DOI:
10.1039/C6RA03637F
(Paper)
RSC Adv., 2016,
6, 33998-34004
A wash-durable polyelectrolyte complex that extinguishes flames on polyester–cotton fabric†
Received
8th February 2016
, Accepted 29th March 2016
First published on 30th March 2016
Abstract
Polyester–cotton (PECO) blends are widely used in the apparel industry due to their desirable combination of comfort and durability, but their high flammability remains a safety concern. Layer-by-layer (LbL) assembly has proven to be a powerful tool in reducing the flammability of textiles, although the high number of processing steps is a challenge. In an effort to produce nanocoatings similar to an existing LbL recipe, consisting of poly(allylamine) and a polyphosphate, deposition of a water-soluble polyelectrolyte complex (PEC) onto PECO fabric was investigated. Treatment of this one-step coating with an acidic buffer yields a water-insoluble nanocoating capable of extinguishing flames during vertical flame testing, outperforming a similar multilayer coating assembled layer-by-layer. Pyrolysis-combustion flow calorimetry revealed a total heat release reduction of 30% for PECO coated with 18 wt% PEC. The coated fabric also exhibited a 78% and 31% reduction in peak heat release for cotton and polyester, respectively. In addition to stopping fire, this coating is durable to five home laundering cycles (and eight hours in boiling water) without losing any flame retardant activity. Although microscopy revealed identical structures in LbL and PEC coatings, elemental analysis revealed a difference in composition. The PEC coating showed greater flame retardancy than layer-by-layer assembled coatings using the same ingredients, while also greatly reducing the number of processing steps, making this a promising treatment for large scale fabric protection.
Introduction
Over the past ten years fabrics made of synthetic fibers, such as polyester, have emerged as the largest component of the textile industry and continue to increase in demand.1 Polyester–cotton (PECO) blends are used to combine comfort and breathability (of cotton) with strength and durability (of polyester).2 The high flammability of these textiles, however, pose a significant danger. More than 4300 serious burn injuries associated with clothing occur annually in the United States.3 The hazard associated with PECO-based apparel is further worsened by polyester's tendency to melt, clinging to the wearer's body and causing severe burning.4 Reducing fabric flammability is a challenge due to the combination of melting polyester and non-melting cotton fibers that tend to produce a scaffolding effect, resulting in greater flammability than that of the individual components.4 Approaches developed to reduce PECO flammability include the use of organohalogen additives and flame retardant (FR) back-coatings.5–8 Using additives at the fiber spinning stage often results in weaker fibers as well as difficulties in spinning.9,10 Furthermore, halogen additives have been linked to biological persistence and toxicity.11,12
Many of the challenges associated with flame retardant approaches for fabric have been recently overcome by applying conformal coatings using layer-by-layer (LbL) assembly.13–16 This technology has been shown to render polyester,17,18 ramie,19 and cotton13,17,20–23 fabric flame retardant using environmentally-benign aqueous solutions applied under ambient conditions. LbL assembly involves the deposition of thin films by alternate layering of oppositely charged polyelectrolytes that impart the desired functionality onto a substrate.24–26 Although these treatments are effective, the LbL process involves multiple cycles of immersion to deposit enough flame retardant to achieve appreciable flame suppression (Fig. 1). In an effort to apply FR coatings of similar chemistry onto fabric with very few processing steps, a method involving water-soluble polyelectrolytes complexes (PEC) from a single solution was recently developed.27,28 Two different fabric types were rendered self-extinguishing by applying a nanocoating in just two or three steps using the pH-dependent solubility of PECs. PECs are formed by the entropy-driven association of oppositely charged polyelectrolytes in water. Their solubility is largely dependent on concentration, ionic strength, and in the case of weak polyelectrolytes, solution pH.29–31 The flame retardant PECs were kept stable at high pH and deposited onto fabric. After drying, the PEC was rendered water-insoluble by exposure to an acidic buffer solution (Fig. 1).
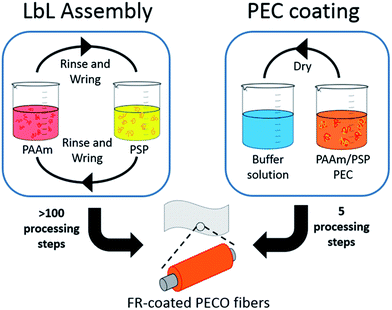 |
| Fig. 1 Schematic of the LbL assembly and PEC coating procedures for applying poly(allylamine) and poly(sodium phosphate) onto polyester–cotton blend fabric. | |
To better demonstrate the effectiveness of the PEC-deposition technique, this study set out to directly compare flame retardant PEC and LbL coatings prepared with the same polyelectrolytes. Coatings deposited layer-by-layer with poly(allylamine) and poly(sodium phosphate) were previously shown to promote char formation on cotton fabric.21 This multilayered nanocoating is compared to a polyelectrolyte complex coating in terms of flame retardancy, weight gain, and composition. A greater reduction in peak heat release rate has been achieved using the PEC coating (78% reduction for cotton, 31% for polyester) relative to the LbL deposited coating (45% for cotton, 19% for polyester). PAAm/polyphosphate complex coatings self-extinguish PECO fabric with less added weight (17.9%) than those assembled layer-by-layer (22.2%). Investigation into the possible cause for this improved FR effect revealed a significant difference in composition between coatings produced with these two techniques. The PEC coating proved to be extraordinarily durable to washing, maintaining flame retardancy after five home launderings and 8 h in boiling water. PEC coatings provide a powerful treatment to reduce the flammability of blended fabrics, using water-based ingredients and very few processing steps.
Experimental
Materials
Polyester–cotton fabric with a weight of 4.5 oz yd−2 (65% polyester) and 1993 AATCC standard detergent were purchased from Testfabrics, Inc (West Pittston, PA). Sodium hexametaphosphate (crystalline, +200 mesh, 96%) [PSP] was purchased from Sigma-Aldrich (Milwaukee, WI). Poly(allylamine hydrochloride) (Mw ∼ 15
000 g mol−1) [PAAm hydrochloride] was purchased from Beckmann-Kenko (Bassum, Germany) and used as received. Citric acid monohydrate and sodium citrate dihydrate were purchased from Sigma-Aldrich and used to make buffer solutions. 1 M NaOH made from pellets (anhydrous; reagent grade, ≥98%), and 5 M HCl, made from concentrated acid (ACS reagent, 37%), both purchased from Sigma-Aldrich, were used to adjust the pH of the deposition and treatment solutions. All individual solutions were prepared with 18.2 MΩ cm deionized water.
Preparation and deposition of PEC coating
PAAm and PSP were found to be stable in the same suspension at pH ≥ 12. Below this pH, the polyelectrolytes associate and flocculate from the suspension. The pKa of PAAm is 8.7, so its amine groups are largely unprotonated and the polyelectrolyte has very little positive charge at pH 12.32 Under these conditions there are only weak interactions between PAAm and polyphosphate, allowing for a stable polyelectrolyte suspension to form. In order to achieve high weight gain with a single dip, the PEC solution contained high polymer concentrations. PEC coating solutions were prepared using a 7
:
12 ratio of PAAm hydrochloride to PSP (near equimolar proportion of the two polymers by monomer units). The PEC suspension was prepared with 9.2 wt% PAAm hydrochloride and 15.8 wt% PSP (25 wt% solids in water). PAAm hydrochloride was dissolved in water, while an equimolar amount of NaOH was added to neutralize the hydrochloride. PSP was dissolved in a separate solution. The two solutions were added together and stirred vigorously to generate a pearlescent suspension. The pH of the stable suspension was ∼12. Medium and low concentration PEC suspensions were prepared according to the same procedure using lower polyelectrolyte concentrations. Medium concentration consisted of 7.4 wt% PAAm hydrochloride and 12.6 wt% PSP (20 wt% solids), while low concentration consisted of 5.5 wt% PAAm hydrochloride and 9.5 wt% PSP (15 wt% solids).
PECO fabric was dipped in a PEC solution for 30 s immediately after preparation. Fabric was then squeezed and hung to dry in a 70 °C oven for 1 h prior to buffer treatment. A pH 4 buffer solution was prepared using 100 mM citric acid/sodium citrate. Coated PECO was treated by soaking in 300 mL of the buffer solution for 5 min. The acidic environment of the buffer treatment protonated the PAAm contained in the coating. The positively-charged PAAm then strongly complexed with the anionic polyphosphate, forming an insoluble complex. The fabric was then rinsed vigorously in water to remove citrate and free polymer, then dried at 70 °C for 2 h prior to testing.
Layer-by-layer deposition
The LbL assembly of PAAm/PSP films was modified from an earlier literature procedure.21 2.0 wt% PSP and 1.0 wt% PAAm hydrochloride solutions, both at pH 4, were prepared with deionized water (18.2 MΩ) as separate deposition solutions. Multilayer nanocoatings were deposited on PECO fabric by dipping into the polyelectrolyte solution, alternating between cationic PAAm and anionic PSP, with each cycle corresponding to one bilayer (BL). The first dip into each mixture was for 5 min, beginning with cationic poly(allylamine). Subsequent dips were for 1 min each. Between dips, the fabric was wrung and rinsed in deionized water to remove excess polymer. After the desired number of bilayers were reached, the fabric was hung to dry for 2 h at 70 °C prior to testing.
General characterization
Coated and uncoated cotton substrates were mounted on aluminum stubs and sputter coated with 6 nm of platinum/palladium (Pt/Pd) alloy prior to imaging with a field-emission scanning electron microscope (FESEM, Model JSM-7500F, JEOL; Tokyo, Japan). XPS measurements were conducted using a PHI Quantera XPS (Chanhassen, MN), using a focused monochromatic Al Kα X-ray (1486.7 eV) source (using 50 W, 15 kV and a 200 μm diameter). The vacuum pressure during analysis was 5 × 10−8 torr. XPS survey scan spectra in the 0–1100 eV binding energy range were recorded in 0.5 eV steps, with a pass energy of 140.0 eV. pH was measured with a IntelliCAL pH electrode (Hach, Loveland, CO). Elemental analyses were performed by Midwest Microlab LLC (Indianapolis, IN).
Thermal stability, flammability, and combustibility of fabric
Thermal stability of uncoated and PEC-coated cotton fabric (approximately 30 mg) was evaluated in triplicate using a Q-50 thermogravimetric analyzer (TGA; TA Instruments, New Castle, DE), under a controlled heating ramp of 20 °C min−1, from ambient temperature up to 600 °C, with a sample purge flow of 60 mL s−1 air and a balance purge flow of 40 mL s−1 nitrogen. Fabric samples were cut into 8 × 30 cm strips that were vertically hung in a metal clamp within a model VC-2 vertical flame cabinet (Govmark, Farmingdale, NY) and vertical flame testing was performed according to ASTM D6413-08. All fabric was run in triplicate for microscale combustion calorimetry at a 1 °C sec−1 heating rate, from 150–550 °C, using method A of ASTM D7309 (pyrolysis under nitrogen) at the University of Dayton Research Institute (Dayton, OH). Home laundering was performed according to AATCC 135, however the water temperature was 30 °C using a Samsung 4 cubic foot top-load washer (purchased at Home Depot, College Station, TX). Coating durability was also tested by refluxing 5 g (23 × 10 cm) of fabric in 400 mL of water for 8 h.
Results and discussion
Flame retardant behavior
Polyester–cotton blended fabric was coated with poly(allylamine)–polyphosphate complexes of varying concentration in water. Similar to earlier reports of flame retardant complex coatings,27,28 sufficient weight gain on fabric was achieved with very few processing steps. High concentration polyelectrolyte complexes (HPEC) achieved the highest average coating weight gain of 17.9 wt%. Low and medium concentration complexes (LPEC and MPEC) achieved weight gain of 8.7% and 13.7%, respectively. These coatings maintained the whiteness of the fabric, although there was some increase in fabric stiffness. For direct comparison to multilayer coatings, PAAm/PSP multilayers were applied to PECO fabric using LbL assembly adapted from the procedure reported by Li et al.21 Fabric was alternately dipped into cationic PAAm and PSP solutions until the desired number of bilayers were deposited. 20, 25, and 30 BL achieved coatings of weight gain 12.3, 17.2, and 22.2%, respectively. Weight gain and vertical flame test results for all PECO samples are summarized in Table 1.
Table 1 Vertical flame test results of coated and uncoated PECO fabric
Coating system |
Sample |
Wt gain (%) |
Afterflame (s) |
Char length (cm) |
Residue (wt%) |
Control |
— |
— |
14 ± 2 |
Consumed |
45 ± 2 |
LbL |
20 BL |
12.3 ± 0.6 |
10 ± 4 |
Entire sample |
75 ± 4 |
25 BL |
17.2 ± 1.1 |
10 ± 3 |
Entire sample |
73 ± 5 |
30 BL |
22.2 ± 0.8 |
<1 |
16 ± 1 |
95 ± 3 |
PEC |
LPEC |
8.7 ± 0.2 |
20 ± 3 |
Entire sample |
61 ± 4 |
MPEC |
13.7 ± 0.3 |
Inconsistent |
Inconsistent |
Inconsistent |
HPEC |
17.9 ± 0.6 |
<1 |
16 ± 2 |
94 ± 2 |
The flame retardancy of LbL and PEC coatings was evaluated with vertical flame testing (VFT). Uncoated control fabric ignited and was almost completely consumed in an average of 14 s, leaving 45% residue from melted remains at the sides, with no visible charring. Both polyelectrolyte coating systems showed improved VFT performance by an evident charring mechanism. 20 and 25 BL PAAm/PSP coatings were able to produce significant char residue. 30 BL achieved sufficient weight gain (22.2%) to cause PECO to self-extinguish during burn testing, yielding a 16 cm char length. PEC coatings on PECO performed significantly better than the LbL coatings, with lower coating weight needed to impart self-extinguishing behavior. MPEC was able to inconsistently self-extinguish with only 13.7% coating weight, suggesting this was a borderline weight for effective flame retardancy. HPEC and 25 BL LbL coatings had the same weight gain (17%), but the polyelectrolyte complex (deposited in just two steps) consistently self-extinguished, while the 25 BL fabric burned over the entire sample. Fig. S1† shows representative images of each coated fabric after vertical flame testing.
To further investigate the flame retardancy of the coatings, pyrolysis-combustion flow calorimetry (PCFC) was used to obtain the heat release rate (HRR) of uncoated, PEC and LbL FR fabrics. HPEC and 25 BL coatings result in similar weight on PECO and both reduce the total heat release (THR) of the fabric by 30%, as shown in Fig. 2. This is due to the coatings' ability to promote the formation of char, reducing the amount of flammable material available for pyrolysis and heat release. Although both systems have the same reduction in THR, HPEC shows a greater reduction in peak HRR. The peak heat release rate is a measure of the maximum rate at which fabric releases heat during a fire. This released heat continues the combustion cycle, propagating fire (i.e., greater peak HRR correlates to greater material flammability). In addition to reducing the peak HRR, both coating systems decrease the temperature at which these peaks occur (Table 2).
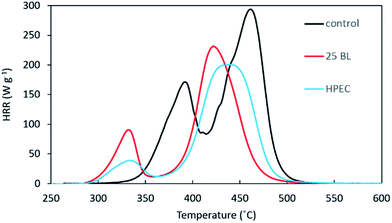 |
| Fig. 2 Heat release rate as a function of temperature for uncoated fabric and PECO coated with 25 PAAm/PSP bilayers or high concentration polyelectrolyte complex, as measured by pyrolysis-combustion flow calorimetry. | |
Table 2 Pyrolysis-combustion flow calorimetry results for uncoated and coated PECO fabric
Coating System |
Wt gain (%) |
PCFC |
VFT (Table 1) |
pHRR (W g−1) |
THR (kJ g−1) |
Control |
— |
170 at 392 °C |
15.6 ± 0.1 |
Burned |
294 at 461 °C |
25 BL |
17.2 ± 1.1 |
93 at 322 °C |
11.0 ± 0.1 |
Burned |
237 at 423 °C |
HPEC |
17.9 ± 0.6 |
38 at 334 °C |
10.9 ± 0.2 |
Self-extinguishing |
203 at 438 °C |
This earlier decomposition temperature is actually desirable, because it changes the decomposition pathway of the fabric. The first peak for uncoated PECO (Fig. 2) corresponds to cotton decomposition at 392 °C, while the larger second peak is from polyester (at 461 °C). The HPEC coating reduces the peak HRR of cotton by 78% and the peak HRR of polyester by 31%, both of which are greater reductions than those achieved by the 25 BL coating at 45% and 19%, respectively. The earlier decomposition caused by the coating is also observed during thermogravimetric analysis under both air and nitrogen as shown in Fig. S2 and S3,† respectively.
Coating morphology
Scanning electron microscopy was used to visualize the PAAm/PSP coatings on the polyester–cotton fabric, as shown in Fig. 3. The micrographs of both systems (HPEC and 25 BL) look very similar owing to their similar weight. Layer-by-layer deposition produces a conformal coating on the polyester–cotton fibers, maintaining the overall weave structure. Low solution concentration (≤2 wt% polyelectrolyte), and rinsing between layers, minimizes polyelectrolyte aggregation and fiber bridging with the LbL process. This process affords higher-quality nanocoatings, but requires a large number of processing steps (over 100 for 25 BL). The HPEC-coated fabric has a few more aggregated particles (Fig. 3), but appears nearly as good as the LbL-coated fabric and requires only two processing steps. SEM micrographs of the char after VFT testing (bottom images in Fig. 3) show that both FR coating systems preserve the weave of the fabric. Evidence of a bubbled intumescent charring mechanism can be observed in the char residues. Polyester can be observed as melted residue surrounding the fibers.
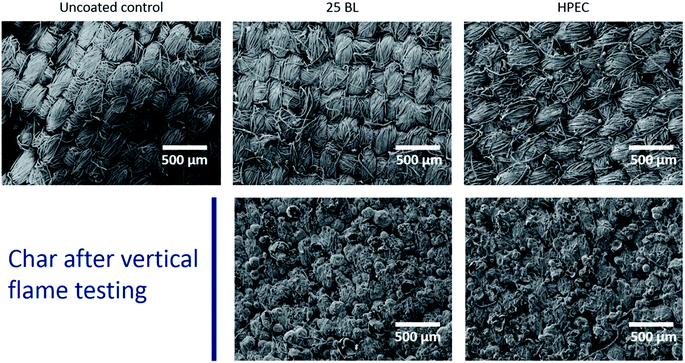 |
| Fig. 3 SEM micrographs of coated and uncoated PECO fabric. | |
Wash durability
Durability of the polyelectrolyte complex coating was demonstrated by laundering with detergent. No appreciable weight loss was observed for HPEC-coated PECO after five home-laundering cycles with ionic detergent. Additionally, much of the original softness and flexibility of the fabric was restored. After five home launderings, the samples were still able to self-extinguish during VFT, with a char length of 16 ± 2 cm and 90% residue. HPEC-coated PECO also maintained its flame retardant behavior after 8 h in boiling water. Although not fully understood, HPEC-coated fabrics lost on average 1.6 ± 0.1 wt% after boiling, but VFT performance improved, resulting in an average char length of 13 ± 2 cm. Fig. 4 shows HPEC coated fabric after vertical flame testing and washing. To quantify the wash-durability of the FR coating, PCFC curves of HPEC-coated fabric after laundering and boiling are compared in Fig. 4d. The HPEC coating maintains the greatest reduction in peak HRR relative to uncoated cotton and polyester after both washing procedures. After five home launderings, the cotton peak increased in HRR, while the polyester peak was reduced even further. The basic environment of detergent in water may have removed some of the coating on cotton, but the coating on polyester was maintained, largely preserving the FR effect of the PEC. Eight hours in boiling water did not affect the cotton peak HRR and further reduced the polyester peak. PCFC results are summarized in Table S1.† This is the first FR polyelectrolyte coating to show such impressive wash-durability on fabric, likely due to the insolubility of the polyelectrolyte complex, even at high solution pH.33
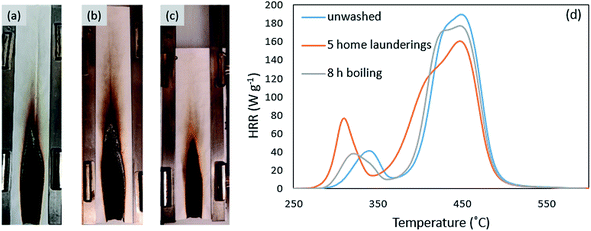 |
| Fig. 4 Representative VFT results of HPEC coated PECO fabric: (a) unwashed, (b) after five home launderings, and (c) after 8 h in boiling water. (d) Heat release rate as a function of temperature for washed and unwashed HPEC fabric, as measured by pyrolysis-combustion flow calorimetry. | |
Coating composition
Although both 25 BL and HPEC coatings have the same weight, the difference in FR performance is likely due to differences in composition. Phosphorus and nitrogen concentration are indicative of the amount of polyphosphate and PAAm in the coating, respectively. These concentrations were determined by elemental analysis and revealed differences between the two coating systems. A polyelectrolyte complex obtained from the simple mixing of dilute PSP and PAAm solutions (both at pH 4), had a molar phosphorus–nitrogen ratio (P/N) of 0.93, close to what would be expected for charge-neutral stoichiometric complexation (P/N = 1). HPEC-coated fabric had a P/N ratio of 0.74, which is a stoichiometric excess of PAAm units and in agreement with a prior study of complexes containing polyethylenimine and polyphosphate.27 This suggests the protonation of PAAm in the HPEC coating during buffer treatment was incomplete. In contrast, the P/N ratio of the LbL film was 1.26, indicative of a stoichiometric excess of polyphosphate, likely due to from incomplete charge neutralization during polymer adsorption.34 Table 3 summarizes elemental analysis of these systems.
Table 3 Elemental analysis of flame retardant coatings
|
Phosphorus (wt%) |
Nitrogen (wt%) |
P/N (mol mol−1) |
Na/Ca |
Na/C ratio obtained from X-ray photoelectron spectroscopy. |
25 BL |
3.11 ± 0.08 |
1.11 ± 0.04 |
1.26 ± 0.01 |
8.87 × 10−3 |
HPEC |
2.85 ± 0.08 |
1.74 ± 0.12 |
0.74 ± 0.03 |
4.90 × 10−3 |
From solution |
17.38 ± 0.04 |
8.45 ± 0.01 |
0.93 ± 0.00 |
— |
Polyphosphate in the films phosphorylate the C(6) unit of the glucose monomer, preventing cellulose pyrolysis and promoting char formation. As the active flame retardant ingredient, it is often proposed that higher phosphorus content leads to a greater FR effect.20,21,27 This makes the poorer flame retardant performance of the 25 BL system unexpected, given its greater phosphorus content relative to the self-extinguishing HPEC-coated PECO. While polyphosphate may function as an acid source for char formation during burning, it may function most efficiently when its surrounding environment contains protons. The availability of protons allows the polyphosphate to decompose into phosphoric acid, the charring catalyst in a fire. The FR performance of two polyphosphates with different counterions, ammonium (APP) and sodium (PSP), provides a useful demonstration of this concept. The protons available from the ammonium counterion allow APP to decompose at 150 °C, while aprotic PSP is stable all the way up to 500 °C, as shown in Fig. S4.†
When these polyphosphates were adsorbed on PECO fabric by wet pickup, similar weight gains were obtained (17%), but APP outperformed PSP as a flame retardant during VFT. PECO fabric coated with APP was able to self-extinguish, while PECO coated with PSP only left 33% char residue. Polyphosphate on the PSP-coated PECO has no available protons during burning. In contrast, the APP readily decomposes through the deprotonation of ammonium. In the case of the LbL and PEC films, the protonated amines of PAAm are an effective source of these protons for polyphosphate decomposition. The excess of PAAm in the HPEC coating means there are enough available protons to decompose polyphosphate completely, leading to more efficient char formation. The 25 BL films have a P/N ratio of 1.26, so there is only enough PAAm available in the film to protonate (at maximum) 79% of the polyphosphate units. The negative charge of the excess polyphosphate is neutralized by sodium ions, as suggested by the atomic composition determined by X-ray photoelectron spectroscopy (XPS). The sodium–carbon atomic ratio (Na/C) is much higher for 25 BL (8.87 × 10−3) than it is for PAAm/polyphosphate HPEC (4.90 × 10−3). The excess positive charge expected for the HPEC film may be neutralized by the negative surface charge of the cotton or residual citrate. No chloride was detected by elemental analysis in any of the films.
Conclusions
Polyester–cotton fabric was rendered flame retardant with a stable polyelectrolyte complex of poly(allylamine) and polyphosphate, which formed a wash durable coating. In addition to having far fewer processing steps than a multilayer coating using the same polymers, the PEC exhibited improved flame retardancy when adjusted for weight gain, owing to its higher nitrogen content contributing to the strong charring of the system. The high concentration complex was able to successfully maintain the whiteness and microscopic weave of the fabric, while also maintaining the hand after washing. With only 17.9% weight gain, HPEC-coated PECO was able to self-extinguish in VFT and reduce the peak HRR by 78% and 31% for the cotton and polyester, respectively. After five home launderings with detergent, this coated fabric maintained its flame retardant behavior, which is the first demonstration of laundering durability for polyelectrolyte complex coatings. This water-based process provides a powerful framework to apply durable films to textiles of high commercial interest.
Acknowledgements
The authors would like to thank Dr Alexander Morgan at the University of Dayton Research Institute for pyrolysis-combustion flow calorimeter testing and helpful discussions. The authors further acknowledge the Texas A&M Engineering Experiment Station (TEES) and the Microscopy and Imaging Center (MIC) for infrastructural support of this work.
References
- S. Shui and A. Plastina, World Apparel Fiber Consumption Survey, International Cotton Advisory Committee, Washington DC, 2013 Search PubMed.
- M. Day, T. Suprunchuk and M. Wiles, in Fire Safety Science: Proceedings of the First International Symposium, ed. P. J. P. C. E. Grant, Hemisphere Publishing Corporation, Taylor & Francis, 1986, pp. 401–402 Search PubMed.
- J. Hoebel, G. Damant, S. Spivak and G. Berlin, Fire Technol., 2010, 46, 765 CrossRef.
- C. Jarvis and R. Barker, in Flame – Retardant Polymeric Materials, ed. M. Lewin, S. M. Atlas and E. M. Pearce, Springer, US, 1978, ch. 5, pp. 133–158, DOI:10.1007/978-1-4684-6973-8_5.
- A. R. Horrocks, M. Y. Wang, M. E. Hall, F. Sunmonu and J. S. Pearson, Polym. Int., 2000, 49, 1079–1091 CrossRef CAS.
- E. D. Weil and S. V. Levchik, J. Fire Sci., 2008, 26, 243–281 CrossRef CAS.
- A. R. Horrocks and B. K. Kandola, in Plastics Flammability Handbook: Principles, Regulations, Testing, and Approval, ed. J. Troitzsch, Hanser Gardener Publications, Cincinnati, 3rd edn, 2004, ch. 6.3, pp. 182–186 Search PubMed.
- C. Drevelle, J. Lefebvre, S. Duquesne, M. Le Bras, F. Poutch, M. Vouters and C. Magniez, Polym. Degrad. Stab., 2005, 88, 130–137 CrossRef CAS.
- M. Lewin and S. B. Sello, Handbook of Fiber Science and Technology: Functional Finishes. Chemical processing of fibers and fabrics, Taylor & Francis, 1984 Search PubMed.
- H. Yang and C. Q. Yang, Polym. Degrad. Stab., 2005, 88, 363–370 CrossRef CAS.
- C. A. de Wit, Chemosphere, 2002, 46, 583–624 CrossRef CAS PubMed.
- Y. R. Kim, F. A. Harden, L.-M. L. Toms and R. E. Norman, Chemosphere, 2014, 106, 1–19 CrossRef CAS PubMed.
- J. Alongi, F. Carosio and G. Malucelli, Polym. Degrad. Stab., 2014, 106, 138–149 CrossRef CAS.
- S. Bourbigot, M. Le Bras, S. Duquesne and M. Rochery, Macromol. Mater. Eng., 2004, 289, 499–511 CrossRef CAS.
- A. B. Morgan and J. W. Gilman, Fire Mater., 2013, 37, 259–279 CrossRef CAS.
- J. Alongi and G. Malucelli, RSC Adv., 2015, 5, 24239–24263 RSC.
- F. Carosio, J. Alongi and G. Malucelli, Carbohydr. Polym., 2012, 88, 1460–1469 CrossRef CAS.
- F. Carosio, G. Laufer, J. Alongi, G. Camino and J. C. Grunlan, Polym. Degrad. Stab., 2011, 96, 745–750 CrossRef CAS.
- T. Zhang, H. Yan, L. Wang and Z. Fang, Ind. Eng. Chem. Res., 2013, 52, 6138–6146 CrossRef CAS.
- G. Laufer, C. Kirkland, A. B. Morgan and J. C. Grunlan, Biomacromolecules, 2012, 13, 2843–2848 CrossRef CAS PubMed.
- Y.-C. Li, S. Mannen, A. B. Morgan, S. Chang, Y.-H. Yang, B. Condon and J. C. Grunlan, Adv. Mater., 2011, 23, 3926–3931 CrossRef CAS PubMed.
- Y.-C. Li, J. Schulz, S. Mannen, C. Delhom, B. Condon, S. Chang, M. Zammarano and J. C. Grunlan, ACS Nano, 2010, 4, 3325–3337 CrossRef CAS PubMed.
- X. Wang, M. Q. Romero, X.-Q. Zhang, R. Wang and D.-Y. Wang, RSC Adv., 2015, 5, 10647–10655 RSC.
- J. Borges and J. F. Mano, Chem. Rev., 2014, 114, 8883–8942 CrossRef CAS PubMed.
- G. Decher and J. B. Schlenoff, Multilayer thin films: sequential assembly of nanocomposite materials, Wiley-VCH, Weinheim, 2nd edn, 2012 Search PubMed.
- P. T. Hammond, Adv. Mater., 2004, 16, 1271–1293 CrossRef CAS.
- M. Haile, C. Fincher, S. Fomete and J. C. Grunlan, Polym. Degrad. Stab., 2015, 114, 60–64 CrossRef CAS.
- M. Leistner, M. Haile, S. Rohmer, A. Abu-Odeh and J. C. Grunlan, Polym. Degrad. Stab., 2015, 122, 1–7 CrossRef CAS.
- N. Acar, M. B. Huglin and T. Tulun, Polymer, 1999, 40, 6429–6435 CrossRef CAS.
- A. Shovsky, I. Varga, R. Makuska and P. M. Claesson, Langmuir, 2009, 25, 6113–6121 CrossRef CAS PubMed.
- S. A. Sukhishvili, E. Kharlampieva and V. Izumrudov, Macromolecules, 2006, 39, 8873–8881 CrossRef CAS.
- M. Fang, C. H. Kim, G. B. Saupe, H.-N. Kim, C. C. Waraksa, T. Miwa, A. Fujishima and T. E. Mallouk, Chem. Mater., 1999, 11, 1526–1532 CrossRef CAS.
- F. Carosio and J. Alongi, RSC Adv., 2015, 5, 71482–71490 RSC.
- A. Vanerek and T. G. M. van de Ven, Colloids Surf., A, 2006, 273, 55–62 CrossRef CAS.
Footnote |
† Electronic supplementary information (ESI) available. See DOI: 10.1039/c6ra03637f |
|
This journal is © The Royal Society of Chemistry 2016 |