nanomaterials into nanotechnology-based drug delivery systems is futuristic, and the market value is projected to skyrocket six-times fold for approximately US$334 billion by 2025 compared to that of over a decade ago.298 Nanomedicine will witness rigorous research progress within the next 20 years as more focus on materials design is driven by clinical needs.299 Therefore, a new paradigm of psoriasis treatments is moving towards this novel therapeutic platform.300,301
Interestingly, lipid-based nanoparticles have garnered special attention in psoriasis treatments because of their adaptability and feasibility approach. Each different type of lipid-based nanoparticle is distinctive since their lipid components as surfactants can be tailored per suitability. This leads to a further novel approach as researchers can broaden their horizon in selecting the lipid components as the starting materials for more natural and greener products, such as cardanol, sugar-based surfactants, glycolipids, and biosurfactants.302–306 However, the Cpp of the lipid surfactants must be acknowledged since the morphology prediction would determine the success of preparing any lipid-based nanoparticles. For example, non-ionic lipid surfactants that have (1/2 < Cpp < 1) would form vesicles (niosomes) in an aqueous medium but other types of non-ionic lipid surfactants with (1/3 < Cpp < 1/2) would form cylindrical micelles (nanoemulsions),171–173 as discussed in Section 5.0.
Moreover, the option of encapsulating the API does not limit to a certain type of lipid-based nanoparticles based on the compilation in Table 1. In this regard, we can see the developments of the same API via different nanocarriers such as methotrexate was successfully encapsulated into SLNs,272 NLCs,276,279 transfersomes,290 ethosomes,291 and nanoemulsions,295 with significant therapeutic profiles. Furthermore, these different types of lipid-based nanoparticles have successfully encapsulated several different antipsoriatic agents or API. For example, liposomes have encapsulated cyclosporine,280,282 psoralens,281 zedoary turmeric oil and tretinoin,283trans-retinoic acid and betamethasone,284 L-SNA,285 and bexarotene.286 Therefore, these discriminations demonstrate that lipid-based nanoparticles are versatile and highly promising drug carriers for antipsoriatic activity. They also have a high potential for clinical studies and thus, to be developed in pharmaceuticals.
An online platform, http://www.ClinicalTrials.gov, serves as the clinical trials database that gathers worldwide research and any clinical studies should be reported there to avoid evidence distortion for clinical practice.307,308 However, based on the database, there is no psoriasis nano-drug yet to be approved for use after clinical studies. The database also shows that there is only one psoriasis nano-drug that is undergoing clinical trial. The study (Identifier: NCT03348462) successfully developed two different anthralin nanoformulations encapsulated into liposomes and ethosomes, which has already completed phase 4 clinical trial and showed higher potent effects through ethosomal systems.292
In the pharmaceutical industry, it was reported that commercialised nano drugs are currently at the first generation of phase II clinical trials carried out by small and medium-sized enterprises (SMEs) before entering the market.298 The tedious procedures of clinical trials and financial constraints have hindered the current progress whilst big pharmaceutical companies are only interested in acquiring these SMEs once these emerging drugs get approved by the FDA.298 Some other challenges to realise in pharmaceuticals include that there is a need for further nanoparticle characterisation profile for safety and toxicity-related issues, as well as a lack of nanotechnology-regulated guidelines for the industry.309,310 It is also noteworthy to mention that current nanomedicine investigation on nano drugs is limited to the evaluation of in vitro and in vivo studies.311 Lastly, the interactions between the tissues and lipid-based nanoparticles should also be deeply explored since the drug delivery systems may exhibit intricate behaviour in the human's body.312 For example, nanoparticles may be deposited in the internal organs and thus interact with other immune cells, which could develop other immune reactions.31 However, the bottom-up approach through the self-assembled lipid nanoparticles could improve the safety measures.31 Besides, the application of state-of-the-art technologies namely artificial intelligence (AI) and machine learning could facilitate the nanotherapeutic delivery via stimulation and modelling processes by understanding both biochemical and physicochemical properties of the nano drugs.313 Therefore, profound knowledge on the cytotoxicity and biological activities of nano-drugs are vital to be further investigated inclusively to providing coherent knowledge on their short-term and long-term effects before being pursued in clinical studies.31,311,312
7 Conclusion
Psoriasis is a skin disease that is affected by several known factors including environmental and genetic, causing cell hyperproliferation. Psoriasis treatments provide a wide array of treatment options, namely topical, systemic nonbiologic, systemic biologics, and phototherapy. Despite the many antipsoriatic drugs with different mechanisms of action, topical treatment serves as the most convenient way to transport the therapeutics across the skin barrier. Lipid-based nanoparticles are promising novel nano delivery systems to improve the API delivery to the target site. Recent works show that different APIs can be encapsulated into different lipid nanocarriers but liposomes and nanoemulsions have the highest number of successful nano-based drugs formulated for psoriasis treatments. Enhanced skin permeation and alleviated psoriasis symptoms are the improvements of antipsoriatic agents in tandem with lipid-based nanoparticles. Besides, the therapeutics also show highly favourable outcomes in alleviating psoriasis lesions as they enhance skin penetration, retention, and prolonged release due to their large surface area at nanoscale size. However, further research and opportunities with other types of lipid-based nanocarriers like niosomes, transfersomes, and ethosomes are needed to explore their tremendous potential in the antipsoriatic application's topical delivery. In general, the production cost of nano drugs is expensive in the pharmaceutical industry. The development of nano drugs is also associated with cytotoxicity and safety issues but could be overcome by an in-depth understanding of their interactions in the human's body. Based on the compilation of the recent works of the API with lipid-based nanocarriers, it is also observed that they exhibit higher drug penetration and bioavailability compared to that of the control variable (conventional treatments without nanocarrier). Therefore, the production of nano drugs is guaranteed success if optimised strategies are taken in nanomedicine applications.
Author contributions
Conceptualization, U. U. M. N., N. A., N. S. and N. S. M. Y.; methodology, U. U. M. N.; writing – original draft preparation, U. U. M. N.; writing – review & editing, N. A., N. S. and N. S. M. Y.; visualization, U. U. M. N.; supervision, N. A. and N. S.; project administration, N. A. and N. S.; funding acquisition, N. A.; all authors have read and agreed to the published version of the manuscript.
Conflicts of interest
There are no conflicts to declare.
Acknowledgements
The authors gratefully acknowledge the Ministry of Higher Education Malaysia (MOHE) for the research project financial support through the Fundamental Research Grant Scheme (FP037-2019A) and the University of Malaya.
References
- J. Takeshita, S. Grewal, S. M. Langan, N. N. Mehta, A. Ogdie, A. S. Van Voorhees and J. M. Gelfand, J. Am. Acad. Dermatol., 2017, 76, 377–390 CrossRef PubMed.
- R. Parisi, I. Y. Iskandar, E. Kontopantelis, M. Augustin, C. E. Griffiths and D. M. Ashcroft, Br. Med. J., 2020, 369, m1590 CrossRef PubMed.
- A. W. Armstrong and C. Read, J. Am. Med. Assoc., 2020, 323, 1945–1960 CrossRef CAS PubMed.
- B. Elewski, A. F. Alexis, M. Lebwohl, L. S. Gold, D. Pariser, J. D. Rosso and G. Yosipovitch, J. Eur. Acad. Dermatol. Venereol., 2019, 33, 1465–1476 CrossRef CAS PubMed.
- Z. Yiu, R. Warren and A. Menter, in Handbook of Psoriasis and Psoriatic Arthritis, ed. R. Warren and A. Menter, Adis, Cham, 2016 Search PubMed.
- S. K. Ippagunta, R. Gangwar, D. Finkelstein, P. Vogel, S. Pelletier, S. Gingras, V. Redecke and H. Häcker, Proc. Natl. Acad. Sci. U.S.A., 2016, 113, E6162–E6171 CrossRef CAS PubMed.
- W. Masson, M. Lobo and G. Molinero, Adv. Ther., 2020, 37, 2017 CrossRef PubMed.
- A. Rendon and K. Schäkel, Int. J. Mol. Sci., 2019, 20, 1475 CrossRef CAS PubMed.
- J. M. Fernández-Armenteros, X. Gómez-Arbonés, M. Buti-Solé, A. Betriu-Bars, V. Sanmartin-Novell, M. Ortega-Bravo, M. Martínez-Alonso and J. M. Casanova-Seuma, Actas Dermo-Sifiliográficas, 2019, 110, 385–392 CrossRef PubMed.
- E. Ogawa, Y. Sato, A. Minagawa and R. Okuyama, J. Dermatol., 2018, 45, 264–272 CrossRef CAS PubMed.
- C.-H. Lin, S.-H. Chang and J.-Y. Fang, Curr. Drug Ther., 2016, 11, 21–40 CrossRef CAS.
- R. Parisi, D. P. Symmons, C. E. Griffiths and D. M. Ashcroft, J. Invest. Dermatol., 2013, 133, 377–385 CrossRef CAS PubMed.
- M. Rademaker, K. Agnew, N. Anagnostou, M. Andrews, K. Armour, C. Baker, P. Foley, K. Gebauer, M. Gupta and G. Marshman, Australas. J. Dermatol., 2019, 60, 91–98 CrossRef PubMed.
- A. Menter, K. M. Cordoro, D. M. R. Davis, D. Kroshinsky, A. S. Paller, A. W. Armstrong, C. Connor, B. E. Elewski, J. M. Gelfand, K. B. Gordon, A. B. Gottlieb, D. H. Kaplan, A. Kavanaugh, M. Kiselica, D. Kivelevitch, N. J. Korman, M. Lebwohl, C. L. Leonardi, J. Lichten, H. W. Lim, N. N. Mehta, S. L. Parra, A. L. Pathy, E. A. Farley Prater, R. N. Rupani, M. Siegel, B. Stoff, B. E. Strober, E. B. Wong, J. J. Wu, V. Hariharan and C. A. Elmets, J. Am. Acad. Dermatol., 2020, 82, 161–201 CrossRef PubMed.
- D. M. Balak and E. Hajdarbegovic, Psoriasis, 2017, 7, 87–94 CrossRef CAS PubMed.
- N. J. Korman, Br. J. Dermatol., 2020, 182, 840–848 CrossRef CAS PubMed.
- S. González-Parra and E. Daudén, Actas Dermo-Sifiliográficas, 2019, 110, 12–19 CrossRef PubMed.
- F. M. Sahi, A. Masood, N. A. Danawar, A. Mekaiel and B. H. Malik, Cureus, 2020, 12(8), e9708 Search PubMed.
- C. Fink, C. Alt, L. Uhlmann, C. Klose, A. Enk and H. A. Haenssle, Br. J. Dermatol., 2019, 180, 390–396 CrossRef CAS PubMed.
- D. Hägg, A. Sundström, M. Eriksson and M. Schmitt-Egenolf, Am. J. Clin. Dermatol., 2017, 18, 583–590 CrossRef PubMed.
- V. K. Rapalli, G. Singhvi, S. K. Dubey, G. Gupta, D. K. Chellappan and K. Dua, Biomed. Pharmacother., 2018, 106, 707–713 CrossRef CAS PubMed.
- G. Singhvi, S. Hejmady, V. K. Rapalli, S. K. Dubey and S. Dubey, in Delivery of Drugs, Elsevier, 2020, pp. 75–96 Search PubMed.
- M. Bhat, S. Pukale, S. Singh, A. Mittal and D. Chitkara, J. Drug Deliv. Sci. Technol., 2021, 62, 102328 CrossRef CAS.
- A. W. Armstrong, L. Puig, A. Joshi, M. Skup, D. Williams, J. Li, K. A. Betts and M. Augustin, JAMA Dermatol., 2020, 156, 258–269 CrossRef PubMed.
- P. Rider, Y. Carmi and I. Cohen, Int. J. Cell Biol., 2016, 2016, 9259646 Search PubMed.
- A. Menter, B. E. Strober, D. H. Kaplan, D. Kivelevitch, E. F. Prater, B. Stoff, A. W. Armstrong, C. Connor, K. M. Cordoro, D. M. R. Davis, B. E. Elewski, J. M. Gelfand, K. B. Gordon, A. B. Gottlieb, A. Kavanaugh, M. Kiselica, N. J. Korman, D. Kroshinsky, M. Lebwohl, C. L. Leonardi, J. Lichten, H. W. Lim, N. N. Mehta, A. S. Paller, S. L. Parra, A. L. Pathy, R. N. Rupani, M. Siegel, E. B. Wong, J. J. Wu, V. Hariharan and C. A. Elmets, J. Am. Acad. Dermatol., 2019, 80, 1029–1072 CrossRef PubMed.
- M. Pradhan, A. Alexander, M. R. Singh, D. Singh, S. Saraf and S. Saraf, Biomed. Pharmacother., 2018, 107, 447–463 CrossRef CAS PubMed.
- A. R. Fernandes, C. Martins-Gomes, A. Santini, A. M. Silva and E. B. Souto, in Design of Nanostructures for Versatile Therapeutic Applications, ed. A. M. Grumezescu, William Andrew Publishing, 2018, pp. 379–406 Search PubMed.
- M. R. Gigliobianco, C. Casadidio, R. Censi and P. Di Martino, Pharmaceutics, 2018, 10, 134 CrossRef CAS PubMed.
- W. Kaialy and M. Al Shafiee, Adv. Colloid Interface Sci., 2016, 228, 71–91 CrossRef CAS PubMed.
- W. Lu, J. Yao, X. Zhu and Y. Qi, Biomed. Pharmacother., 2021, 134, 111103 CrossRef CAS PubMed.
- X. Zhang, Z. Li, J. Gao, Z. Wang, X. Gao, N. Liu, M. Li, H. Zhang and A. Zheng, Molecules, 2020, 25, 1080 CrossRef CAS PubMed.
- M. Swierczewska, R. M. Crist and S. E. McNeil, in Characterization of Nanoparticles Intended for Drug Delivery, Humana Press, New York, NY, 2018, pp. 3–16 Search PubMed.
- S. L. Jyothi, K. L. Krishna, V. K. Ameena Shirin, R. Sankar, K. Pramod and H. V. Gangadharappa, J. Drug Deliv. Sci. Technol., 2021, 62, 102364 CrossRef CAS.
- N. Vincent, D. D. Ramya and H. B. Vedha, Dermatol. Rep., 2014, 6, 5451 Search PubMed.
- T. W. Prow, J. E. Grice, L. L. Lin, R. Faye, M. Butler, W. Becker, E. M. T. Wurm, C. Yoong, T. A. Robertson, H. P. Soyer and M. S. Roberts, Adv. Drug Deliv. Rev., 2011, 63, 470–491 CrossRef CAS PubMed.
- D. Medina-Cruz, B. Saleh, A. Vernet-Crua, A. Ajo, A. K. Roy and T. J. Webster, in Wound Healing, Tissue Repair, and Regeneration in Diabetes, ed. D. Bagchi, A. Das and S. Roy, Academic Press, 2020, pp. 439–488 Search PubMed.
- C. Su, Y. Liu, R. Li, W. Wu, J. P. Fawcett and J. Gu, Adv. Drug Deliv. Rev., 2019, 143, 97–114 CrossRef CAS PubMed.
- T. Ramezanli, B. E. Kilfoyle, Z. Zhang and B. B. Michniak-Kohn, Int. J. Pharm., 2017, 516, 196–203 CrossRef CAS PubMed.
- A. L. Savian, D. Rodrigues, J. Weber, R. F. Ribeiro, M. H. Motta, S. R. Schaffazick, A. I. H. Adams, D. F. de Andrade, R. C. R. Beck and C. B. da Silva, Mater. Sci. Eng. C, 2015, 46, 69–76 CrossRef CAS PubMed.
- P. K. Tripathi, B. Gorain, H. Choudhury, A. Srivastava and P. Kesharwani, Heliyon, 2019, 5, e01343 CrossRef PubMed.
- M. Lapteva, V. Santer, K. Mondon, I. Patmanidis, G. Chiriano, L. Scapozza, R. Gurny, M. Möller and Y. N. Kalia, J. Control. Release, 2014, 196, 9–18 CrossRef CAS PubMed.
- K.-L. Mao, Z.-L. Fan, J.-D. Yuan, P.-P. Chen, J.-J. Yang, J. Xu, D.-L. ZhuGe, B.-H. Jin, Q.-Y. Zhu, B.-X. Shen, Y. Sohawon, Y.-Z. Zhao and H.-L. Xu, Colloids Surf. B Biointerfaces, 2017, 160, 704–714 CrossRef CAS PubMed.
- S. A. Fereig, G. M. El-Zaafarany, M. G. Arafa and M. M. A. Abdel-Mottaleb, Carbohydr. Polym., 2021, 268, 118238 CrossRef CAS PubMed.
- K. Yadav, A. Soni, D. Singh and M. R. Singh, Prog. Biomater., 2021, 10, 1–17 CrossRef CAS PubMed.
- S. Sur, A. Rathore, V. Dave, K. R. Reddy, R. S. Chouhan and V. Sadhu, Nano-Struct. Nano-Objects, 2019, 20, 100397 CrossRef CAS.
- M. T. C. P. Bernardes, S. B. N. Agostini, G. R. Pereira, L. P. da Silva, J. B. da Silva, M. L. Bruschi, R. D. Novaes and F. C. Carvalho, Eur. J. Pharm. Sci., 2021, 165, 105956 CrossRef CAS PubMed.
- D. Limón, K. Talló Domínguez, M. L. Garduño-Ramírez, B. Andrade, A. C. Calpena and L. Pérez-García, Colloids Surf. B Biointerfaces, 2019, 181, 657–670 CrossRef PubMed.
- Q. Li, F. Li, X. Qi, F. Wei, H. Chen and T. Wang, Colloids Surf. B Biointerfaces, 2020, 195, 111246 CrossRef CAS PubMed.
- S. Kumar, M. Prasad and R. Rao, Mater. Sci. Eng. C, 2021, 119, 111605 CrossRef CAS PubMed.
- S. Meng, L. Sun, L. Wang, Z. Lin, Z. Liu, L. Xi, Z. Wang and Y. Zheng, Colloids Surf. B Biointerfaces, 2019, 182, 110352 CrossRef CAS PubMed.
- S. Jacob, A. B. Nair, J. Shah, N. Sreeharsha, S. Gupta and P. Shinu, Pharmaceutics, 2021, 13, 357 CrossRef CAS PubMed.
- X. Li, J. F. Lovell, J. Yoon and X. Chen, Nat. Rev. Clin. Oncol., 2020, 17, 657–674 CrossRef PubMed.
- G. R. Nirmal, Z.-C. Lin, M.-J. Tsai, S.-C. Yang, A. Alalaiwe and J.-Y. Fang, J. Controlled Release, 2021, 333, 487–499 CrossRef CAS PubMed.
- J. Chen, Z. Zeng, L. Huang, S. Luo, J. Dong, F. H. Zhou, K. Zhou, L. Wang and L. Kang, Am. J. Transl. Res., 2020, 12, 3089–3115 CAS.
- H.-Q. Liu, Y.-M. Wang, W.-F. Li, C. Li, Z.-H. Jiang, J. Bao, J.-F. Wei, H.-T. Jin and A.-P. Wang, Cell. Physiol. Biochem., 2017, 44, 200–214 CrossRef PubMed.
- P. R. Ebani, L. Stefanello, B. Luiza Kuhn, C. Piccinin Frizzo, T. Augusto Lima Burgo, C. Luisa Kloster and M. Antonio Villetti, J. Mol. Liq., 2021, 336, 116874 CrossRef.
- R. Kumar, in Nanocarriers for Drug Delivery, ed. S. S. Mohapatra, S. Ranjan, N. Dasgupta, R. K. Mishra and S. Thomas, Elsevier, 2019, pp. 249–284 Search PubMed.
- M. Pradhan, D. Singh and M. R. Singh, J. Controlled Release, 2013, 170, 380–395 CrossRef CAS PubMed.
- M. Gupta, U. Agrawal and S. P. Vyas, Expet Opin. Drug Deliv., 2012, 9, 783–804 CrossRef CAS PubMed.
- B. García-Pinel, C. Porras-Alcalá, A. Ortega-Rodríguez, F. Sarabia, J. Prados, C. Melguizo and J. M. López-Romero, Nanomaterials, 2019, 9, 638 CrossRef PubMed.
- P. Ghasemiyeh and S. Mohammadi-Samani, Res. Pharm. Sci, 2018, 13, 288–303 CrossRef PubMed.
- S.-Y. Chuang, C.-H. Lin, T.-H. Huang and J.-Y. Fang, Nanomaterials, 2018, 8, 42 CrossRef PubMed.
- C. Albanesi, in Clinical Immunology, Elsevier, 2019, pp. 871–878. e1 Search PubMed.
- B. Lukasch, H. Westerdahl, M. Strandh, H. Winkler, Y. Moodley, F. Knauer and H. Hoi, PeerJ, 2017, 5, e3679 CrossRef PubMed.
- M. Wieczorek, E. T. Abualrous, J. Sticht, M. Álvaro-Benito, S. Stolzenberg, F. Noé and C. Freund, Front. Immunol., 2017, 8, 292 Search PubMed.
- V. Kumar, A. K. Abbas and J. C. Aster, Robbins Basic Pathology, Elsevier, Philadelphia, PA, 10th edn, 2018 Search PubMed.
- J. B. Zabriskie, Essential clinical immunology, Cambridge University Press, 2009 Search PubMed.
- M. P. Schön, Front. Immunol., 2019, 10, 1764 CrossRef PubMed.
- J. M. Hugh and J. M. Weinberg, Cutis, 2018, 102, 6–12 Search PubMed.
- H. R. Hampton and T. Chtanova, Front. Immunol., 2019, 10, 1168 CrossRef CAS PubMed.
- A. Wang and Y. Bai, J. Dermatol., 2020, 47, 104–113 CrossRef PubMed.
- C. de Jesús-Gil, L. Sans-de SanNicolàs, I. García-Jiménez, M. Ferran, A. Celada, A. Chiriac, R. M. Pujol and L. F. Santamaria-Babí, Front. Immunol., 2021, 12, 524 Search PubMed.
- V. R. Khairutdinov, A. F. Mikhailichenko, I. E. Belousova, E. Sh. Kuligina, A. V. Samtsov and E. N. Imyanitov, An. Bras. Dermatol., 2017, 92, 41–44 CrossRef PubMed.
- B. Polese, H. Zhang, B. Thurairajah and I. L. King, Front. Immunol., 2020, 11, 242 CrossRef CAS PubMed.
- B. Wawrzycki, A. Pietrzak, E. Grywalska, D. Krasowska, G. Chodorowska and J. Roliński, Arch. Immunol. Ther. Exp., 2019, 67, 103–108 CrossRef CAS PubMed.
- N. C. Brembilla, L. Senra and W.-H. Boehncke, Front. Immunol., 2018, 9, 1682 CrossRef PubMed.
- M. Tampa, M.-I. Sarbu, M.-I. Mitran, C.-I. Mitran, C. Matei and S.-R. Georgescu, Dis. Markers, 2018, 2018, 5823684 Search PubMed.
- A. M. Martins, A. Ascenso, H. M. Ribeiro and J. Marto, Mol. Neurobiol., 2020, 57, 2391–2419 CrossRef CAS PubMed.
- G. P. Thami and R. Sarkar, Clin. Exp. Dermatol., 2002, 27, 99–103 CrossRef PubMed.
- J. A. Zeichner, J. Clin. Aesthetic Dermatol., 2010, 3, 37–40 Search PubMed.
- A. Bhatia, B. Singh, B. Amarji, P. Negi, A. Shukla and O. P. Katare, Int. J. Dermatol., 2011, 50, 1246–1248 CrossRef PubMed.
- S. Sekhon, C. Jeon, M. Nakamura, L. Afifi, D. Yan, J. J. Wu, W. Liao and T. Bhutani, J. Dermatol. Treat., 2018, 29, 230–232 CrossRef CAS PubMed.
- W. R. Heymann, J. Am. Acad. Dermatol., 2019, 80, 56–57 CrossRef PubMed.
- K. Blakely and M. Gooderham, Psoriasis Auckl. NZ, 2016, 6, 33–40 CAS.
- H. Myśliwiec, P. Myśliwiec, A. Baran and I. Flisiak, Adv. Med. Sci., 2016, 61, 207–211 Search PubMed.
- K. A. Hollywood, C. L. Winder, W. B. Dunn, Y. Xu, D. Broadhurst, C. E. M. Griffiths and R. Goodacre, Mol. Biosyst., 2015, 11, 2198–2209 RSC.
- C. Mo, D. Shetti and K. Wei, Molecules, 2019, 24, 2727 CrossRef CAS PubMed.
- T. Benezeder, C. Painsi, V. Patra, S. Dey, M. Holcmann, B. Lange-Asschenfeldt, M. Sibilia and P. Wolf, eLife, 2020, 9, e56991 CrossRef CAS PubMed.
- J. Holstein, B. Fehrenbacher, J. Brück, E. Müller-Hermelink, I. Schäfer, M. Carevic, B. Schittek, M. Schaller, K. Ghoreschi and F. C. Eberle, J. Dermatol. Sci., 2017, 87, 236–245 CrossRef CAS PubMed.
- V. Kadian, S. Kumar, K. Saini, V. Kakkar and R. Rao, Curr. Drug Res. Rev., 2020, 12, 82–96 CrossRef CAS PubMed.
- M. S. Heath, D. R. Sahni, Z. A. Curry and S. R. Feldman, Expert Opin. Drug Metab. Toxicol., 2018, 14, 919–927 CrossRef CAS PubMed.
- İ. Erol, N. Ü. Okur, D. Orak, H. Sipahi, A. Aydın and Ö. Özer, Pharm. Dev. Technol., 2020, 25, 909–918 CrossRef PubMed.
- E. L. Roux and H. Frow, Prescriber, 2020, 31, 9–17 CrossRef.
- E. Tanghetti, M. Lebwohl and L. Stein Gold, J. Drugs Dermatol. JDD, 2018, 17, 1280–1287 Search PubMed.
- L. S. Gold, M. G. Lebwohl, J. L. Sugarman, D. M. Pariser, T. Lin, G. Martin, R. Pillai, R. Israel and T. Ramakrishna, J. Am. Acad. Dermatol., 2018, 79, 287–293 CrossRef CAS PubMed.
- G. Micali, A. E. Verzì, G. Giuffrida, E. Panebianco, M. L. Musumeci and F. Lacarrubba, Clin. Cosmet. Investig. Dermatol., 2019, 12, 953–959 CrossRef CAS PubMed.
- J. F. Merola, A. Qureshi and M. E. Husni, Dermatol. Ther., 2018, 31, e12589 Search PubMed.
- L. Guenther, C. Lynde and Y. Poulin, J. Cutan. Med. Surg., 2019, 23, 27S–34S CrossRef CAS PubMed.
- S. Jindal, R. Awasthi, D. Singhare and G. T. Kulkarni, Med. Hypotheses, 2020, 142, 109838 CrossRef CAS PubMed.
- Z. Yin, J. Xu and D. Luo, J. Biomed. Res., 2011, 25, 385–391 CrossRef CAS PubMed.
- X. Huang and B. Xu, Dermatology, 2015, 231, 41–49 CrossRef CAS PubMed.
- R. Torsekar and M. M. Gautam, Indian Dermatol. Online J., 2017, 8, 235 CrossRef CAS PubMed.
- C. Stull, S. Grossman and G. Yosipovitch, Am. J. Clin. Dermatol., 2016, 17, 617–624 CrossRef PubMed.
- S. Baboota, M. S. Alam, S. Sharma, J. K. Sahni, A. Kumar and J. Ali, Int. J. Pharm. Investig., 2011, 1, 139–147 CrossRef CAS PubMed.
- C. L. Carroll, J. Clarke, F. Camacho, R. Balkrishnan and S. R. Feldman, Arch. Dermatol., 2005, 141, 43–46 CAS.
- A. Tosti, B. M. Piraccini, N. Cameli, F. Kokely, C. Plozzer, G. E. Cannata and C. Benelli, Br. J. Dermatol., 1998, 139, 655–659 CrossRef CAS PubMed.
- M. S. Al-Dhubaibi, Int. J. Health Sci., 2018, 12, 33 Search PubMed.
- A. Filoni, M. Vestita, M. Congedo, G. Giudice, S. Tafuri and D. Bonamonte, Medicine, 2018, 97, e11185 CrossRef CAS PubMed.
- K. Satake, T. Amano and T. Okamoto, Sci. Rep., 2019, 9, 16322 CrossRef PubMed.
- S. Gabros, T. A. Nessel and P. M. Zito, in StatPearls, StatPearls Publishing, Treasure Island (FL), 2021 Search PubMed.
- E. C. Kleyn, E. Morsman, L. Griffin, J. J. Wu, P. Cm van de Kerkhof, W. Gulliver, J. M. van der Walt and L. Iversen, J. Dermatol. Treat., 2019, 30, 311–319 CrossRef PubMed.
- T. Frantz, E. G. Wright, E. A. Balogh, A. Cline, A. L. Adler-Neal and S. R. Feldman, Children, 2019, 6, 125 CrossRef PubMed.
- E. Choi, N. S. Chandran and C. Tan, Singapore Med. J., 2020, 61(3), 149 CrossRef PubMed.
- S. Y. Song, S.-Y. Jung and E. Kim, J. Dermatol. Treat., 2019, 30, 245–250 CrossRef PubMed.
- G. Martin, M. Young and L. Aldredge, J. Clin. Aesthetic Dermatol., 2019, 12, 13–26 Search PubMed.
- A. Menter, J. M. Gelfand, C. Connor, A. W. Armstrong, K. M. Cordoro, D. M. R. Davis, B. E. Elewski, K. B. Gordon, A. B. Gottlieb, D. H. Kaplan, A. Kavanaugh, M. Kiselica, D. Kivelevitch, N. J. Korman, D. Kroshinsky, M. Lebwohl, C. L. Leonardi, J. Lichten, H. W. Lim, N. N. Mehta, A. S. Paller, S. L. Parra, A. L. Pathy, E. F. Prater, R. S. Rahimi, R. N. Rupani, M. Siegel, B. Stoff, B. E. Strober, E. B. Tapper, E. B. Wong, J. J. Wu, V. Hariharan and C. A. Elmets, J. Am. Acad. Dermatol., 2020, 82, 1445–1486 CrossRef CAS PubMed.
- T. Elango, A. Thirupathi, S. Subramanian, P. Ethiraj, H. Dayalan and P. Gnanaraj, Clin. Exp. Med., 2017, 17, 371–381 CrossRef CAS PubMed.
- C. A. da Silva, K. Von Kossel, M. Leszczynski, T. Melnik and R. Riera, Cochrane Database Syst. Rev., 2019, 2019, CD010498 Search PubMed.
- J. J. Wu, A. Guérin, M. Sundaram, K. Dea, M. Cloutier and P. Mulani, J. Am. Acad. Dermatol., 2017, 76, 81–90 CrossRef CAS PubMed.
- C. W. Choi, B. R. Kim, J. Ohn and S. W. Youn, Ann. Dermatol., 2017, 29, 55–60 CrossRef CAS PubMed.
- E. Baskan, S. Yazici, S. Tunali and H. Saricaoglu, J. Dermatol. Treat., 2016, 27, 328–331 CrossRef PubMed.
- V. Di Lernia, L. Stingeni, V. Boccaletti, P. G. Calzavara Pinton, C. Guarneri, A. Belloni Fortina, M. Panzone, M. Corazza, I. Neri, S. Cambiaghi, C. Lasagni, E. Ficarelli and P. Gisondi, J. Dermatol. Treat., 2016, 27, 395–398 CrossRef CAS PubMed.
- S. Dogra, R. Mahajan, T. Narang and S. Handa, J. Dermatol. Treat., 2017, 28, 18–20 CrossRef CAS PubMed.
- B. R. Kim, S. Yang, E. J. Doh, C. W. Choi and S. W. Youn, Ann. Dermatol., 2018, 30, 143–149 CrossRef CAS PubMed.
- C. J. Oh, K. M. Das and A. B. Gottlieb, J. Am. Acad. Dermatol., 2000, 42, 829–830 CrossRef CAS PubMed.
- M. Frleta, S. Siebert and I. B. McInnes, Curr. Rheumatol. Rep., 2014, 16, 414 CrossRef PubMed.
- J. E. Hawkes, B. Y. Yan, T. C. Chan and J. G. Krueger, J. Immunol., 2018, 201, 1605–1613 CrossRef CAS PubMed.
- K. Godse, Indian J. Dermatol., 2017, 62, 195–199 CrossRef PubMed.
- M. Shirley and L. J. Scott, Drugs, 2016, 76, 1135–1145 CrossRef PubMed.
- A. Blauvelt, Expert Opin. Drug Saf., 2016, 15, 1413–1420 CrossRef CAS PubMed.
- R. Reszke and J. C. Szepietowski, Immunotherapy, 2017, 9, 229–238 CrossRef CAS PubMed.
- P. Mease and I. B. McInnes, Rheumatol. Ther., 2016, 3, 5–29 CrossRef PubMed.
- A. Gottlieb, J. Sullivan, M. van Doorn, A. Kubanov, R. You, A. Parneix, S. Hugot and M. Milutinovic, J. Am. Acad. Dermatol., 2017, 76, 70–80 CrossRef CAS PubMed.
- A. Johar, S. Thevarajah, A. Heng, L. C. Chan, C. C. Ch’ng, N. A. Mohd Safdar, P. Muniandy, T. Taib, W. C. Tan and K. E. Tey, Dermatol. Res. Pract., 2019, 2019, 8923168 Search PubMed.
- A. Egeberg, J. P. Thyssen, J. J. Wu and L. Skov, Br. J. Dermatol., 2019, 180, 116–121 CrossRef CAS PubMed.
- J. J. Wu, S. R. Feldman, S. Rastogi, B. Menges, M. Lingohr-Smith and J. Lin, J. Dermatol. Treat., 2018, 29, 769–774 CrossRef PubMed.
- M. Lebwohl, R. Rivera-Oyola and D. F. Murrell, J. Am. Acad. Dermatol., 2020, 82, 1217–1218 CrossRef CAS PubMed.
- P. C. M. van de Kerkhof and F. R. de Gruijl, J. Eur. Acad. Dermatol. Venereol., 2020, 34, 926–931 CrossRef CAS PubMed.
- K. Hyde, L. A. Cardwell, R. Stotts and S. R. Feldman, Am. J. Pharm. Benefits, 2018, 10, 18–21 Search PubMed.
- C. A. Elmets, H. W. Lim, B. Stoff, C. Connor, K. M. Cordoro, M. Lebwohl, A. W. Armstrong, D. M. R. Davis, B. E. Elewski, J. M. Gelfand, K. B. Gordon, A. B. Gottlieb, D. H. Kaplan, A. Kavanaugh, M. Kiselica, D. Kivelevitch, N. J. Korman, D. Kroshinsky, C. L. Leonardi, J. Lichten, N. N. Mehta, A. S. Paller, S. L. Parra, A. L. Pathy, E. A. Farley Prater, R. N. Rupani, M. Siegel, B. E. Strober, E. B. Wong, J. J. Wu, V. Hariharan and A. Menter, J. Am. Acad. Dermatol., 2019, 81, 775–804 CrossRef PubMed.
- J. M. Vassantachart, T. Soleymani and J. J. Wu, J. Drugs Dermatol. JDD, 2016, 15, 995–1000 Search PubMed.
- V. T. Ma, C. S. Katzman, P. L. Palmbos, R. M. Patel, J. E. Gudjonsson and A. S. Alva, Curr. Probl. Cancer Case Rep., 2020, 1, 100004 Search PubMed.
- A. Ighani, A. C. R. Partridge, N. H. Shear, C. Lynde, W. P. Gulliver, C. Sibbald and P. Fleming, J. Cutan. Med. Surg., 2019, 23, 204–221 CrossRef PubMed.
- P. Zhang and M. X. Wu, Lasers Med. Sci., 2018, 33, 173–180 CrossRef PubMed.
- S. H. Ibbotson, Front. Med., 2018, 5, 184 CrossRef PubMed.
- A. Morita, J. Dermatol., 2018, 45, 287–292 CrossRef CAS PubMed.
- E. Rácz and E. P. Prens, in Ultraviolet Light in Human Health, Diseases and Environment. Advances in Experimental Medicine and Biology, ed. S. Ahmad, Springer, Cham, 2017, pp. 287–294 Search PubMed.
- V. Ardeleanu, D. S. Radaschin and A. L. Tatu, Exp. Ther. Med., 2020, 20, 52–55 Search PubMed.
- M. Nakamura, B. Farahnik and T. Bhutani, F1000Res., 2016, 5, 1684 Search PubMed.
- S. Das, Int. J. Res. Rev., 2019, 6, 258–263 CAS.
- B. Farahnik, M. Nakamura, R. K. Singh, M. Abrouk, T. H. Zhu, K. M. Lee, M. V. Jose, R. DaLovisio, J. Koo, T. Bhutani and W. Liao, Dermatol. Ther., 2016, 6, 315–324 CrossRef PubMed.
- Y. Ren, X. Song, L. Tan, C. Guo, M. Wang, H. Liu, Z. Cao, Y. Li and C. Peng, Front. Pharmacol., 2020, 11, 571535 CrossRef CAS PubMed.
- J. Koo and M. Nakamura, in Clinical Cases in Phototherapy. Clinical Cases in Dermatology, Springer, Cham, 2017, pp. 13–31 Search PubMed.
- E. S. Chambers and M. Vukmanovic-Stejic, Immunology, 2020, 160, 116–125 CrossRef CAS PubMed.
- S. J. van Rensburg, A. Franken and J. L. D. Plessis, Skin Res. Technol., 2019, 25, 595–605 CrossRef PubMed.
- J. D. Bos and M. M. H. M. Meinardi, Exp. Dermatol., 2000, 9, 165–169 CrossRef CAS PubMed.
- M. B. Murphrey, J. H. Miao and P. M. Zito, in StatPearls, StatPearls Publishing, Treasure Island (FL), 2020 Search PubMed.
- D. J. Moore and A. V. Rawlings, Int. J. Cosmet. Sci., 2017, 39, 366–372 CrossRef CAS PubMed.
- K. Vávrová, A. Kováčik and L. Opálka, Eur. Pharm. J., 2017, 64, 28–35 CrossRef.
- Y. Badhe, R. Gupta and B. Rai, J. Mol. Model., 2019, 25, 140 CrossRef PubMed.
- F. Arriagada and J. Morales, Curr. Pharm. Des., 2019, 25, 455–466 CrossRef CAS PubMed.
- T. Jiang, T. Wang, T. Li, Y. Ma, S. Shen, B. He and R. Mo, ACS Nano, 2018, 12, 9693–9701 CrossRef CAS PubMed.
- N. Tiwari, A. S. Sonzogni and M. Calderón, Nanomed, 2019, 14, 2891–2895 CrossRef CAS PubMed.
- X. Zhou, Y. Hao, L. Yuan, S. Pradhan, K. Shrestha, O. Pradhan, H. Liu and W. Li, Chin. Chem. Lett., 2018, 29, 1713–1724 CrossRef CAS.
- T. Sych, Y. Mély and W. Römer, Philos. Trans. R. Soc. Lond. B Biol. Sci., 2018, 373, 20170117 CrossRef PubMed.
- W. Lu, in Encyclopedia of Nanotechnology, ed. B. Bhushan, Springer Netherlands, Dordrecht, 2012, pp. 2371–2382 Search PubMed.
- H. Su, F. Wang, W. Ran, W. Zhang, W. Dai, H. Wang, C. F. Anderson, Z. Wang, C. Zheng, P. Zhang, Y. Li and H. Cui, Proc. Natl. Acad. Sci. U.S.A., 2020, 117, 4518–4526 CrossRef CAS PubMed.
- S. H. Crayton, A. K. Chen, J. F. Liu, E. M. Higbee-Dempsey, C.-H. Huang, A. Tsourkas and Z. Cheng, in Comprehensive Biomaterials II, ed. P. Ducheyne, Elsevier, Oxford, 2017, pp. 424–466 Search PubMed.
- S. Rana, J. Bhattacharjee, K. C. Barick, G. Verma, P. A. Hassan and J. V. Yakhmi, in Nanostructures for Cancer Therapy, ed. A. Ficai and A. M. Grumezescu, Elsevier, 2017, pp. 177–209 Search PubMed.
- R. A. Khalil and A. A. Zarari, Appl. Surf. Sci., 2014, 318, 85–89 CrossRef CAS.
- D. Lombardo, M. A. Kiselev, S. Magazù and P. Calandra, Adv. Condens. Matter Phys., 2015, 2015, e151683 Search PubMed.
- J. N. Israelachvili, D. J. Mitchell and B. W. Ninham, J. Chem. Soc., Faraday Trans. 2, 1976, 72, 1525–1568 RSC.
- D. Lombardo, P. Calandra, L. Pasqua and S. Magazù, Materials, 2020, 13, 1048 CrossRef CAS PubMed.
- P. Iqbal, J. A. Preece and P. M. Mendes, in Supramolecular Chemistry, American Cancer Society, 2012 Search PubMed.
- Z. Huang, W. Song and X. Chen, Front. Chem., 2020, 8, 380 CrossRef CAS PubMed.
- A. Sikder and S. Ghosh, Mater. Chem. Front., 2019, 3, 2602–2616 RSC.
- L. T. Varma, N. Singh, B. Gorain, H. Choudhury, M. M. Tambuwala, P. Kesharwani and R. Shukla, Curr. Drug Deliv., 2020, 17, 279–291 CrossRef CAS PubMed.
- A. Walduck, P. Sangwan, Q. Anh Vo, J. Ratcliffe, J. White, B. W. Muir and N. Tran, RSC Adv., 2020, 10, 33608–33619 RSC.
- R. H. Müller, K. Mäder and S. Gohla, Eur. J. Pharm. Biopharm., 2000, 50, 161–177 CrossRef.
- N. Naseri, H. Valizadeh and P. Zakeri-Milani, Adv. Pharm. Bull., 2015, 5, 305–313 CrossRef CAS PubMed.
- W. Mehnert and K. Mäder, Adv. Drug Deliv. Rev., 2012, 64, 83–101 CrossRef.
- V. R. Salvi and P. Pawar, J. Drug Deliv. Sci. Technol., 2019, 51, 255–267 CrossRef CAS.
- A. C. Silva, E. González-Mira, M. L. García, M. A. Egea, J. Fonseca, R. Silva, D. Santos, E. B. Souto and D. Ferreira, Colloids Surf. B Biointerfaces, 2011, 86, 158–165 CrossRef CAS PubMed.
- V. Mishra, K. K. Bansal, A. Verma, N. Yadav, S. Thakur, K. Sudhakar and J. M. Rosenholm, Pharmaceutics, 2018, 10, 191 CrossRef CAS PubMed.
- Y. Duan, A. Dhar, C. Patel, M. Khimani, S. Neogi, P. Sharma, N. S. Kumar and R. L. Vekariya, RSC Adv., 2020, 10, 26777–26791 RSC.
- C. D. Pizzol, F. B. Filippin-Monteiro, J. A. S. Restrepo, F. Pittella, A. H. Silva, P. Alves de Souza, A. Machado de Campos and T. B. Creczynski-Pasa, Int. J. Environ. Res. Public. Health, 2014, 11, 8581–8596 CrossRef PubMed.
- A. H. Silva, F. B. Filippin-Monteiro, B. Mattei, B. G. Zanetti-Ramos and T. B. Creczynski-Pasa, Sci. Total Environ., 2012, 432, 382–388 CrossRef CAS PubMed.
- V.-A. Duong, T.-T.-L. Nguyen and H.-J. Maeng, Molecules, 2020, 25, 4781 CrossRef CAS PubMed.
- E. B. Souto, I. Baldim, W. P. Oliveira, R. Rao, N. Yadav, F. M. Gama and S. Mahant, Expet Opin. Drug Deliv., 2020, 17, 357–377 CrossRef CAS PubMed.
- G. Amasya, G. Sandri, A. Onay-Besikci, U. Badilli, C. Caramella, M. C. Bonferoni and N. Tarimci, Curr. Drug Deliv., 2016, 13, 1100–1110 CrossRef CAS PubMed.
- R. A. Khallaf, H. F. Salem and A. Abdelbary, Drug
Deliv., 2016, 23, 3452–3460 CrossRef CAS PubMed.
- G. M. Gelfuso, M. S. Cunha-Filho and T. Gratieri, Ther. Deliv., 2016, 7, 735–737 CrossRef CAS PubMed.
- B. Gaba, M. Fazil, S. Khan, A. Ali, S. Baboota and J. Ali, Bull. Fac. Pharm. Cairo Univ., 2015, 53, 147–159 CrossRef.
- A. Khosa, S. Reddi and R. N. Saha, Biomed. Pharmacother., 2018, 103, 598–613 CrossRef CAS PubMed.
- V. K. Rapalli, V. Kaul, T. Waghule, S. Gorantla, S. Sharma, A. Roy, S. K. Dubey and G. Singhvi, Eur. J. Pharm. Sci., 2020, 152, 105438 CrossRef CAS PubMed.
- S. Vaz, R. Silva, M. H. Amaral, E. Martins, J. M. Sousa Lobo and A. C. Silva, Colloids Surf. B Biointerfaces, 2019, 179, 242–249 CrossRef CAS PubMed.
- S. Wadhwa, V. Garg, M. Gulati, B. Kapoor, S. K. Singh and N. Mittal, in Pharmaceutical Nanotechnology: Basic Protocols, ed. V. Weissig and T. Elbayoumi, Springer, New York, NY, 2019, pp. 1–17 Search PubMed.
- N. Grimaldi, F. Andrade, N. Segovia, L. Ferrer-Tasies, S. Sala, J. Veciana and N. Ventosa, Chem. Soc. Rev., 2016, 45, 6520–6545 RSC.
- H. Daraee, A. Etemadi, M. Kouhi, S. Alimirzalu and A. Akbarzadeh, Artif. Cells Nanomed. Biotechnol., 2016, 44, 381–391 CrossRef CAS PubMed.
- N. Filipczak, J. Pan, S. S. K. Yalamarty and V. P. Torchilin, Adv. Drug Deliv. Rev., 2020, 156, 4–22 CrossRef CAS PubMed.
- E. Elizondo, E. Moreno, I. Cabrera, A. Córdoba, S. Sala, J. Veciana and N. Ventosa, in Progress in Molecular Biology and Translational Science, ed. A. Villaverde, Academic Press, 2011, vol. 104, pp. 1–52 Search PubMed.
- L. Maja, K. Željko and P. Mateja, J. Supercrit. Fluids, 2020, 165, 104984 CrossRef CAS.
- K. S. Ahmed, S. A. Hussein, A. H. Ali, S. A. Korma, Q. Lipeng and C. Jinghua, J. Drug Target., 2019, 27, 742–761 CrossRef CAS PubMed.
- A. Reis and C. M. Spickett, Biochim. Biophys. Acta Biomembr., 2012, 1818, 2374–2387 CrossRef CAS PubMed.
- M. A. Obeid, R. J. Tate, A. B. Mullen and V. A. Ferro, in Lipid Nanocarriers for Drug Targeting, ed. A. M. Grumezescu, William Andrew Publishing, 2018, pp. 313–359 Search PubMed.
- K. Yadav, D. Singh, M. R. Singh and M. Pradhan, Med. Hypotheses, 2020, 145, 110322 CrossRef CAS PubMed.
- P. Bhardwaj, P. Tripathi, R. Gupta and S. Pandey, J. Drug Deliv. Sci. Technol., 2020, 56, 101581 CrossRef CAS.
- S. Chen, S. Hanning, J. Falconer, M. Locke and J. Wen, Eur. J. Pharm. Biopharm., 2019, 144, 18–39 CrossRef CAS PubMed.
- R. Bartelds, M. H. Nematollahi, T. Pols, M. C. A. Stuart, A. Pardakhty, G. Asadikaram and B. Poolman, PloS One, 2018, 13, e0194179 CrossRef PubMed.
- G. M. El Maghraby, M. F. Arafa and E. A. Essa, in Applications of Nanocomposite Materials in Drug Delivery, ed. E. M. Inamuddin, A. M. Asiri and A. Mohammad, Woodhead Publishing, 2018, pp. 469–490 Search PubMed.
- T. G. Singh and N. Sharma, in Nanobiomaterials in Galenic Formulations and Cosmetics, ed. A. M. Grumezescu, William Andrew Publishing, 2016, pp. 149–174 Search PubMed.
- I. Ali, M. R. Shah, S. Yousuf, S. Ahmed, K. Shah and I. Javed, Toxicol. Res., 2018, 7, 771–778 CrossRef CAS PubMed.
- G. P. Kumar and P. Rajeshwarrao, Acta Pharm. Sin. B, 2011, 1, 208–219 CrossRef.
- Y. Zhang, K. Zhang, Z. Wu, T. Guo, B. Ye, M. Lu, J. Zhao, C. Zhu and N. Feng, Int. J. Pharm., 2015, 478, 138–146 CrossRef CAS PubMed.
- R. Liang, L. Chen, W. Yokoyama, P. A. Williams and F. Zhong, J. Agric. Food Chem., 2016, 64, 9180–9188 CrossRef CAS PubMed.
- M. H. Nematollahi, A. Pardakhty, M. Torkzadeh-Mahanai, M. Mehrabani and G. Asadikaram, RSC Adv., 2017, 7, 49463–49472 RSC.
- R. Rajera, K. Nagpal, S. K. Singh and D. N. Mishra, Biol. Pharm. Bull., 2011, 34, 945–953 CrossRef CAS PubMed.
- P. L. Yeo, C. L. Lim, S. M. Chye, A. P. K. Ling and R. Y. Koh, Asian Biomed., 2018, 11, 301–314 CrossRef.
- B. Lu, Y. Huang, Z. Chen, J. Ye, H. Xu, W. Chen and X. Long, Molecules, 2019, 24, 2322 CrossRef CAS PubMed.
- R. Muzzalupo and E. Mazzotta, Expet Opin. Drug Deliv., 2019, 16, 1145–1147 CrossRef CAS PubMed.
- J. Chen, W.-L. Lu, W. Gu, S.-S. Lu, Z.-P. Chen and B.-C. Cai, Expet Opin. Drug Deliv., 2013, 10, 845–856 CrossRef CAS PubMed.
- B. Piumitali, U. Neeraj and J. Jyotivardhan, Int. J. Nanosci., 2020, 19, 1950033 CrossRef CAS.
- G. Cevc and A. Chopra, in Percutaneous penetration enhancers chemical methods in penetration enhancement, Springer, 2016, pp. 39–59 Search PubMed.
- P.-S. Wu, Y.-S. Li, Y.-C. Kuo, S.-J. J. Tsai and C.-C. Lin, Molecules, 2019, 24, 600 CrossRef PubMed.
- S. A. T. Opatha, V. Titapiwatanakun and R. Chutoprapat, Pharmaceutics, 2020, 12, 855 CrossRef CAS PubMed.
- S. Rai, V. Pandey and G. Rai, Nano Rev. Exp., 2017, 8, 1325708 CrossRef PubMed.
- P. Chaurasiya, E. Ganju, N. Upmanyu, S. K. Ray and P. Jain, J. Drug Deliv. Ther., 2019, 9, 279–285 CrossRef CAS.
- P. Chauhan and B. K. Tyagi, J. Drug Deliv. Ther., 2018, 8, 162–168 CrossRef.
- S. Jain, P. Jain, R. B. Umamaheshwari and N. K. Jain, Drug Dev. Ind. Pharm., 2003, 29, 1013–1026 CrossRef CAS PubMed.
- J.-P. Zhang, Y.-H. Wei, Y. Zhou, Y.-Q. Li and X.-A. Wu, Arch. Pharm. Res., 2012, 35, 109–117 CrossRef CAS PubMed.
- A. Ascenso, S. Raposo, C. Batista, P. Cardoso, T. Mendes, F. G. Praça, M. V. L. B. Bentley and S. Simões, Int. J. Nanomedicine, 2015, 10, 5837–5851 CrossRef CAS PubMed.
- I. M. Abdulbaqi, Y. Darwis, N. A. K. Khan, R. A. Assi and A. A. Khan, Int. J. Nanomedicine, 2016, 11, 2279–2304 CrossRef CAS PubMed.
- M. M. A. Elsayed, O. Y. Abdallah, V. F. Naggar and N. M. Khalafallah, Int. J. Pharm., 2006, 322, 60–66 CrossRef CAS PubMed.
- E. Touitou, B. Godin and C. Weiss, Drug Dev. Res., 2000, 50, 406–415 CrossRef CAS.
- D. Aggarwal and U. Nautiyal, Int. J. Pharm. Med. Res., 2016, 4, 354–363 Search PubMed.
- P. Verma and K. Pathak, J. Adv. Pharm. Technol. Res., 2010, 1, 274–282 CrossRef CAS PubMed.
- D. Mishra, N. Balekar, V. Dhote and P. Mishra, 2017.
- E. Touitou, N. Dayan, L. Bergelson, B. Godin and M. Eliaz, J. Control. Release, 2000, 65, 403–418 CrossRef CAS PubMed.
- B. Godin and E. Touitou, Crit. Rev. Ther. Drug Carr. Syst., 2003, 20, 63–102 CrossRef CAS PubMed.
- L.-N. Shen, Y.-T. Zhang, Q. Wang, L. Xu and N.-P. Feng, Int. J. Pharm., 2014, 460, 280–288 CrossRef CAS PubMed.
- Y.-T. Zhang, L.-N. Shen, Z.-H. Wu, J.-H. Zhao and N.-P. Feng, Int. J. Pharm., 2014, 471, 449–452 CrossRef CAS PubMed.
- C. Fan, X. Li, Y. Zhou, Y. Zhao, S. Ma, W. Li, Y. Liu and G. Li, BioMed Res. Int., 2013, 161943 Search PubMed.
- L. Yang, L. Wu, D. Wu, D. Shi, T. Wang and X. Zhu, Int. J. Nanomedicine, 2017, 12, 3357–3364 CrossRef CAS PubMed.
- P. K. Jaiswal, S. Kesharwani, R. Kesharwani and D. K. Patel, J. Drug Deliv. Ther., 2016, 6, 7–17 CAS.
- X.-Q. Niu, D.-P. Zhang, Q. Bian, X.-F. Feng, H. Li, Y.-F. Rao, Y.-M. Shen, F.-N. Geng, A.-R. Yuan, X.-Y. Ying and J.-Q. Gao, Int. J. Pharm. X, 2019, 1, 100027 CAS.
- V. K. Rai, N. Mishra, K. S. Yadav and N. P. Yadav, J. Controlled Release, 2018, 270, 203–225 CrossRef CAS PubMed.
- H. H. Tayeb and F. Sainsbury, Nanomed, 2018, 13, 2507–2525 CrossRef CAS PubMed.
- N. Salim, N. Ahmad, S. H. Musa, R. Hashim, T. F. Tadros and M. Basri, RSC Adv., 2016, 6, 6234–6250 RSC.
- S. A. Yousef, Y. H. Mohammed, S. Namjoshi, J. E. Grice, H. A. E. Benson, W. Sakran and M. S. Roberts, Pharmaceutics, 2019, 11, 639 CrossRef CAS PubMed.
- O. Bayraktar, İ. Erdoğan, M. D. Köse and G. Kalmaz, in Nanostructures for Antimicrobial Therapy, Elsevier, 2017, pp. 395–412 Search PubMed.
- R. K. Harwansh, R. Deshmukh and M. A. Rahman, J. Drug Deliv. Sci. Technol., 2019, 51, 224–233 CrossRef CAS.
- A. de J. Cenobio-Galindo, J. Ocampo-López, A. Reyes-Munguía, M. L. Carrillo-Inungaray, M. Cawood, G. Medina-Pérez, F. Fernández-Luqueño and R. G. Campos-Montiel, Antioxidants, 2019, 8, 500 CrossRef CAS PubMed.
- Q. Liu, H. Huang, H. Chen, J. Lin and Q. Wang, Molecules, 2019, 24, 4242 CrossRef CAS PubMed.
- L. Pavoni, D. R. Perinelli, G. Bonacucina, M. Cespi and G. F. Palmieri, Nanomaterials, 2020, 10, 135 CrossRef CAS PubMed.
- D. S. Shaker, R. A. H. Ishak, A. Ghoneim and M. A. Elhuoni, Sci. Pharm., 2019, 87, 17 CrossRef CAS.
- E. Sánchez-López, M. Guerra, J. Dias-Ferreira, A. Lopez-Machado, M. Ettcheto, A. Cano, M. Espina, A. Camins, M. L. Garcia and E. B. Souto, Nanomaterials, 2019, 9, 821 CrossRef PubMed.
- C. Pulce and J. Descotes, in Human Toxicology, ed. J. Descotes, Elsevier Science B.V., Amsterdam, 1996, pp. 683–702 Search PubMed.
- Y. Lu, E. Zhang, J. Yang and Z. Cao, Nano Res., 2018, 11, 4985–4998 CrossRef PubMed.
- T. Tadros, in Encyclopedia of Colloid and Interface Science, ed. T. Tadros, Springer, Berlin, Heidelberg, 2013, pp. 209–210 Search PubMed.
- Y. Singh, J. G. Meher, K. Raval, F. A. Khan, M. Chaurasia, N. K. Jain and M. K. Chourasia, J. Controlled Release, 2017, 252, 28–49 CrossRef CAS PubMed.
- M. Miastkowska, E. Sikora and J. Ogonowski, Period. Polytech. Chem. Eng., 2014, 58, 43–48 CrossRef.
- M. Kumar, R. S. Bishnoi, A. K. Shukla and C. P. Jain, Prev. Nutr. Food Sci., 2019, 24, 225–234 CrossRef CAS PubMed.
- A. Gupta, A. Z. M. Badruddoza and P. S. Doyle, Langmuir, 2017, 33, 7118–7123 CrossRef CAS PubMed.
- O. Alliod, J.-P. Valour, S. Urbaniak, H. Fessi, D. Dupin and C. Charcosset, Colloids Surf., A, 2018, 557, 76–84 CrossRef CAS.
- L. Wang, X. Guan, C. Zheng, N. Wang, H. Lu and Z. Huang, Langmuir, 2020, 36, 10082–10090 CrossRef CAS PubMed.
- M. N. Yukuyama, D. D. M. Ghisleni, T. J. A. Pinto and N. A. Bou-Chacra, Int. J. Cosmet. Sci., 2016, 38, 13–24 CrossRef CAS PubMed.
- S. Manickam, K. Sivakumar and C. H. Pang, Ultrason. Sonochem., 2020, 69, 105258 CrossRef CAS PubMed.
- L. A. Pascual-Pineda, E. Flores-Andrade, M. Jiménez-Fernández and C. I. Beristain, Int. J. Food Sci. Technol., 2015, 50, 1174–1181 CrossRef CAS.
- A. Simonazzi, A. G. Cid, M. Villegas, A. I. Romero, S. D. Palma and J. M. Bermúdez, in Drug Targeting and Stimuli Sensitive Drug Delivery Systems, ed. A. M. Grumezescu, William Andrew Publishing, 2018, pp. 81–116 Search PubMed.
- C. Solans and I. Solé, Curr. Opin. Colloid Interface Sci., 2012, 17, 246–254 CrossRef CAS.
- M. Ferreira, L. Barreiros, M. A. Segundo, T. Torres, M. Selores, S. A. Costa Lima and S. Reis, Colloids Surf. B Biointerfaces, 2017, 159, 23–29 CrossRef CAS PubMed.
- S. Trombino, R. Russo, S. Mellace, G. P. Varano, A. S. Laganà, F. Marcucci and R. Cassano, J. Drug Deliv. Sci. Technol., 2019, 49, 563–569 CrossRef CAS.
- A. Ali, S. Ali, M. Aqil, S. S. Imam, A. Ahad and A. Qadir, J. Drug Deliv. Sci. Technol., 2019, 52, 713–721 CrossRef CAS.
- J.-H. Kang, J. Chon, Y.-I. Kim, H.-J. Lee, D.-W. Oh, H.-G. Lee, C.-S. Han, D.-W. Kim and C.-W. Park, Int. J. Nanomedicine, 2019, 14, 5381–5396 CrossRef CAS PubMed.
- P. Tripathi, A. Kumar, P. K. Jain and J. R. Patel, Int. J. Biol. Macromol., 2018, 120, 1322–1334 CrossRef CAS PubMed.
- N. Kaur, K. Sharma and N. Bedi, Pharm. Nanotechnol., 2018, 6, 133–143 CrossRef CAS PubMed.
- P. Sathe, R. Saka, N. Kommineni, K. Raza and W. Khan, Drug Dev. Ind. Pharm., 2019, 45, 826–838 CrossRef CAS PubMed.
- Y. O. Agrawal, U. B. Mahajan, H. S. Mahajan and S. Ojha, Int. J. Nanomedicine, 2020, 15, 4763–4778 CrossRef CAS PubMed.
- R. Kumar, S. Dogra, B. Amarji, B. Singh, S. Kumar, K. Vinay, R. Mahajan and O. Katare, JAMA Dermatol., 2016, 152, 807–815 CrossRef PubMed.
- S. Doppalapudi, A. Jain, D. K. Chopra and W. Khan, Eur. J. Pharm. Sci., 2017, 96, 515–529 CrossRef CAS PubMed.
- M. Walunj, S. Doppalapudi, U. Bulbake and W. Khan, J. Liposome Res., 2020, 30, 68–79 CrossRef CAS PubMed.
- J. Chen, Y. Ma, Y. Tao, X. Zhao, Y. Xiong, Z. Chen and Y. Tian, J. Liposome Res., 2020, 1–15 Search PubMed.
- W. Wang, G. Shu, K. Lu, X. Xu, M. Sun, J. Qi, Q. Huang, W. Tan and Y. Du, J. Nanobiotechnol., 2020, 18, 80 CrossRef CAS PubMed.
- H. Liu, R. S. Kang, K. Bagnowski, J. M. Yu, S. Radecki, W. L. Daniel, B. R. Anderson, S. Nallagatla, A. Schook, R. Agarwal, D. A. Giljohann and A. S. Paller, J. Invest. Dermatol., 2020, 140, 435–444 CrossRef CAS PubMed.
- R. Saka, H. Jain, N. Kommineni, N. Chella and W. Khan, J. Drug Deliv. Sci. Technol., 2020, 58, 101691 CrossRef CAS.
- S. R. M. Moghddam, A. Ahad, M. Aqil, S. S. Imam and Y. Sultana, Mater. Sci. Eng. C, 2016, 69, 789–797 CrossRef CAS PubMed.
- I. I. Abu Hashim, N. F. Abo El-Magd, A. R. El-Sheakh, M. F. Hamed and A. E.-G. H. Abd El-Gawad, Int. J. Nanomedicine, 2018, 13, 1059–1079 CrossRef CAS PubMed.
- V. Parkash, S. Maan, V. Chaudhary, V. Jogpal, G. Mittal and V. Jain, J. Bioequivalence Bioavailab., 2018, 10, 99–106 Search PubMed.
- M. Bahramizadeh, M. Bahramizadeh, B. Kiafar, A. H. Jafarian, A. R. Nikpoor, M. Hatamipour, H. Esmaily, Z. Rezaeemehr, S. Golmohammadzadeh, S. A. Moosavian and M. R. Jafari, Int. J. Pharm., 2019, 569, 118623 CrossRef CAS PubMed.
- A. Chandra, G. Aggarwal, S. Manchanda and A. Narula, Pharm. Nanotechnol., 2019, 7, 362–374 CrossRef CAS PubMed.
- D. Fathalla, E. M. K. Youssef and G. M. Soliman, Pharmaceutics, 2020, 12, 446 CrossRef CAS PubMed.
- S. H. Musa, M. Basri, H. R. Fard Masoumi, N. Shamsudin and N. Salim, Int. J. Nanomedicine, 2017, 12, 2427–2441 CrossRef CAS PubMed.
- S. Sahu, S. S. Katiyar, V. Kushwah and S. Jain, Nanomed, 2018, 13, 1985–1998 CrossRef CAS PubMed.
- P. Rajitha, P. Shammika, S. Aiswarya, A. Gopikrishnan, R. Jayakumar and M. Sabitha, J. Drug Deliv. Sci. Technol., 2019, 49, 463–476 CrossRef CAS.
- M. S. Algahtani, M. Z. Ahmad and J. Ahmad, J. Drug Deliv. Sci. Technol., 2020, 59, 101847 CrossRef CAS.
- M. S. Algahtani, M. Z. Ahmad, I. H. Nourein and J. Ahmad, Biomolecules, 2020, 10, 968 CrossRef CAS PubMed.
- R. Bosetti and S. L. Jones, Nanomed, 2019, 14, 1367–1370 CrossRef CAS PubMed.
- J. J. Richardson and F. Caruso, Nano Lett., 2020, 20, 1481–1482 CrossRef CAS PubMed.
- E. C. Murphy, S. W. Schaffter and A. J. Friedman, Curr. Dermatol. Rep., 2019, 8, 14–25 CrossRef.
- S. Saleem, M. K. Iqubal, S. Garg, J. Ali and S. Baboota, Expet Opin. Drug Deliv., 2020, 17, 817–838 CrossRef CAS PubMed.
- N. Ahmad, R. Ramsch, M. Llinàs, C. Solans, R. Hashim and H. A. Tajuddin, Colloids Surf. B Biointerfaces, 2014, 115, 267–274 CrossRef CAS PubMed.
- L. Bai and D. J. McClements, J. Colloid Interface Sci., 2016, 479, 71–79 CrossRef CAS PubMed.
- M. S. Behalo, E. Bloise, L. Carbone, R. D. Sole, D. Lomonaco, S. E. Mazzetto, G. Mele and L. Mergola, J. Exp. Nanosci., 2016, 11, 1274–1284 CrossRef CAS.
- O. W. Mak, N. Seman, N. H. Harun, R. S. D. Hussen, N. M. Rodzi and T. Heidelberg, J. Surfactants Deterg., 2015, 18, 973–980 CrossRef CAS.
- A. Sedaghat Doost, J. Van Camp, K. Dewettinck and P. Van der Meeren, Food Chem., 2019, 293, 134–143 CrossRef CAS PubMed.
- N. J. DeVito, S. Bacon and B. Goldacre, Lancet, 2020, 395, 361–369 CrossRef.
- M. Zwierzyna, M. Davies, A. D. Hingorani and J. Hunter, Br. Med. J., 2018, 361, k2130 CrossRef PubMed.
- J. K. Patra, G. Das, L. F. Fraceto, E. V. R. Campos, M. del Pilar Rodriguez-Torres, L. S. Acosta-Torres, L. A. Diaz-Torres, R. Grillo, M. K. Swamy and S. Sharma, J. Nanobiotechnol., 2018, 16, 71 CrossRef PubMed.
- C. L. Ventola, Pharm. Ther., 2017, 42, 742–755 Search PubMed.
- R. Kumar, S. V. Dalvi and P. F. Siril, ACS Appl. Nano Mater., 2020, 3, 4944–4961 CrossRef CAS.
- D. Lombardo, M. A. Kiselev and M. T. Caccamo, J. Nanomater., 2019, 2019, 3702518 Search PubMed.
- A. V. Singh, M. H. D. Ansari, D. Rosenkranz, R. S. Maharjan, F. L. Kriegel, K. Gandhi, A. Kanase, R. Singh, P. Laux and A. Luch, Adv. Healthc. Mater., 2020, 9, 1901862 CrossRef CAS PubMed.
|
This journal is © The Royal Society of Chemistry 2021 |