Open Access Article
DOI:
10.1039/C3PY21104E
(Paper)
Polym. Chem., 2013,
4, 2608-2614
Dual-functional materials via CCTP and selective orthogonal thiol-Michael addition/epoxide ring opening reactions†
Received
14th December 2012
, Accepted 12th January 2013
First published on 14th January 2013
Abstract
Poly(glycidyl methacrylate) (PGMA) has been synthesised by cobalt catalysed chain transfer polymerisation (CCTP) yielding, in one step, polymers with two points for post polymerisation functionalisation; the activated terminal vinyl bond and in chain epoxide groups. Epoxide ring-opening and a combination of thiol-Michael addition and epoxide ring-opening has been used for the post-functionalisation with amines and thiols to prepare a range of functional materials.
Introduction
Epoxides are a highly reactive functional group arising from high ring strain of the three-membered ring.1 This strained ring system ring-opens by reaction with a wide range of nucleophiles.2 This provides a facile route to the synthesis of functional materials. Commercial realisation that epoxide containing polymers, such as glycidyl methacrylate (GMA), could undergo such post polymerisation modifications occurred in the mid 1950's,3 whilst academic research in this field emerged in the 1960's.4–7
There has been a resurgence in the interest involving epoxide polymers, from reactive surfaces and functional particles,8–19 through to polymer chain end modifications with small molecule epoxides.20,21 In particular several research groups have used GMA as an alternative method to introduce azide groups along the polymer backbone.22,23 Tsarevsky et al. synthesised copolymers of GMA and methyl methacrylate (MMA) via atom transfer radical polymerisation (ATRP), yielding bromine terminated polymers containing epoxides.24 Several functionalisation steps led to the formation of graft copolymers via the reaction of epoxide and terminal bromine groups with sodium azide; the subsequent copper catalysed azide alkyne cycloaddition reaction (CuAAC) with alkyne terminated polymers yielding loosely grafted polymer brushes.25 Recently published work, by our group, reported the CCTP of GMA followed by epoxide ring opening with sodium azide yielding azide-functional polymers with vinylic groups at the chain end; subsequent reaction of the azide with synthesised sugar alkynes, yielded glycopolymers with ω-unsaturated end groups.
Khan et al. has reported the functionalisation of an epoxy backbone generated via free radical polymerisation and in more recent work copolymers. In this case, lithium hydroxide was implemented as a catalyst to facilitate the ring-opening of epoxide groups with functional thiols. To introduce additional functionality at the same polymer unit, subsequent reactions at the hydroxyl groups, generated during the ring-opening, were carried out via esterification, creating dual functionalised polymers.26,27
The scope for post polymerisation functionalisation has proven to be fruitful for epoxide containing polymers, and many combinations of polymerisations and functionalisations remain unexplored. The focus for this current work is the functionalisation of GMA synthesised by CCTP via epoxide ring-opening with both primary and secondary amines. One of the advantages of using amines is due to their strong nucleophilic and basic nature, the majority of primary and secondary amines can take part in epoxide ring-opening reactions without the use of a catalyst, also there is a wealth of primary and secondary amines available commercially. Additionally by employing CCT as the polymerisation method, the molecular weight of the polymer can be controlled with high conversion and a ω-unsaturated end group is incorporated into the polymer, providing a second reactive handle for functionalisation, either by thiol–ene chemistry28–32 or nucleophilic attack by functional primary amines. By tuning the selectivity of the reactions we have been able to optimise and generate dual functional polymers (at the terminus and along the polymer backbone) which would be difficult to obtain by other methods (Scheme 1).
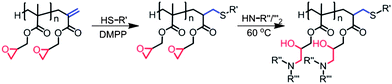 |
| Scheme 1 Dual functionalisation of poly-GMA via thiol-Michael addition to vinyl groups and epoxide ring-opening with functional amines. | |
Results and discussion
Synthesis of poly(glycidyl methacrylate)
Linear poly-GMA was synthesised via CCTP using low levels of bis(boron difluorodimethylglyoximate) (CoBF) as a chain transfer agent. CCTP of methacrylates leads to terminal vinyl group formation which is favoured over in-chain double bonds, Scheme 2. Measurement of the chain transfer efficiency (Cs) of CoBF in acetonitrile GMA gave a value of 6400 (see ESI†). Variation of the concentration of catalyst provides a high level of control over the molecular weight, with increasing catalyst concentration yielding lower molecular weight products, Table 1.33–35
Table 1 Poly-GMA polymers synthesised in this work
Name |
[CoBF : GMA] (mol) |
M
n (g mol−1) |
M
w (g mol−1) |
PDi |
Conversion (%) |
A |
[1 : 98k] |
800 |
1400 |
1.76 |
90.2 |
B |
[1 : 195k] |
1600 |
2900 |
1.86 |
91.2 |
C |
[1 : 391k] |
3100 |
5800 |
1.86 |
99.0 |
D |
[1 : 783k] |
5000 |
9100 |
1.84 |
93.4 |
Functionalisation of poly-GMA (B) with primary amines
CCTP synthesised poly-GMA possesses two potential sites for functionalisation; each polymer chain contains a single terminal vinyl group and epoxide functionality equal to the DPn, Scheme 3. To assess the activity of these two groups a series of reactions were conducted with poly-GMA (B) with n-propylamine under ambient conditions, with the addition of a small excess of propylamine (1.2 excess) in a variety of polar aprotic solvents, Table 2. Under these conditions it was found that the vinyl groups are more susceptible to reaction with propylamine than the epoxide, Scheme 3, which although somewhat surprising considering the ring strain of the epoxide group, has been previously stated in work by Zhang et al.22 Polar aprotic solvents are good solvents for SN2 ring-opening reactions yet under these conditions ring-opening of the epoxide is slow (conversion increases slowly with increasing solvent polarity) and within the time frame of 70 h does not reach high conversion in any solvent used. Hence, for complete functionalisation at both the epoxide and vinyl terminal group larger excesses of amine were employed in DMSO which provides a good solvent for a wide range of both hydrophobic and hydrophilic primary amine reagents.
Solvent |
Conversion of epoxide |
Conversion of vinyl group |
MEK |
0% |
32% |
EtOAc |
0% |
65% |
DCM |
24% |
100% |
ACN |
35% |
31% |
DMSO |
58% |
100% |
DMF |
64% |
100% |
Ring-opening reactions between poly-GMA and propylamine were carried out using 2-fold, 5-fold and 10-fold excesses of amine relative to epoxide groups, Fig. 1. Conversion of both vinyl and epoxide were measured by 1H NMR over the course of 70 hours. Using a 10-fold excess of amine, nucleophilic addition to vinyl groups is complete within 1 hour at ambient temperature, with a slower rate of reaction observed for epoxide ring-opening, which reached 100% conversion within 25 hours. In the cases of 5-fold and 2-fold excess of amine the same trend is observed but the rate of reaction for functionalisation of both epoxide and vinyl groups is reduced, with the 2-fold excess rate being the slowest. An important observation of these reactions was that in cases where lower amine excesses were used (such as 3-fold excess and below, at ambient temperatures) the products became insoluble/sparingly soluble after several days. This is most likely caused by the use of a primary amine in the ring-opening reaction undergoing inter-molecular crosslinking when lower excesses of amine are used at ambient temperatures.
In order to reduce the excess of amine used and decrease reaction time, ring-opening reactions were conducted at 60 °C with a 1.2-fold excess of amine, unless otherwise stated. 1H NMR confirms the reactions proceeded to high conversion within 6 hours for primary amine functionalisation, but on termination of reactions at this time the products undergo inter-molecular crosslinking; to avoid this all reactions were conducted over 24 hours. A comparison of the 1H NMR spectra for propylamine functionalised and unfunctionalised poly-GMA is given in Fig. 2. Reactions of propargyl amine and decylamine led to the formation of gels and crosslinking respectively, on use of a 1.2 excess of amine, hence a higher excess of 5-fold was employed, which circumvented crosslinking. It is also possible that a certain amount of intra-molecular crosslinking occurs, but this would not lead to insoluble products and would be difficult to spectroscopically distinguish from inter-molecular reactions.
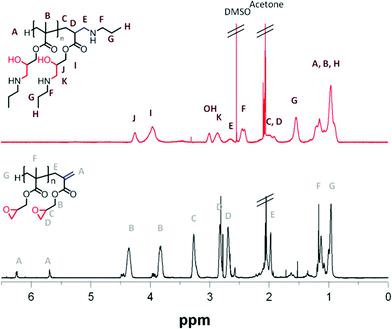 |
| Fig. 2
1H NMR comparison of propylamine functionalised poly-GMA (B) (red trace, top) and unfunctionalised poly-GMA (B) (black trace, bottom) | |
GPC data shows an increase in molecular weight upon reaction of poly-GMA with primary amines, GPC eluent was chosen based on the compatibility of both the unfunctionalised and functionalised poly-GMA, as in the case of ring-opening with decylamine the functionalised polymer was soluble only in hydrophobic solvents, limiting the choice for GPC analysis. Conversely ring-opening with aminopropanediol yielded a polymer only soluble in hydrophilic solvents (Table 3). The observed increase in molecular weight and PDi is most likely due to the change in hydrophobicity/hydrophilicity and the effect on hydrodynamic volume.
Table 3 GPC data of products of epoxide ring-opening of poly-GMA (B) with a range of functional primary amines
Functional amine |
M
n
(g mol−1) |
M
w (g mol−1) |
PDi |
Calibrated against PMMA standards.
|
DMF GPC eluent
|
Unfunctionalised P-GMA B |
2800 |
4400 |
1.6 |
Propylamine |
6200 |
8700 |
1.4 |
Benzylamine |
7500 |
15 400 |
2.0 |
Aminopropanediol |
5600 |
10 600 |
1.9 |
Propargylamine |
12 700 |
18 100 |
1.4 |
|
CHCl
3
GPC eluent
|
Unfunctionalised P-GMA B |
2000 |
4100 |
2.1 |
Decylamine |
4100 |
6200 |
1.4 |
Ethylene diamine |
Crosslinked particles |
Ring-opening of the epoxide and addition of functional amines was also observed via1H NMR, whereby peaks corresponding to the epoxide at 2.6, 2.7 and 3.15 ppm shifted towards low field, with no discernible epoxide peaks remaining. Peaks at 3.8 and 4.3 ppm arising from the constrained OCH2 adjacent to the epoxide converge on ring-opening, indicating a release of constraint. Peaks corresponding to the amine reactants are also observed confirming the incorporation of the functional amines to the polymer (see ESI†). A disappearance of vinyl peaks was also observed via1H NMR and the introduction of a peak at approximately 2.5 ppm indicates the formation of CH2NH. When ethylene diamine was employed the product precipitated out of solution with 100% of epoxide groups ring-opening to form crosslinked particles (ESI†). Polymers were also investigated by MALDI-ToF spectroscopy, major products observed correspond to fully functionalised poly-GMA (both epoxide and vinyl groups) and poly-GMA with one group per chain remaining unfunctionalised, a typical MALDI-ToF spectrum is shown in Fig. 3.
Functionalisation of poly-GMA with secondary amines
Functionalisation of poly-GMA with secondary amines has the advantage over using primary amines in that crosslinking of epoxide groups with tertiary amine chains formed cannot occur, allowing for reactions times to be shortened to around 12 hours. For shorter reaction times however, gelation is still observed, either through primary amine impurities in the reagents or epoxide–epoxide ring-opening reactions. Secondary amines show a reduced activity in the reaction with terminal vinyl groups on the polymer in comparison to primary amines. Ambient temperature experiments using various excesses of secondary amine were conducted to assess the reactivity of both the epoxide and vinyl groups of poly-GMA (B) to nucleophilic attack with diethylamine, Fig. 4.
Reaction of secondary amines with epoxides is slower than primary amines and reaction of vinyl groups with secondary amines is low, with conversions of only 5–15% observed over 48 hours, Fig. 4, whereas in the case of primary amine reactions the vinyl groups proved more reactive to amine attack than epoxides, Fig. 1. Due to the low activity of vinyl groups with secondary amines functionalisation of the vinyl groups can be conducted prior to or post ring-opening, Scheme 4.
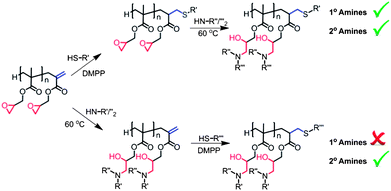 |
| Scheme 4 Alternative dual functionalisation routes for use of primary and secondary amines. | |
Reaction of poly-GMA (B) with secondary amines, diethylamine, diethanolamine and diphenylamine were carried out at 60 °C in DMSO with a 1.2 excess of amine. Diethylamine and diethanolamine were successful, showing a shift to higher molecular weight via GPC, Table 4, and were characterised by 1H NMR and MALDI-ToF. Products yielded contained no discernible epoxide signals via1H NMR with retention of a high level (∼90%) of vinyl functionality. Reaction of poly-GMA (B) with diphenylamine shows a low level of epoxide ring-opening of approximately 6% via1H NMR. As with SN2 reactions the steric bulk of the phenyl functionality will block nucleophilic attack at the side opposite to the leaving group hence reactivity is reduced. Reaction of poly-GMA (B) under the same conditions with 2-anilinoethanol was attempted with approximately 15% conversion of epoxide groups observed. Reaction of poly-GMA (B) with dibenzylamine was attempted as it was postulated that the CH2 groups between the amine and phenyl ring may reduce the steric hindrance, although a low level of approximately 35% amine addition was observed, the majority of epoxide peaks remained, indicating limitations in the functionalisation of these materials using hindered secondary amines.
Functional amine |
M
n (g mol−1) |
M
w (g mol−1) |
PDi |
Unfunctionalised P-GMA B |
2800 |
4400 |
1.6 |
Diethylamine |
8600 |
13 600 |
1.6 |
Diethanolamine |
6100 |
10 500 |
1.7 |
Diphenylamine |
3500 |
5600 |
1.6 |
2-Anilinoethanol |
3500 |
5300 |
1.5 |
Dibenzylamine |
3700 |
6400 |
1.7 |
Dual functionalisation of poly-GMA
Due to the non-selective reactivity of primary amines with both epoxides and terminal vinyl groups on poly-GMA, on use of primary amines the thiol-Michael addition was conducted prior to the epoxide ring-opening reaction, Scheme 1. Reactions were conducted in DMSO, unless stated, using dimethylphenylphosphine (DMPP) as a catalyst, under ambient conditions. Reaction of benzyl mercaptan via thiol-Michael addition was successful, with a complete loss of vinyl peaks and the appearance of a peak at 2.35 ppm corresponding to hydrothiolation of the vinyl group. No reaction of epoxide groups were observed by 1H NMR, hence selectivity of the thiol-Michael addition was upheld. GPC with refractive index (RI) and photodiode array (PDA) detection showed an increase in molecular weight from RI detection on functionalisation with benzyl mercaptan with a signal at λ = 270 nm observed corresponding to the polymer elution time (∼17 minutes), indicating successful functionalisation of poly-GMA (A) with benzyl mercaptan, Fig. 5. Thiol-Michael addition was also confirmed by MALDI-ToF, with the main peak series denoting increasing molecular weight of poly-GMA with each peak showing the addition of a single benzyl mercaptan per chain (an increase of 124.20 Daltons), whilst giving a single distribution of peaks with a mass difference of 142 Daltons corresponding to the repeat unit of GMA (see ESI†).
Epoxide ring-opening of benzyl mercaptan functionalised poly-GMA was conducted with propylamine, it is noted that no workup between thiol-Michael addition and ring-opening reactions is necessary. Successful epoxide ring-opening was confirmed by 1H NMR. Due to the change in hydrophobicity of the polymer on ring-opening of the epoxide, GPC analysis had to be conducted in DMF rather than THF. DMF is a poor solvent for the thiol-Michael addition product due to the addition of benzyl mercaptan to the polymer, hence peak tailing is observed. Increases in molecular weight are observed for both the benzyl mercaptan thiol-Michael addition product and dual functionalised product with epoxide ring-opening with propylamine, Fig. 6. Addition of propylamine to the polymer was also confirmed by MALDI-ToF, with the major peak series denoting increasing molecular weight of poly-GMA (A), functionalised with a single benzyl mercaptan unit per chain and full epoxide ring-opening with propylamine. Minor peaks correspond to chains functionalised with two benzyl mercaptan units and zero benzyl mercaptan units, but full functionalisation with propylamine, Fig. 7. The benzyl mercaptan thiol-Michael addition poly-GMA (A) was also successfully ring-opened with secondary amine diethylamine, confirmed by 1H NMR, GPC and MALDI-ToF (see ESI†), hence dual functionalisation of CCTP poly-GMA can be carried out in a site selective manner by conducting the thiol-Michael addition prior to the epoxide ring-opening reaction with both primary and secondary functional amines, Scheme 1.
Alternatively, as the retention of a high level of vinyl functionality is observed on conducting epoxide ring-opening reactions with secondary amines; more versatility can be achieved in the dual functionalisation of poly-GMA, as both reactions provide site selective functionalisation, hence, the order in which thiol-Michael addition and epoxide ring-opening reactions are performed is irrelevant for secondary amine use, Scheme 4. Poly-GMA (B) underwent epoxide ring-opening with secondary amine, diethylamine, with retention of a high level of vinyl groups confirmed by 1H NMR. This ring-opened product was then reacted via phosphine mediated thiol-Michael addition with benzyl mercaptan to yield a dual functionalised poly-GMA, confirmed by 1H NMR. MALDI-ToF analysis of the dual functionalised product indicated the main species corresponds to poly-GMA with a single benzyl mercaptan per chain and complete functionalisation with secondary amine diethylamine, as shown in Fig. 8; no evidence of poly-GMA fully functionalised with diethylamine or multiple attachments of benzyl mercaptan are observed, indicating a high purity of the product. GPC with UV detection also confirms the addition of a chromophore to the polymer, and an increase in molecular weight is observed at each functionalisation step, via RI detection, Fig. 9.
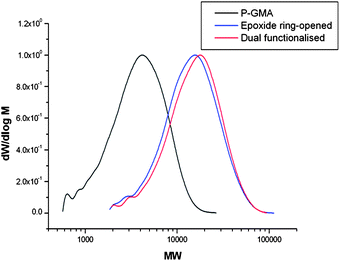 |
| Fig. 9 GPC spectra (DMF eluent) of unfunctionalised poly-GMA (B) (black trace), epoxide ring-opening with diethylamine (blue trace) and the dual functionalisation of poly-GMA (B), epoxide ring-opening with diethylamine and thiol-Michael addition with benzyl mercaptan (red trace). | |
Conclusions
Epoxide containing polymers were synthesised via CCTP of glycidyl methacrylate, with control of molecular weight accessed by increasing or decreasing the CoBF catalyst concentration. Epoxide containing polymers were subsequently functionalised via several routes; full functionalisation with primary amines led to the functionalisation of both epoxide and vinyl groups, whereas functionalisation with secondary amines retained high levels of vinyl functionality whilst obtaining full amine addition to epoxide groups. Dependent on whether functionalisation with primary or secondary amines was desired; combinations of thiol-Michael addition to terminal vinyl groups and epoxide ring-opening with commercially available amines could be conducted; providing several routes to the dual functionalisation of these polymers.
The synthesis and functionalisation of poly-GMA via CCTP and selective orthogonal thiol-Michael addition/epoxide ring opening reactions yielded a range of functional polymers with drastically altered hydrophobicity/hydrophilicity in very few synthetic steps. Using this strategy there is no requirement to use protecting groups during the selective chemical modification steps providing a facile route with scope for synthesis and functionalisation of a vast array of polymers. Furthermore, there remains the capacity to carry out esterification reactions on the hydroxyl groups generated during the ring opening reactions providing a third point for chemical modification.
Acknowledgements
Equipment used was supported by the Innovative Uses for Advanced Materials in the Modern World (AM2), with support from Advantage West Midlands (AWM) and part funded by the European Regional Development Fund (ERDF). DMH is a Royal-Society/Wolfson Fellow. KM acknowledges the EPSRC and Polymer Laboratories (Agilent) for funding, SS thanks Unilever for funding.
Notes and references
- H. C. Kolb, M. G. Finn and K. B. Sharpless, Angew. Chem., Int. Ed., 2001, 40, 2004–2021 CrossRef CAS.
-
J. Clayden, N. Greeves, S. Warren and P. Wothers, Organic Chemistry, Oxford University Press, Oxford, 2001 Search PubMed.
-
E. I. Dupont de Numours, Chemical Products, GB Patent 740
720 , 1952 Search PubMed.
- Y. Iwakura, T. Kurosaki and N. Nakabayashi, Die Makromolekulare Chemie, 1961, 44, 570–590 CrossRef.
- Y. Iwakura, T. Kurosaki, N. Ariga and T. Ito, Die Makromolekulare Chemie, 1966, 97, 128–138 CrossRef CAS.
- Y. Iwakura, T. Kurosaki and Y. Imai, Die Makromolekulare Chemie, 1965, 86, 73–79 CrossRef CAS.
- J. Kalal, F. Švec and V. Maroušek, J. Polym. Sci., Polym. Symp., 1974, 47, 155–166 CrossRef CAS.
- S. Wang, L. Shao, Z. Song, J. Zhao and Y. Feng, J. Appl. Polym. Sci., 2012, 124, 4827–4837 CAS.
- A. K. Ekenseair, K. W. M. Boere, S. N. Tzouanas, T. N. Vo, F. K. Kasper and A. G. Mikos, Biomacromolecules, 2012, 13, 1908–1915 CrossRef CAS.
- D. Wei, R. Zhou, Y. Guan, A. Zheng and Y. Zhang, J. Appl. Polym. Sci., 2013, 127, 666–674 CrossRef CAS.
- P. Zhao, Y. Yan, X. Feng, L. Liu, C. Wang and Y. Chen, Polymer, 2012, 53, 1992–2000 CrossRef CAS.
- Y. Bondar and D. Han, Russ. J. Appl. Chem., 2012, 85, 272–276 CrossRef CAS.
- D. Sung, S. Park and S. Jon, Langmuir, 2012, 28, 4507–4514 CrossRef CAS.
- R. Barbey, E. Kauffmann, M. Ehrat and H.-A. Klok, Biomacromolecules, 2010, 11, 3467–3479 CrossRef CAS.
- R. Barbey and H.-A. Klok, Langmuir, 2010, 26, 18219–18230 CrossRef CAS.
- S. B. Rahane, R. M. Hensarling, B. J. Sparks, C. M. Stafford and D. L. Patton, J. Mater. Chem., 2012, 22, 932–943 RSC.
- A. E. v. d. Ende, E. J. Kravitz and E. Harth, J. Am. Chem. Soc., 2008, 130, 8706–8713 CrossRef.
- A. I. Abdelrahman, S. C. Thickett, Y. Liang, O. Ornatsky, V. Baranov and M. A. Winnik, Macromolecules, 2011, 44, 4801–4813 CrossRef CAS.
- J. L. de la Fuente, P. F. Cañamero and M. Fernández-García, J. Polym. Sci., Part A: Polym. Chem., 2006, 44, 1807–1816 CrossRef CAS.
- M. A. Harvison, T. P. Davis and A. B. Lowe, Polym. Chem., 2011, 2, 1347–1354 RSC.
- G. C. Sanders, B. G. P. van Ravensteijn, R. Duchateau and J. P. A. Heuts, Polym. Chem., 2012, 3, 2200–2208 RSC.
- Q. Zhang, S. Slavin, M. W. Jones, A. J. Haddleton and D. M. Haddleton, Polym. Chem., 2012, 3, 1016 RSC.
- C. Li, Z. Ge, J. Fang and S. Liu, Macromolecules, 2009, 42, 2916–2924 CrossRef CAS.
- N. V. Tsarevsky, S. A. Bencherif and K. Matyjaszewski, Macromolecules, 2007, 40, 4439–4445 CrossRef CAS.
- N. V. Tsarevsky and W. Jakubowski, J. Polym. Sci., Part A: Polym. Chem., 2011, 49, 918–925 CrossRef CAS.
- S. De and A. Khan, Chem. Commun., 2012, 48 RSC.
- S. De, C. Stelzer and A. Khan, Polym. Chem., 2012, 3, 2342–2345 RSC.
- G. Z. Li, R. K. Randev, A. H. Soeriyadi, G. Rees, C. Boyer, Z. Tong, T. P. Davis, C. R. Becer and D. M. Haddleton, Polym. Chem., 2010, 1, 1196–1204 RSC.
- A. H. Soeriyadi, G. Z. Li, S. Slavin, M. W. Jones, C. M. Amos, C. R. Becer, M. R. Whittaker, D. M. Haddleton, C. Boyer and T. P. Davis, Polym. Chem., 2011, 2, 815–822 RSC.
- A. H. Soeriyadi, C. Boyer, J. Burns, C. R. Becer, M. R. Whittaker, D. M. Haddleton and T. P. Davis, Chem. Commun., 2010, 46, 6338–6340 RSC.
- A. B. Lowe, Polym. Chem., 2010, 1, 17–36 RSC.
- C. E. Hoyle and C. N. Bowman, Angew. Chem., Int. Ed., 2010, 49, 1540–1573 CrossRef CAS.
- A. A. Gridnev, J. Polym. Sci., Part A: Polym. Chem., 2000, 38, 1753–1766 CrossRef CAS.
- J. P. A. Heuts, G. E. Roberts and J. D. Biasutti, Aust. J. Chem., 2002, 55, 381–398 CrossRef CAS.
-
S. Slavin, K. A. McEwan and D. M. Haddleton, in Polymer Science: a Comprehensive Reference, ed. K. Matyjaszewski and M. Möller, Elsevier, 2012, pp. 249–275 Search PubMed.
Footnote |
† Electronic supplementary information (ESI) available: Full experimental details. See DOI: 10.1039/c3py21104e |
|
This journal is © The Royal Society of Chemistry 2013 |