DOI:
10.1039/C6RA13851A
(Paper)
RSC Adv., 2016,
6, 66745-66755
Encapsulation of curcumin over carbon dot coated TiO2 nanoparticles for pH sensitive enhancement of anticancer and anti-psoriatic potential
Received
28th May 2016
, Accepted 23rd June 2016
First published on 28th June 2016
Abstract
The surface engineering of rutile phase TiO2 nanoparticles was performed by carbogenic carbon dots for biological applications. Carbon dots were synthesized by a green hydrothermal method from the ginger herb and TiO2 nanoparticles were synthesized by co-precipitation of TTIP. The carbon dots were successfully coated over the surface of TiO2 nanoparticles and the hydrophobic multi-therapeutic drug curcumin was wet loaded chemically. These nanostructures were characterized using UV-Vis, PL and FTIR spectroscopy and XRD and TEM analysis. The curcumin loaded C dot coated TiO2 nanoparticles have shown higher anticancer potential than unloaded nanoparticles on MCF-7 breast cancer cells and anti-psoriatic potential on HaCaT keratinocyte skin cells. The enhancement in biocompatibility was observed for curcumin after loading on TiO2 nanoparticles, thus destroying the hydrophobicity after C dot surface engineering.
1. Introduction
Titanium dioxide nanoparticles (TiO2NPs) are environmentally friendly and have good biocompatibility, along with weak toxicity, in vitro and in vivo on normal cells. However, TiO2 nanoparticles need surface functionalization with a surface coating by water dispersible agents such as cyclodextrins, polymeric carbohydrate derivatives or by carbon materials.11 Along with TiO2 functionalization, if drug loading is performed on the surface, then the biological activities may be enhanced and these nanosystems act as drug delivery platforms. Rutile phase TiO2 nanoparticles are mostly used in skin cell therapy in the form of lotions and creams for sunburn treatment.7 Therefore, these rutile nanoparticles have shown biocompatibility on skin cell models.8–10 Carbon dots are a special category of carbon nanomaterials containing plenty of mobile electrons with conjugated SP2 and SP3 carbon atom systems with C, H, O and major heteroatoms as constituents.4,5 These carbogenic C dots can be derived from waste and herb materials. These C dots generally show significant non-blinking fluorescence and can act as biomaterial labeling agents in cellular applications.1–3 The C dots increase band gaps, porosity and activities of biologically important nanomaterials such as TiO2 after surface engineering by coating and composite formation.6
Curcumin is a traditional hydrophobic multi-therapeutic drug used from ancient times in south Asia and possesses various biological activities such as anticancer, anti-oxidant, wound healing, and anti-Alzheimer's disease potentials. However, the use of curcumin is limited for therapy due to its hydrophobicity. Numerous attempts have been performed to destroy the hydrophobicity of curcumin and make it bioavailable, but very few attempts have been performed to enhance its anticancer, as well as anti-psoriatic, performances on breast cancer and especially on skin cancer models.6,9
Herein, in this study, we have synthesized carbogenic carbon nanodots by using the natural ginger herb. These carbon nanodots were coated over biocompatible TiO2 nanoparticles prepared by co-precipitation using a simple bottom up wet chemical method from TTIP. Then, the curcumin drug was successfully wet loaded onto the C dots coated TiO2 nanoparticles chemically.
After physicochemical characterization of these C dots, TiO2 nanoparticles, C dot coated TiO2 and curcumin loaded nanocomposites, the anticancer and anti-psoriatic performances of C dots, C dots coated TiO2 and curcumin loaded nanocomposites were elaborated by suitable in vitro SRB dye assays on HaCaT keratinocyte skin, psoriatic mutated and cancerous cell lines. The green and nontoxic nature of these nanohybrids on normal cells was determined by a suitable in vitro MTT assay on mouse McCoy fibroblast cell lines. The anticancer potentials of C dots, C dots coated TiO2 nanoparticles and curcumin loaded nanocomposites were determined on MCF-7 human breast cancer cells by MTT assay, eosin–hematoxylin dying and cell culture imaging methods.
2. Materials and methods
All chemicals used for synthesis of nanostructures, biocompatibility, anticancer, and anti-psoriatic screening were of A. R. grade and were procured from S. D. fine chem. Ltd or Merck Ltd. Cell culture medium, MCF-7, HaCaT keratinocyte and mouse McCoy fibroblast cell lines were procured from NCCS center, Pune, India. Double distilled water was obtained from a Millipore system and used for both synthesis and biological screening.
2.1 Synthesis of C dot coated TiO2 nanostructures and curcumin loading
Fresh tenders of ginger were purchased from a local market, peeled, cut into small pieces and then crushed by mortar and pestle. The aqueous extract was taken in an appropriate volume (25 mL) in a beaker. Then, 0.01 M NaOH (10 mL) and 15 mL double distilled water was added into a beaker and the basic extract of ginger was carbonized at 200 °C for 3 hours over a hotplate under ambient air. The residue was diluted in 150 mL water and dialyzed through a dialysis membrane with porosity 2 nm in dialysis bags with sizes 10 kDa for 8 h to get a brown C dots solution. These carbogenic C dots were dried in an oven on Petri plates whenever required. C dots coated curcumin loaded TiO2 nanoparticles were synthesized by wet chemical precipitation by co-precipitation of TTIP (titanium tertiary isopropoxide) by adding 15 mL ethanol dropwise to 5 mL TTIP, stirring the solution for 3 h and then the hydroxide precipitate was dried in an oven and finally calcined at 450 °C for 3 h. Furthermore, a 5 mg mL−1 suspension of TiO2 nanoparticles in water, about 20 mL, was sonicated and stirred with about 10 mL of a 10 μg mL−1 original C dot suspension for 3 h. Then, the precipitate was washed three times with water and centrifuged at 600 rpm for 20 min. Then, the precipitate was dried in an oven below 100 °C. These C dot coated TiO2 nanoparticles, about 5 mg mL−1, were suspended in water and stirred for 2 h with 1 mg mL−1 alcoholic curcumin after sonication. Curcumin loading was performed after stirring for 2 h to form the required nanostructure. The curcumin loaded C dots coated TiO2 nanocomposites were washed 3 times with water and suspended in water and whenever required, these nanocomposites dried in an oven.
Structural and morphological characterization of C dots, TiO2 nanoparticles and curcumin loaded C dot coated TiO2 nanostructures. The structure, packing, morphology, particle size and types of atoms of C dots, bare TiO2, C dot coated TiO2, and curcumin loaded nanostructures were confirmed by physicochemical characterization on the basis of UV-Vis, PL and FTIR spectral analysis, and TEM, XRD and DLS analysis. A Systronics UV-Vis spectrometer, JASCO type PL spectrophotometer and Perkin Elmer series FTIR spectrometer with KBr pallet technique were used for spectral analysis. A JEUL type TEM instrument, powder diffraction XRD techniques with CuKα source and Malvern type DLS instrument were used for structural and morphological characterization of the nanostructures.
Drug loading efficiency. The curcumin loading amounts, in percentages, in the nanocomposites were determined by a real time simultaneous UV-Visible spectrometric assay method. In brief, during the synthesis of curcumin loaded C dots capped TiO2 nanohybrids, the un-reacted curcumin in the supernatant was assessed at 460 nm. 2 mg of the dried nanocomposites were sonicated in 20 mL ethanol for 10 minutes and freed curcumin in supernatant solution measured against ethanol as blank at the same wavelength to obtain a w/w mass of curcumin in the nanohybrids. The absorbances were related to concentrations of curcumin from the standard loading formulation concentration of 5 mg mL−1. Finally, the drug loading percentage and efficiencies in the nanohybrids were determined using formula:
2.2 Anti-psoriatic potential of C dot coated TiO2 nanoparticles and curcumin loaded nanocomposites by in vitro SRB dye assay on HaCaT keratinocyte skin cancer cell lines
The sulphorhodamine B (SRB) assay is used for in vitro anti-psoriatic studies and anticancer potential of the nanomaterial on skin cancer models due to the staining of SRB dye on skin cancer cells for microscope imaging. Herein, the cell culture was trypsinized and the cell count adjusted to 1.0 × 103 cells per mL using growth medium into a 96 well microtitre plate. Then, 0.1 mL of the diluted cell suspension (approximately 500 cells per well) was added. After 24 hours, when a partial monolayer was formed, the supernatant was flicked off, the monolayer was washed once and 100 μL of the drug dilution prepared in maintenance media at 10, 50, or 100 μg mL−1 of nanomaterials was added per well into the microtitre plates. The plates were then incubated at 37 °C for 3 days in a 5% CO2 atmosphere, as mutated skin cells are less reactive, and microscopic examination was carried out with observations recorded every 24 hours. After 72 hours, 25 μL of 50% trichloroacetic acid was added to the wells gently such that it forms a thin layer over the nanomaterial drug dilutions to form an overall concentration of 10%. The plates were then incubated at 4 °C for one hour. The plates were flicked, the culture was washed five times with distilled water to remove traces of media, drug and serum, and was then air dried. The air-dried plates were stained with SRB for 30 minutes. The unbound dye was then removed by rapidly washing four times with 1% acetic acid. The plates were then air-dried. 100 μL of 10 mM Tris base was then added to the wells to solubilize the dye. The plates were shaken vigorously for 5 minutes. The cell culture imaging was performed for three concentrations of nanomaterial dosing under microscopic analysis. The absorbance was measured using a microplate reader at a wavelength of 540 nm. The cells without drug dosing were treated as the control. The activities were tested for nanomaterials with three various concentrations using the formula as follows:
2.3 Cell viability of normal cell lines for exposure of nanomaterials by MTT assay on mouse McCoy fibroblast normal cell mimics
Normal McCoy fibroblast cell lines were grown and maintained in suitable (DMEM (E) with NEAA + FCS) culture media. All cells were trypsinated using trypsin–EDTA solution and seeded in 96-well plates. The newly prepared and fresh phosphate buffered [pH = 7.4] nanomaterials with the same three concentrations were evaluated for their in vitro cell viability effects against normal McCoy fibroblast cell lines by the standard MTT assay. Untreated cells were considered as controls. The cell survival percentages by nanomaterial exposure was determined by the following formula,
Surviving cells (%) = mean OD of test material/mean OD of control × 100%. |
2.4 Anticancer potential of free C dots, TiO2, C dot coated TiO2 nanoparticles and curcumin loaded nanocomposites by in vitro MTT assay on MCF-7 human breast cancer cell lines
MCF-7 (human breast cancer) cell lines were purchased from NCCS center, Pune, India. MCF-7 cell lines were grown and maintained in suitable (DMEM) media and were grown and sub cultured in medium supplemented with 10% fetal bovine serum, 1% L-glutamine and 1% penicillin streptomycin antibiotic solution. All cells were trypsinated using trypsin–EDTA solution and seeded in 96-well plates. The newly synthesized nano-assemblies, with three concentrations in phosphate buffer saline solutions, were evaluated for their in vitro cytotoxic effects against the MCF-7 (breast cancer cell line), by the standard MTT (3-(4,5-dimethylthiazol-2-yl)-2,5-diphenyl tetrazolium bromide) assay and compared with free curcumin and bare C dots. Cells without dosing were treated as controls. In brief, the MCF-7 cell line was maintained in DMEM medium supplemented with 10% fetal bovine serum. The cells were plated at a density of 1 × 103 cells per well in 96-well plates and cultured for 24 h at 37 °C. The cells were subsequently exposed to 10, 50 and 100 μg mL−1 concentrations of nano-formulations. The plates were then incubated for 48 hours and cell proliferation or apoptosis (programmed death) was measured by adding 10 μL of MTT dye (5 mg mL−1 in phosphate-buffered saline) per well. The plates were incubated for a further 4 h at 37 °C in a humidified chamber containing 5% CO2. The formazan crystals formed due to reduction of dye by viable cells in each well were dissolved in 200 μL DMSO (dimethyl sulphoxide) and absorbance was read at 490 nm. Lastly, the percent cytotoxicity of the nanomaterials was calculated using the formula as follows:
Percent cytotoxicity in terms of cell viability = reading of control [OD1] − reading of treated cells [OD2]/reading of control [OD1] × 100%. |
3. Results and discussion
3.1 Morphological and structural characterization of nanostructures
UV-Vis absorption and PL spectra of C dots and C dot coated TiO2. The UV-Vis spectra of C dots have shown two absorption peaks at 210 nm and 315 nm with long tailing in the visible spectra. These observations clearly indicate the presence of π and non-bonding electrons in carbogenic C dots [Fig. 1A]. The absorption spectra of C dots prove that two transitions of π and non-bonding electrons elaborate the conjugated system of carbogenic C dot carbon atoms. The PL spectra of C dots in Fig. 1C prove the morphology and fluorescence ability of ginger derived carbon nanodots. The C dots synthesized from ginger exhibited non-blinking fluorescence in the PL spectra with an emission peak at 470 nm. This observation supports the good fluorescence property of C dots due to presence of free electrons. The bare TiO2 nanoparticles and C dot coated TiO2 nanostructures in the absorption spectra in Fig. 1B show peak shifting in the UV-Vis spectra that show the surface engineering of TiO2 with C dots, as the main peak of TiO2 nanoparticles from 212 nm, have shifted and damped at 209 nm due to C dots coating. This again was confirmed in the PL spectra of C dot coated TiO2 nanoparticles showing quenching and peak shifting of C dot emission by TiO2 in Fig. 1D from 470 nm to 645 nm. All these evidences clearly indicate the concentration quenching of surface adsorbed C dots on TiO2 when compared to the normal fluorescence spectra of C dots, as evidenced with peak shifting in the fluorescence emission. These observations prove that the TiO2 nanoparticles are C dots surface functionalized, with their increased electron transition property, which is required for biomedical applications. These UV-Vis absorption and PL emission spectra observations support the better coating of C dots over TiO2 nanoparticles increases the surface activity of TiO2 nanoparticles for specific biological application.
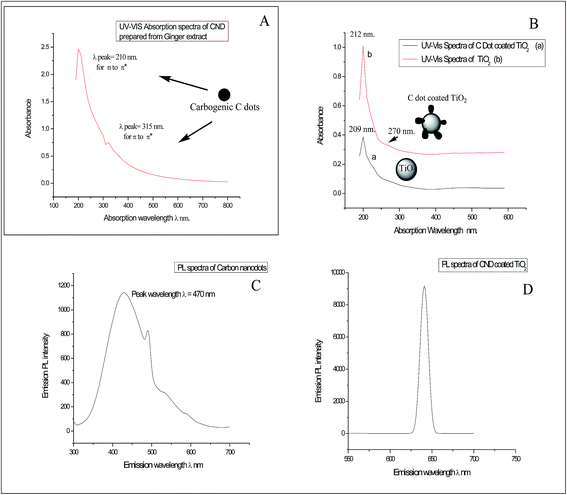 |
| Fig. 1 (A) UV-Vis absorption spectra of C dots derived from ginger. (B) UV-Vis absorption spectra of C dots coated TiO2 and bare TiO2 nanoparticles. (C) PL spectra of C dots. (D) PL spectra of C dots coated TiO2 nanoparticles. | |
FTIR spectra of nanostructures. The FTIR spectra of C dots show a peak frequency at 3486 cm−1 and provide evidence for the presence of aromatic –OH groups. The peak at 2930 cm−1 shows the presence of a conjugated diketonic group. The peak at 1644 cm−1 may be attributed to aromatic –OCH3. All other peaks of the C dots spectra in the fingerprint area may be present due to presence of conjugated –CH groups, aromatic-H, and –C
O stretching [Fig. 2]. The FTIR spectra of bare TiO2 and C dot coated TiO2 in the figure shows different stretching and scissoring frequencies for O–Ti–O bonds in the rutile phases in both spectra, which was due to surface engineering effects of C dots onto the TiO2 nanoparticles by adsorption and weak bonding effects. The three FTIR spectra of free C dots, bare TiO2 and C dots coated TiO2 nanoparticles can be inter-related to prove the surface coating of TiO2 nanoparticles and the presence of conjugated aromatic and diketonic carbon groups in C dots. As per FTIR observations, it is proved that C dots derived from ginger are of carbogenic C dots. Some of the C
N groups in the FTIR spectra were observed for C dots; therefore, this observation demonstrates that the presence of conjugated free electrons containing C, H, O and some N atoms of C dots are interacting with TiO2 nanoparticles for functionalization and activation of the surface. Furthermore, the FTIR observations evidence the presence of polyphenolic fragments in the C dot constituents.
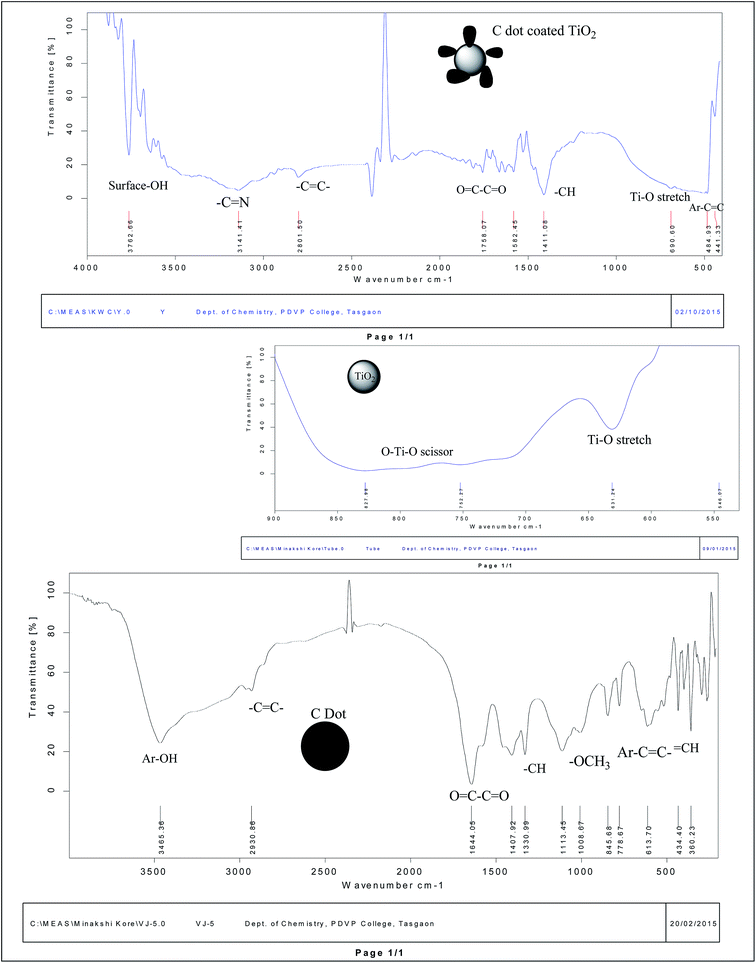 |
| Fig. 2 FTIR spectra of C dots coated TiO2, bare TiO2 nanoparticles and C dots from top to bottom. | |
As FTIR spectra of C dots had revealed the presence of fragments of 6-gingerol and curcuminoids [natural polyphenolic groups of herb ginger],13 which are the minor polyphenolic constituents of herb ginger, the synthesized carbogenic C dots contain π bonded carbon atoms with conjugated C, H, O and some N groups of some polyphenolic fragments and aromatic hydroxyl, along with some –C
C– and –C
N– conjugated groups and in quantum dot materials [as per ref. 12 and 13]. Therefore, after the hydrothermal process on the ginger extract, the carbon residue contains these basic backbone units inside the carbogenic carbon quantum material as C dots. This evidence supports the porous and amorphous nature of the C dots with electronegative atoms that coat TiO2 nanoparticles for functionalization and the ability of these nanohybrids for curcumin loading.
XRD spectra of nanostructures. Fig. 3a presents the XRD spectra of synthesized rutile phase bare TiO2 nanoparticles, C dot coated TiO2 and pure C dots separately. The XRD spectra proved the surface coating of C dots over rutile nanocrystals of TiO2. The XRD peak intensities of bare TiO2 nanoparticles are slightly lifted up due to C dot surface engineering. The crystallite sizes of C dots, bare and C dot coated TiO2 are determined by Debye Scherrer's formula as, K = 0.9λ/β; cos
θ = 22 nm, where β = FWHM, λ = 1.54 nm, θ = diffraction angle, and K = crystallite size. The crystallite sizes were matched with TEM particle sizes of 4 nm and 27 nm for the C dots and C dots coated TiO2 nanoparticles, respectively. While bare rutile TiO2 nanoparticles show a crystallite size 23 nm, the rutile phase of TiO2 and the lattice parameters were confirmed by matching the spectra with JCPDS card no. 82-0514. Therefore, the XRD spectra of bare TiO2 and C dot coated nanoparticles have supported the observations of TEM analysis for coating and functionalization of the TiO2 nanoparticle surfaces with C dots [Fig. 3a and c]. The increase in peak broadness in the XRD spectra of C dots coated TiO2 nanoparticles explains the amorphous nature and porous nature of C dots surface engineered rutile nanoparticles. Same supportive evidences can be observed in the SAED patterns of TEM images of C dots coated TiO2 nanoparticles. The rutile phase of TiO2 nanoparticles was maintained even after C dots surface functionalization, as per observations in the XRD spectra of these nanostructures.
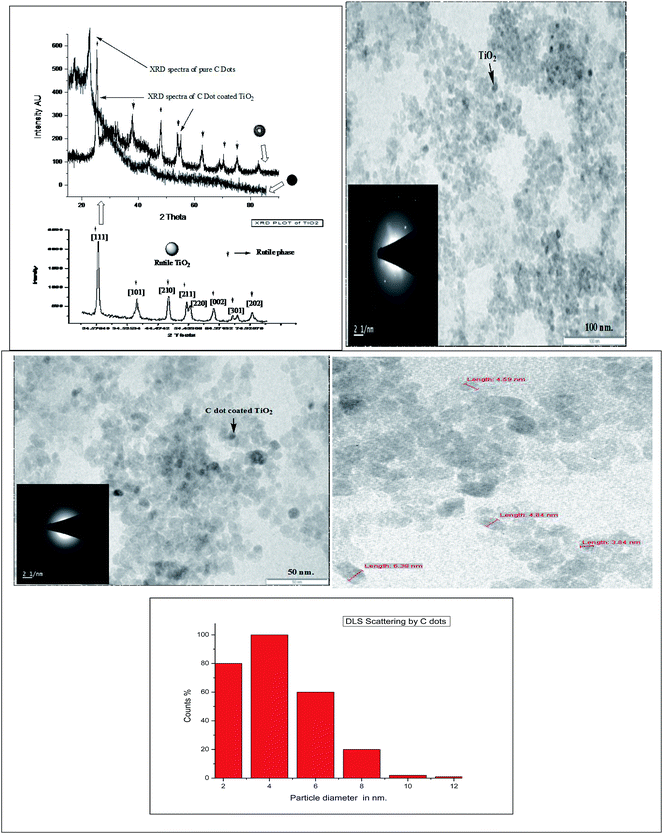 |
| Fig. 3 (a) XRD spectra of respective C dots, TiO2 and C dots coated TiO2 nanostructures. (b) TEM image and SAED pattern of bare TiO2 nanoparticles. (c) TEM image and SAED pattern of C dot coated TiO2 nanoparticles. (d) TEM image of bare C dots. (e) From top to bottom, DLS scattering for HDD = 4 to 6 nm for C dots. | |
TEM images and DLS analysis. The DLS scattering spectra of C dots aqueous suspension reveal that the average hydrodynamic diameter HDD or size of the carbon nanoparticles is 4 nm, which was matched with the TEM image of C dots and with the size of these carbon quantum nanoparticles. The sizes of these green synthesized C dots varies from 2 to 8 nm [Fig. 3d and e], but the maximum C dots sizes are between 2 and 6 nm. Therefore, these are quantum dot carbon nanoparticles with an abundance of mobile electrons responsible for light scattering in DLS and electron scattering in TEM analysis. This not only proves the carbogenic conjugated carbon and quantum dot nature of the C dots, but also proves the presence of abundant free electrons. The TEM image of C dots had proved that there is some aggregation, showing the amorphous nature and circular morphology of the CNDs [carbon nanodots] material. The TEM images of bare and C dots coated TiO2 nanoparticles [Fig. 3b and c] supports the XRD spectra observations for surface engineering and the increase in activity. The SAED patterns also explain the crystalline to amorphous phase change of TiO2 nanoparticles by C dots surface functionalization. Overall, this proves the probability for biomedical activities of C dots coated TiO2 nanoparticles.
3.2 Anticancer and anti-psoriatic property of C dots, bare and curcumin loaded TiO2 nanostructures by MTT and SRB dye assay and cell surviving effects of nanostructures on normal fibroblast cells by the MTT assay [cell pH effects]
As TiO2 nanoparticles and especially biocompatible rutile phase nanoparticles are mainly used in skin formulations, enhancement of the anticancer potential of curcumin through loading into C dot coated TiO2, and destroying the hydrophobicity [as per ref. 6], was tested on the skin cancer model HaCaT keratinocyte cell lines by SRB dye assay [refer Fig. 5 images and cell viability plots in Fig. 4a]. The prepared synthetic nanostructures have displayed moderate to good inhibition activities against human skin cancer cell lines. It can be noted that the C dot coated curcumin loaded TiO2 nanostructures exhibited significant and higher inhibitory activities against HaCaT keratinocyte cell lines with means of 60.71%, 52.38%, and 47.61% growth inhibition for three concentrations after triplicate measurements at 100, 50, and 10 μg mL−1, respectively, concentrations compared to the positive control drug 5-fluorouracil (5-FU) (70.23%, 61.90%, and 52.36%, respectively) as 5-FU is used for skin cancer treatment. The non-functionalized rutile TiO2 nanoparticles have shown low anti-psoriatic activity over the C dots functionalized TiO2 nanostructures [refer Fig. 4a and images in Fig. 5]. Therefore, the C dots coated TiO2 rutile nanoparticles are the best platform for delivery of hydrophobic drugs such as curcumin on to skin cancer models for hydrophobic drug delivery and to increase the biocompatibility. These surface functionalized TiO2 nanoparticles delivers drugs to acidic tumor pH activating tumor cell damage factors, such as caspases 3 and 9, producing ROS (reactive oxygen species) by mitochondrial membrane activation from C dots coated TiO2 nanoparticles after endocytosis and curcumin release at the acidic cancer cell pH. Therefore, these C dots functionalized TiO2 nanoparticles are better alternatives for hydrophobic drug delivery, especially on skin cancers. As curcumin has a less stable keto form at basic pH and an enol form at acidic pH, in cancer and mutated cells, the acidic pH stable enol form predominates and this was released from the nanocomposites destabilizing the stable keto form loaded into C dots coated TiO2 nanocomposites. The overall oxidative stress produced in skin cancer mutated cell compartments is higher for curcumin loaded nanocomposites than for free C dots and C dot coated TiO2 nanoparticles, which increased apoptosis of keratinocyte cells observed by the SRB assay. In the MCF-7 cell compartments, the same observations were given from these nanostructures by the MTT assay. Curcumin loaded C dots coated TiO2 nanoparticles have shown higher apoptosis of MCF-7 breast cancer cells than free C dots, TiO2 and C dots coated rutile nanoparticles, as observed in Fig. 6a–d. The apoptosis of MCF-7 cells were studied by eosin–hematoxylin dye staining after MTT assay and imaging under microscope, as this staining of cells is used to view tissue, as well as cell morphology and drug endocytosis. About 88% apoptosis was observed for MCF-7 cells by curcumin loaded C dots coated rutile nanocomposites, elaborating their applications to future in vitro and in vivo anticancer biomedical applications. The MTT assay of these nanostructures on normal McCoy mouse fibroblast cells proves the nontoxic nature of the nanomaterial on normal cells that result in an enhancement of cell division at basic pH by activation of normal cell growth from less stable curcumin at basic physiological pH, as shown in Fig. 4b. This MTT assay of nanostructures on normal mouse cells proved the nontoxic nature of the nanomaterial on normal cells. Therefore, the nanomaterials are pH sensitive, cytotoxic to mutated cells and nontoxic to normal cells.
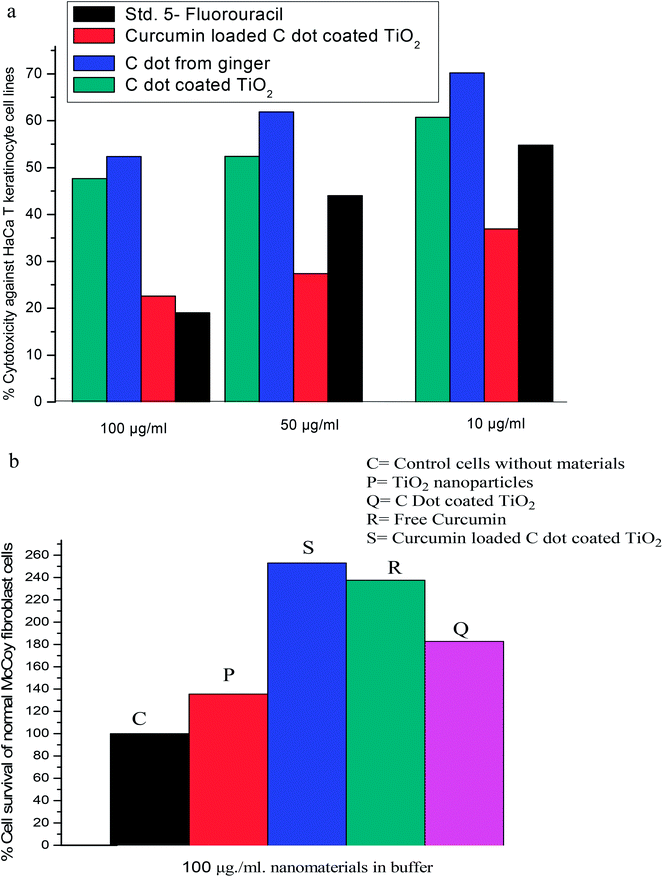 |
| Fig. 4 (a) Anti-psoriatic effects of nanostructures by selective SRB assay for cell viability on HaCaT keratinocyte skin cancer cells. (b) Cell growth effects of nanostructures on mouse fibroblast normal cells by MTT assay. | |
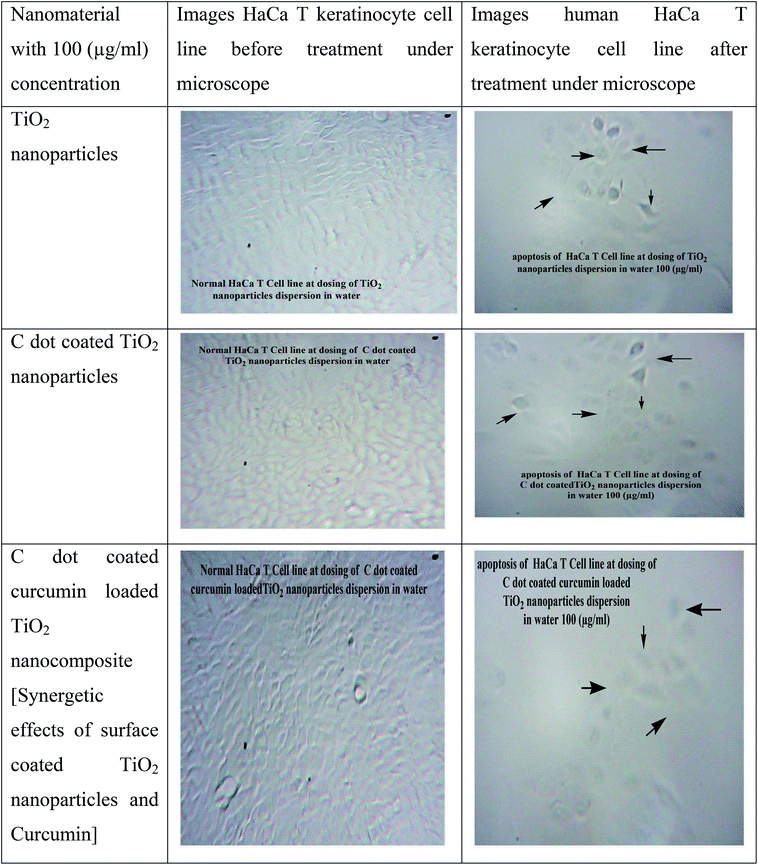 |
| Fig. 5 Microscopic images of HaCaT keratinocyte cells upon exposure to nanostructures [anti-psoriatic effects of nanostructures by SRB dye staining assay]. | |
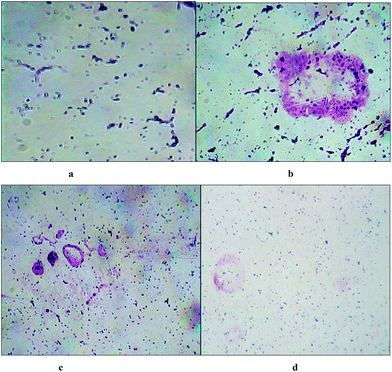 |
| Fig. 6 (a) Apoptotic cell membrane destruction image of MCF-7 human breast cancer cells upon exposure to 100 ppm of free C-dots in phosphate saline buffer by MTT assay – the cells were dyed with eosin hematoxylin dye; (b) apoptosis image of MCF-7 cells in the presence of TiO2 nanoparticles; (c) apoptosis image of MCF-7 cells in the presence of C-dot coated TiO2 nanoparticles and (d) apoptosis image of MCF-7 cells in the presence of curcumin loaded C-dot coated TiO2 nanocomposites with high activity. | |
3.3 Mechanisms for curcumin loading efficiency and C dots surface engineering of rutile TiO2 nanoparticles
The curcumin loading percentage, observed by UV-Vis spectrometric assay, is 89%, which is the highest among all other drug delivery vehicles for curcumin reported in the literature. Therefore, the C dots surface engineering results in the highest hydrophobic drug loading efficiency over rutile phase TiO2 nanoparticles. The probable mechanism of curcumin encapsulation is based on a keto form interaction of curcumin with C dots coating the rutile nanoparticles by weak van der Waals supramolecular type bonding and electrostatic interactions. C dots cause the amorphous nature and low agglomerations of C dots coated rutile nanoparticles and therefore have plausible endocytosis into normal and cancer cells due to low agglomerations before endocytosis. Therefore, these C dots functionalized curcumin loaded rutile nanoparticles are the better platform model for hydrophobic drug delivery, with the highest encapsulation and release efficiencies, resulting in high biocompatibility. The carbogenic carbon dots functionalize rutile nanoparticles by surface adsorption and coating, thus increasing the activity and porosity of rutile nanoparticles along with increasing the adsorption capacity of the curcumin payload. Curcumin is encapsulated in C dots and bonded to rutile nanoparticles that are stabilized at basic pH with the keto form. This keto form can also be converted to the enol form of the diketonic group of curcumin in the acidic pH of the mutated cell environment for detachment and release of curcumin. Therefore, the skin cancer biocompatibility and breast cancer acidic pH drug release of rutile TiO2 nanoparticles is increased by C dots surface engineering and multi-therapeutic curcumin loading.
3.4 pH sensitive enhancement in the anticancer and anti-psoriatic potentials of curcumin after encapsulation in C dots coated TiO2 nanoparticles
Curcumin is released at acidic pH more quickly, giving the active enol form of the diketone constituent in cancer and psoriasis cell environments. This causes more apoptosis at acidic pH along with separate effects of rutile nanoparticles and C dots, as these are activated by surface engineering and by formulation of nanocomposites. The pH sensitive release of curcumin occurs in cancer cell environments reacting curcumin with mutated DNA and activating pro-apoptotic factors such as caspase enzymes and Bax proteins that produce ROS from triggering the mitochondrial membrane. Therefore, at acidic pH, curcumin releases quickly to cause apoptosis. At basic normal cell or blood pH, curcumin from nanocomposites released slowly, which increases cell division and cell growth factors.
4. Conclusion
In conclusion, this study reports the green and hydrothermal synthesis of carbon nanodots from the natural ginger herb and its coating over biocompatible rutile TiO2 nanoparticles to form a drug delivery vehicle for the multi-active drug curcumin. Herein, the curcumin was successfully loaded to destroy its hydrophobicity for in vitro anticancer and anti-psoriatic performances. The skin cancer biocompatibility of TiO2 and the anticancer potential of curcumin have been enhanced using C dots surface engineering for rutile nanoparticles. The better biocompatibilities were exhibited by all the nanostructures on skin cancer model cells and breast cancer cells. The curcumin loaded nanocomposites show cell growth properties of normal cells and destruction properties for mutated cells proving future biomedical anticancer and anti-psoriatic therapy applications are possible. The pH sensitive enhancement of the anticancer potential of curcumin has been discussed. Therefore, carbon dots are a better green option for surface coating and functionalization of TiO2 nanoparticles for better biocompatibility and delivery of hydrophobic drugs such as curcumin. These nanocomposites of curcumin with better anticancer and anti-psoriatic potential, along with their nontoxic nature on normal cells, also find applications for in vivo cancer therapy and photodynamic therapy.
Acknowledgements
The corresponding author is thankful to SAIF center, IIT, Mumbai, India for providing TEM characterization facility and to Biotechnology department of K.W. College, Sangli and Pharmaceutical Chemistry department of A. Birnale pharmacy college, Sangli, India for providing the biological screening facilities.
References
- M. J. Krysmann, A. Kelarakis, P. Dallas and E. P. Giannelis, Formation mechanism of carbogenic nanoparticles with dual photoluminescence emission, J. Am. Chem. Soc., 2012, 134, 747–750 CrossRef CAS PubMed.
- Y. Song, S. Zhu, S. Xiang, X. Zhao, J. Zhang, H. Zhang, Y. Fu and B. Yang, Investigation into the fluorescence quenching behaviors and applications of carbon dots, Nanoscale, 2014, 6, 4676–4682 RSC.
- D. Ding, C. C. Goh, G. Feng, Z. Zhao, J. Liu, R. Liu, N. Tomczak, J. Geng, B. Z. Tang, L. G. Ng and B. Liu, Ultrabright organic dots with aggregation-induced emission characteristics for real-time two-photon intravital vasculature imaging, Adv. Mater., 2013, 25, 6083–6088 CrossRef CAS PubMed.
- T. Lai, E. Zheng, L. Chen, X. Wang, L. Kong, C. You, Y. Ruan and X. Weng, Hybrid carbon source for producing nitrogen-doped polymer nanodots: one-pot hydrothermal synthesis, fluorescence enhancement and highly selective detection of Fe(III), Nanoscale, 2013, 5, 8015–8021 RSC.
- Y. Sun, W. Cao, S. Li, S. Jin, K. Hu, L. Hu, Y. Huang, X. Gao, Y. Wu and X. J. Liang, Ultrabright and multicolorful fluorescence of amphiphilic polyethyleneimine polymer dots for efficiently combined imaging and therapy, Sci. Rep., 2013, 3, 3036 Search PubMed.
- C. Wu and D. T. Chiu, Highly fluorescent semiconducting polymer dots for biology and medicine, Angew. Chem., Int. Ed., 2013, 52, 3086–3109 CrossRef CAS PubMed.
- J. Lausmaa, P. Löfgren and B. Kasemo, Adsorption and co adsorption of water and glycine on TiO2, J. Biomed. Mater. Res., 1999, 44, 227–242 CrossRef CAS PubMed.
- L. K. Adams, D. Y. Lyon and P. J. J. Alvarez, Comparative ecotoxicity of nanoscale TiO2, SiO2, and ZnO water suspensions, Water Res., 2006, 40, 3527–3532 CrossRef CAS PubMed.
- P. J. A. Borm, D. Robbins, S. Haubold, T. Kuhlbusch, H. Fissan, K. Donaldson, R. Schins, V. Stone, W. Kreyling, J. Lademann, J. Krutmann, D. Warheit and E. Oberdorster, The potential risks of nanomaterials: a review carried out for ECETOC, Part. Fibre Toxicol., 2006, 3, 11 CrossRef PubMed.
- J. Chen, H. Zhou, A. C. Santulli and S. S. Wong, Evaluating cytotoxicity and cellular uptake from the presence of variously processed TiO2 nanostructured morphologies, Chem. Res. Toxicol., 2010, 23, 871–879 CrossRef CAS PubMed.
- X. Chen and S. S. Mao, Titanium dioxide nanomaterials: synthesis, properties, modifications, and applications, Chem. Rev., 2007, 107(7), 2891–2959 CrossRef CAS PubMed.
- A. Mewada, S. Pandey, S. Shinde, N. Mishra, G. Oza and M. Thakur, et al., Green synthesis of biocompatible carbon dots using aqueous extract of Trapa bispinosa peel, Mater. Sci. Eng., C, 2013, 33(5), 2914–2917 CrossRef CAS PubMed.
- C.-L. Li, C.-M. Ou, C.-C. Huang, W.-C. Wu, Y.-P. Chen, T.-E. Lin, L.-C. Ho, C.-W. Wang, C.-C. Shih, H.-C. Zhou, Y.-C. Lee, W.-F. Tzeng, T.-J. Chiou, S.-T. Chu, J. Cangm and H.-T. Chang, Carbon dots prepared from ginger exhibiting efficient inhibition of human hepatocellular carcinoma cells, J. Mater. Chem. B, 2014, 2, 4564–4571 RSC.
|
This journal is © The Royal Society of Chemistry 2016 |