DOI:
10.1039/D3RA07282G
(Paper)
RSC Adv., 2024,
14, 154-159
Electrochemical oxidative cyclization of N-allylamides for the synthesis of CF3-containing benzoxazines and oxazolines†
Received
25th October 2023
, Accepted 7th December 2023
First published on 2nd January 2024
Abstract
The introduction of trifluoromethyl (–CF3) groups into compounds is a common synthetic strategy in organic chemistry. Commonly used methods for introducing trifluoromethyl groups are limited by harsh reaction conditions, low regioselectivity, or the need for excess reagents. In this study, a facile electrochemical oxidative and radical cascade cyclization of N-(2-vinylphenyl)amides for the synthesis of CF3-containing benzoxazines and oxazolines was obtained. This sustainable protocol features inexpensive and durable electrodes, a wide range of substrates, diverse functional group compatibility under transition-metal-free, external-oxidant-free, and additive-free conditions, and can be applied in an open environment.
Introduction
Heterocyclic compounds are one of the most important skeletons in organic synthesis, pharmaceutical chemistry, materials science and bioscience. Heterocycles containing N and O atoms play a crucial role in pharmaceuticals and functional molecules.1 Among these, benzoxazines and oxazolines are common privileged fragments frequently found in pharmaceutical molecules and biologically active compounds with remarkable biological activities,2 such as anxiolytic,3 anti-HIV,4 progesterone receptor agonist,5 anti-tuberculosis,6 and anorectant activities7(Fig. 1).
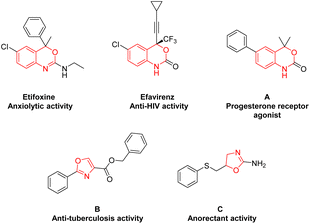 |
| Fig. 1 Bioactive compounds containing benzoxazine or oxazoline motifs. | |
Generally, the incorporation of fluorinated moieties into molecules can significantly change their physical, chemical, and biological properties. For example, the trifluoromethyl (–CF3) moiety is widely present in a variety of drugs (celecoxib, fluoxetine, and trifloxystrobin etc.), which can improve the efficacy, solubility, lipophilicity, metabolic stability, and binding selectivity.8 Efavirenz containing trifluoromethyl benzoxazine shows potent anti-HIV activity.4 As a result, the potential values of these trifluoromethylated benzoxazines and oxazolines have attracted significant attention from chemists to develop efficient strategies for the construction of these intriguing molecule scaffolds.9 Xiao and co-workers reported a visible-light-induced photocatalytic trifluoromethylation of N-allylamides for the synthesis of CF3-containing benzoxazines and oxazolines under Umemoto's reagent and Ru(bpy)3(PF6)2 (Scheme 1a).9m Similarly, Kumar's group developed a copper-catalyzed approach for construction of trifluoromethylated benzoxazines by using Umemoto's reagent (Scheme 1b).10 These methods are effective and versatile, but are limited to transition-metal catalysts and Umemoto's reagent as CF3 sources. In addition, Natarajan and colleagues disclosed a novel 9,10-phenanthrenedione visible-light photocatalysis protocol for the synthesis of trifluoromethylated benzoxazines by using N-(2-vinylphenyl)amides and trifluoromethylsulfinate under oxygen atmosphere (Scheme 1c).9a Nevertheless, it still requires additional photocatalysts and oxidants. Therefore, it is highly desirable to develop alternatively efficient, sustainable, green, and environmentally friendly synthetic methods avoiding transition metal catalysts and chemical oxidants.
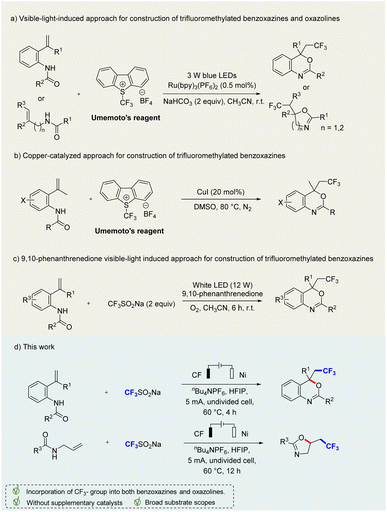 |
| Scheme 1 Strategies for the synthesis of trifluoromethylated benzoxazines. | |
Organic electrochemistry provides an effective and sustainable strategy for the synthesis of valuable chemicals, employing inexpensive and renewable electrons as redox reagents.11 In our continuous efforts, our goal is to develop green, metal-free, and efficient methods to construct diversified heterocyclic scaffolds.12 In our previous work, we reported a direct azidation of benzylic C(sp3)–H bonds through an electrochemical process.13 Herein, we'd like to report a new finding to construct trifluorinated benzoxazines and oxazolines through an effective electrochemical strategy, which may use cheap carbon fibre and nickel plates as electrodes in an undivided cell, without any external chemical oxidants, metal catalysts and additives (Scheme 1d). However, while we were preparing this manuscript, a similar work appeared, focusing on the construction of CF2-substituted benzoxazines,14 in which the reaction system required trifluoroacetic acid as a catalyst, adding complexity to the reaction system. In contrast, our reaction system is simpler and environmentally benign without the need for a transition metal catalyst or external oxidant, and can proceed smoothly with diverse functional group compatibility.
Results and discussion
Based on the above conception, we have attempted to achieve the CF3-containing benzoxazines by treatment of N-(2-(prop-1-en-2-yl)phenyl)benzamide (1a) with CF3SO2Na. The reaction was carried out in an undivided cell equipped with a carbon fibre (CF) anode and a nickel plate (Ni) cathode under a constant current of 5 mA (Table 1). The desired product 2a was obtained in 72% yield when nBu4NPF6 was used as the electrolyte in HFIP at 60 °C for 4 h (entry 1). We tried other electrolytes, such as Et4NBF4, nBu4NOAc, nBu4NBr, and nBu4NI. Et4NBF4 resulted in a significant decrease (entry 2) in yield, only trace of the product was observed when using nBu4NOAc and nBu4NBr as electrolytes (entry 3), and the product was I-containing benzoxazine derivative when using nBu4NI as the electrolyte (entry 4). Besides, the product 2a also was observed in the absence of electrolyte (entry 5). When we replaced solvent with DMSO (entry 6), CH3CN (entry 7), CH3OH (entry 8), and DCE (entry 9), all of them resulted in a slight decrease in the yield. This could be attributed to the ability of HFIP to stabilize radical cation intermediates, thereby aiding in substrate oxidation while preventing the product of overoxidation.15 We further evaluated other electrode materials, including Pt plate (entry 10), Fe plate (entry 11) as anode, and Fe plate (entry 12), Al plate (entry 13) as cathode, none of them was more effective. We transformed the current to 2 mA (entry 14) or 10 mA current (entry 15), the reaction efficiency was noticeably dropped in 2 mA current. The product 2a was decreased under 10 mA current, which speculated that high current may cause peroxidation. The reaction temperature also was investigated, which led to lower yields (entries 16–18). When the equivalent of CF3SO2Na was reduced to 1, it resulted in a slight decrease in the yield (entry 19). Furthermore, electricity (entry 20) was essential for the process of the reaction.
Table 1 Optimization of reaction conditionsa
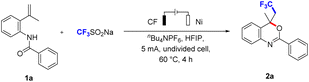
|
Entry |
Variation from standard conditions |
Yieldb [%] |
Reaction conditions: undivided cell, 1a (0.25 mmol), CF3SO2Na (0.5 mmol), solvent (6 mL), nBu4NPF6 (0.5 mmol), 5 mA, 60 °C, 4 h (3.0 F mol−1). Isolated yield. Under air atmosphere. CF = Carbon fibre (1 × 1 × 0.01 cm), Pt = platinum (1 × 1 × 0.01 cm), Ni = nickel (1 × 1 × 0.01 cm). HFIP, 1,1,1,3,3,3-hexafluoro-2-propanol, DCE, 1,2-dichloroethane. |
1 |
None |
72 |
2 |
Et4NBF4 as electrolyte |
39 |
3 |
nBu4NBr or nBu4NOAc as electrolyte |
Trace |
4 |
nBu4NI as electrolyte |
0 |
5 |
No electrolyte |
13 |
6 |
DMSO as solvent |
51 |
7 |
CH3CN as solvent |
38 |
8 |
CH3OH as solvent |
37 |
9 |
DCE as solvent |
66 |
10 |
Pt plate as anode |
25 |
11 |
Fe plate as anode |
17 |
12 |
Fe plate as cathode |
29 |
13 |
Al plate as cathode |
56 |
14 |
2 mA |
42 |
15 |
10 mA |
60 |
16 |
r.t. |
35 |
17 |
40 °C |
64 |
18 |
80 °C |
59 |
19 |
CF3SO2Na (1 equiv.) |
63 |
20 |
No electricity |
0 |
With the optimal conditions in hand, the substrate scope of CF3-containing benzoxazines was explored (Scheme 2). Firstly, we introduced electron-donating groups or electron-withdrawing groups into N-(2-(prop-1-en-2-yl)phenyl)benzamide (1a) and they reacted smoothly to obtain corresponding products 2 in moderate to good yields, such as methyl (2b), methoxy (2c), and halides (2d, 2e, 2f, 2i, and 2j), especially the strong electron-withdrawing groups trifluoromethyl (2g) and nitryl (2h) were all tolerant. Besides, we replaced the R2 group by methyl (2k), tertiary butyl (2l), cyclopropyl (2m), cyclohexyl (2n), which reacted smoothly to afford the target product in good yields. Furyl (2o) or thienyl (2p) was transformed into the desired product in moderate yields, but pyridyl (2q) could not produce the target product. We speculated that the electron-withdrawing effect of pyridine made it difficult for 1q to generate the corresponding intermediate I or II. We also introduced morpholinyl (2r) and naphthyl (2s) into R2 group, the target products were obtained. Further explorations about the R1 group were hydrogen (2t) and phenyl (2u), the corresponding target compounds were also generated and showed great compatibility. In this synthetic system, CF2-substituted benzoxazines were also successfully synthesized using CF2HSO2Na as the difluoromethylation reagent (31% yield for compound 2v), which indicates that the reaction system has good applicability.
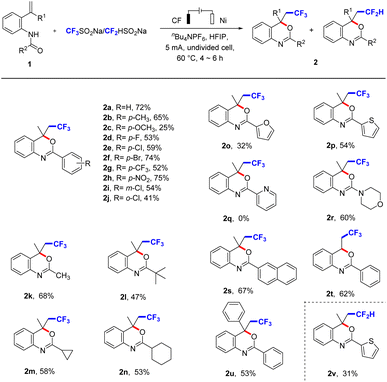 |
| Scheme 2 Substrate scope of CF3-containing benzoxazines. aReaction conditions: undivided cell, 1 (0.25 mmol), CF3SO2Na/CF2HSO2Na (0.5 mmol), nBu4NPF6 (0.5 mmol), HFIP (6 mL), under air atmosphere. | |
We further explored the substrate scope of CF3-containing oxazolines, and the results were shown in Scheme 3. N-allylbenzamide 3a was reacted with CF3SO2Na to access the trifluoromethylation product 2-phenyl-5-(2,2,2-trifluoroethyl)-4,5-dihydrooxazole (4a) in 59% yield. N-allylbenzamides with various substituents such as methyl (4b), methoxy (4c), halides (4d, 4e and 4f) were all tolerant. The benzene rings with electron-deficient nitryl (4g) gave 82% yield. Meanwhile, when introducing furyl (4h) into the R3 group, the corresponding target product also was obtained. But introducing cyclohexyl (4i) into R3 group could not produce the target product, which indicated it had a very great influence on this transformation.
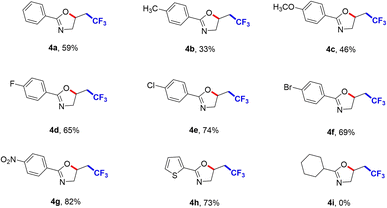 |
| Scheme 3 Substrate scope of CF3-containing oxazolines. aReaction conditions: undivided cell, 3 (0.25 mmol), CF3SO2Na (0.5 mmol), nBu4NPF6 (0.5 mmol), HFIP (6 mL), under air atmosphere. | |
To further evaluate the practicality and potential applications of this method, we performed the reaction on a gram-scale preparation with 1a, and the yield of product 2a was 60% under a constant current of 5 mA for 64 h (Scheme 4a). In addition, the product 2a can be further converted into 2-(2-(benzylamino)phenyl)-4,4,4-trifluorobutan-2-ol (5) at a yield of 78% (Scheme 4b).
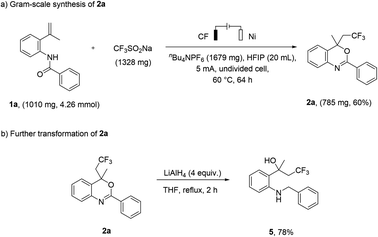 |
| Scheme 4 Gram-scale synthesis and further transformation. | |
In order to investigate the possible mechanism of this transition, several control experiments were performed. No desired product was obtained when 2,2,6,6-Tetramethylpiperidoxyl (TEMPO) was added (Scheme 5).
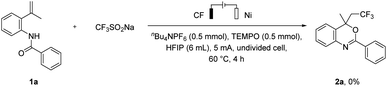 |
| Scheme 5 Control experiments of the reaction. | |
A plausible mechanism for the formation of product has been proposed based on the related reports.16 As explained in Scheme 6, initially the HFIP undergoes cathodic reduction to generate hydrogen gas at the cathode. At the anode, CF3SO2Na produces the CF3SO2 radical under anodic oxidation and further forms the CF3 radical. Subsequently, CF3 radicals are added to the double bonds of the olefins to generate the alkyl radical intermediate I. I undergoes a radical cyclization and anodic oxidation to furnish intermediate II. Afterwards, the intermediate II is finally converted into CF3-containing benzoxazine 2a by deprotonation (Scheme 6).
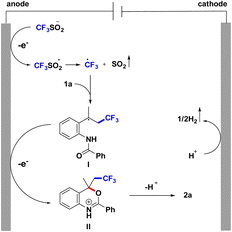 |
| Scheme 6 Proposal mechanism of the reaction. | |
To justify the proposed reaction pathway outlined in Scheme 6, we conducted cyclic voltammetric (CV) experiments. As shown in Fig. 2, the oxidation peak of CF3SO2Na was 0.83 V, and 1a had an oxidation peak of 1.32 V. These results indicated that CF3SO2Na was oxidized preferentially at the anode (see the ESI† for details).
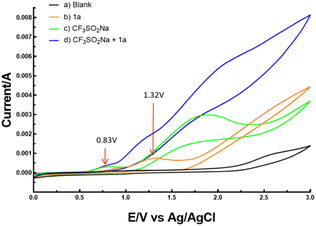 |
| Fig. 2 Cyclic voltammetric experiments of 1a and CF3SO2Na. | |
Conclusions
In summary, we have developed a mild and efficient electrochemical oxidative and radical cascade cyclization of olefinic amides to afford trifluorinated benzoxazines and oxazolines using cheap and durable nickel plates as electrodes. This paper presents a simple, practical, green and environmentally benign protocol for the synthesis of fluorinated benzoxazines and oxazolines. In the absence of any transition metal catalysts, external oxidizers and additives, this protocol proceeds smoothly with diverse functional group compatibility.
Conflicts of interest
There are no conflicts to declare.
Acknowledgements
We are grateful to the National Natural Science Foundation of China (No. 82130105, 82121005, and 91953108), the research funds of Hangzhou institute for advanced study (No. 2022ZZ01015, 2022ZZ01012, 2022ZZ01019).
Notes and references
- G. Eichenbaum, J. Zhou, M. F. Kelley, W. Roosen, P. Costa-Giomi, C. Louden, N. A. Di Prospero, G. Pandina, J. B. Singh, L. Ford, J. A. Moyer, T. M. Nork, J. N. Ver Hoeve and G. D. Aguirre, Implications of retinal effects observed in chronic toxicity studies on the clinical development of a CNS-active drug candidate, Regul. Toxicol. Pharmacol., 2014, 69, 187–200 CrossRef CAS PubMed.
-
(a) J. Ilaš, Ž. Jakopin, T. Borštnar, M. Stegnar and D. Kikelj, 3,4-Dihydro-2H-1,4-benzoxazine Derivatives Combining Thrombin Inhibitory and Glycoprotein IIb/IIIa Receptor Antagonistic Activity as a Novel Class of Antithrombotic Compounds with Dual Function, J. Med. Chem., 2008, 51, 5617–5629 CrossRef PubMed;
(b) T. Kline, N. H. Andersen, E. A. Harwood, J. Bowman, A. Malanda, S. Endsley, A. L. Erwin, M. Doyle, S. Fong, A. L. Harris, B. Mendelsohn, K. Mdluli, C. R. H. Raetz, C. K. Stover, P. R. Witte, A. Yabannavar and S. Zhu, Potent, Novel in vitro Inhibitors of the Pseudomonas aeruginosa Deacetylase LpxC, J. Med. Chem., 2002, 45, 3112–3129 CrossRef CAS PubMed;
(c) H. B. Bode, H. Irschik, S. C. Wenzel, H. Reichenbach, R. Müller and G. Höfle, The Leupyrrins: A Structurally Unique Family of Secondary Metabolites from the Myxobacterium Sorangium cellulosum, J. Nat. Prod., 2003, 66, 1203–1206 CrossRef CAS PubMed;
(d) N. Dias, J.-F. Goossens, B. Baldeyrou, A. Lansiaux, P. Colson, A. Di Salvo, J. Bernal, A. Turnbull, D. J. Mincher and C. Bailly, Oxoazabenzo[de]anthracenes Conjugated to Amino Acids: Synthesis and Evaluation as DNA-Binding Antitumor Agents, Bioconjugate Chem., 2005, 16, 949–958 CrossRef CAS PubMed;
(e) S. J. Hays, B. W. Caprathe, J. L. Gilmore, N. Amin, M. R. Emmerling, W. Michael, R. Nadimpalli, R. Nath, K. J. Raser, D. Stafford, D. Watson, K. Wang and J. C. Jaen, 2-Amino-4H-3,1-benzoxazin-4-ones as Inhibitors of C1r Serine Protease, J. Med. Chem., 1998, 41, 1060–1067 CrossRef CAS PubMed;
(f) A. Krantz, R. W. Spencer, T. F. Tam, T. J. Liak, L. J. Copp, E. M. Thomas and S. P. Rafferty, Design and synthesis of 4H-3,1-benzoxazin-4-ones as potent alternate substrate inhibitors of human leukocyte elastase, J. Med. Chem., 1990, 33, 464–479 CrossRef CAS PubMed.
- R. Schlichter, V. Rybalchenko, P. Poisbeau, M. Verleye and J.-M. Gillardin, Modulation of GABAergic synaptic transmission by the non-benzodiazepine anxiolytic etifoxine, Neuropharmacology, 2000, 39, 1523–1535 CrossRef CAS PubMed.
- M. M. Bastos, C. C. P. Costa, T. C. Bezerra, F. d. C. da Silva and N. Boechat, Efavirenz a nonnucleoside reverse transcriptase inhibitor of first-generation: Approaches based on its medicinal chemistry, Eur. J. Med. Chem., 2016, 108, 455–465 CrossRef CAS PubMed.
-
(a) P. Zhang, E. A. Terefenko, A. Fensome, J. Wrobel, R. Winneker, S. Lundeen, K. B. Marschke and Z. Zhang, 6-Aryl-1,4-dihydro-benzo[d][1,3]oxazin- 2-ones: A Novel Class of Potent, Selective, and Orally Active Nonsteroidal Progesterone Receptor Antagonists, J. Med. Chem., 2002, 45, 4379–4382 CrossRef CAS PubMed;
(b) A. Fensome, R. Bender, R. Chopra, J. Cohen, M. A. Collins, V. Hudak, K. Malakian, S. Lockhead, A. Olland, K. Svenson, E. A. Terefenko, R. J. Unwalla, J. M. Wilhelm, S. Wolfrom, Y. Zhu, Z. Zhang, P. Zhang, R. C. Winneker and J. Wrobel, Synthesis and Structure–Activity Relationship of Novel 6-Aryl-1,4- dihydrobenzo[d][1,3]oxazine-2-thiones as Progesterone Receptor Modulators Leading to the Potent and Selective Nonsteroidal Progesterone Receptor Agonist Tanaproget, J. Med. Chem., 2005, 48, 5092–5095 CrossRef CAS PubMed.
- Z. Jin, Muscarine, imidazole, oxazole and thiazole alkaloids, Nat. Prod. Rep., 2009, 26, 382–445 RSC.
- E. R. Freiter, A. H. Abdallah and S. J. Strycker, 2-Amino-5-substituted oxazolines and intermediates as potential anorectants, J. Med. Chem., 1973, 16, 510–512 CrossRef CAS PubMed.
-
(a) K. Müller, C. Faeh and F. Diederich, Fluorine in Pharmaceuticals: Looking Beyond Intuition, Science, 2007, 317, 1881–1886 CrossRef PubMed;
(b) J. Wang, M. Sánchez-Roselló, J. L. Aceña, C. del Pozo, A. E. Sorochinsky, S. Fustero, V. A. Soloshonok and H. Liu, Fluorine in Pharmaceutical Industry: Fluorine-Containing Drugs Introduced to the Market in the Last Decade (2001–2011), Chem. Rev., 2014, 114, 2432–2506 CrossRef CAS PubMed;
(c) Y. Zhou, J. Wang, Z. Gu, S. Wang, W. Zhu, J. L. Aceña, V. A. Soloshonok, K. Izawa and H. Liu, Next Generation of Fluorine-Containing Pharmaceuticals, Compounds Currently in Phase II–III Clinical Trials of Major Pharmaceutical Companies: New Structural Trends and Therapeutic Areas, Chem. Rev., 2016, 116, 422–518 CrossRef CAS PubMed;
(d) S. Purser, P. R. Moore, S. Swallow and V. Gouverneur, Fluorine in medicinal chemistry, Chem. Soc. Rev., 2008, 37, 320–330 RSC.
-
(a) P. Natarajan, D. Chuskit, Priya and Manjeet, Transition-metal-free synthesis of trifluoromethylated benzoxazines via a visible-light-promoted tandem difunctionalization of o-vinylanilides with trifluoromethylsulfinate, New J. Chem., 2022, 46, 322–327 RSC;
(b) F. Lu, J. Xu, H. Li, K. Wang, D. Ouyang, L. Sun, M. Huang, J. Jiang, J. Hu, H. Alhumade, L. Lu and A. Lei, Electrochemical oxidative radical cascade cyclization of olefinic amides and thiophenols towards the synthesis of sulfurated benzoxazines, oxazolines and iminoisobenzofurans, Green Chem., 2021, 23, 7982–7986 RSC;
(c) T.-J. He, W.-Q. Zhong and J.-M. Huang, The synthesis of sulfonated 4H-3,1-benzoxazines via an electro-chemical radical cascade cyclization, Chem. Commun., 2020, 56, 2735–2738 RSC;
(d) Qumruddeen, A. Yadav, R. Kant and C. B. Tripathi, Lewis Base/Brønsted Acid Cocatalysis for Thiocyanation of Amides and Thioamides, J. Org. Chem., 2020, 85, 2814–2822 CrossRef CAS PubMed;
(e) T.-T. Cao, W.-K. Zhang, F.-H. Qin, Q.-Q. Kang, Y. Dong, Q. Li, C. Kang and W.-T. Wei, Halocyclization of Olefinic 1,3-Dicarbonyls and Olefinic Amides in Aqueous Media Open in Air at Room Temperature, ACS Sustain. Chem. Eng., 2020, 8, 16946–16951 CrossRef CAS;
(f) M. Chaitanya and P. Anbarasan, Acid-Mediated Oxychalcogenation of o-Vinylanilides with N-(Arylthio/arylseleno)succinimides, Org. Lett., 2018, 20, 1183–1186 CrossRef CAS PubMed;
(g) A. Theodorou, I. Triandafillidi and C. G. Kokotos, Organocatalytic Synthesis of Oxazolines and Dihydrooxazines from Allyl-Amides: Bypassing the Inherent Regioselectivity of the Cyclization, Adv. Synth. Catal., 2018, 360, 951–957 CrossRef CAS;
(h) T. Liu, D. Zheng, Z. Li and J. Wu, Synthesis of Sulfonated Benzo[d][1,3]oxazines by Merging Photoredox Catalysis and Insertion of Sulfur Dioxide, Adv. Synth. Catal., 2018, 360, 865–869 CrossRef CAS;
(i) J. Wang, R. Sang, X. Chong, Y. Zhao, W. Fan, Z. Li and J. Zhao, Copper-catalyzed radical cascade oxyalkylation of olefinic amides with simple alkanes: highly efficient access to benzoxazines, Chem. Commun., 2017, 53, 7961–7964 RSC;
(j) W. Fu, X. Han, M. Zhu, C. Xu, Z. Wang, B. Ji, X.-Q. Hao and M.-P. Song, Visible-light-mediated radical oxydifluoromethylation of olefinic amides for the synthesis of CF2H-containing heterocycles, Chem. Commun., 2016, 52, 13413–13416 RSC;
(k) J.-F. Zhao, X.-H. Duan, H. Yang and L.-N. Guo, Transition-Metal-Free Oxyfluorination of Olefinic Amides for the Synthesis of Fluorinated Heterocycles, J. Org. Chem., 2015, 80, 11149–11155 CrossRef CAS PubMed;
(l) H. Yang, X.-H. Duan, J.-F. Zhao and L.-N. Guo, Transition-Metal-Free Tandem Radical Thiocyanooxygenation of Olefinic Amides: A New Route to SCN-Containing Heterocycles, Org. Lett., 2015, 17, 1998–2001 CrossRef CAS PubMed;
(m) Q.-H. Deng, J.-R. Chen, Q. Wei, Q.-Q. Zhao, L.-Q. Lu and W.-J. Xiao, Visible-light-induced photocatalytic oxytrifluoromethylation of N-allylamides for the synthesis of CF3-containing oxazolines and benzoxazines, Chem. Commun., 2015, 51, 3537–3540 RSC;
(n) Y.-M. Wang, J. Wu, C. Hoong, V. Rauniyar and F. D. Toste, Enantioselective Halocyclization Using Reagents Tailored for Chiral Anion Phase-Transfer Catalysis, J. Am. Chem. Soc., 2012, 134, 12928–12931 CrossRef CAS PubMed;
(o) J. G. Yang, X. F. Song, J. Wang, K. Li, X. Y. Chang, L. Y. Tan, C. X. Liu, F. H. Yu, G. L. Cui, G. Cheng, W. P. To, C. L. Yang, C. M. Che and Y. Chen, Highly Efficient Thermally Activated Delayed Fluorescence from Pyrazine-Fused Carbene Au(I) Emitters, Chem.–Eur. J., 2021, 27, 17834–17842 CrossRef CAS PubMed;
(p) N. Yang, A. N. Li, H. Gao, L. M. Liao, Y. P. Yang, P. L. Wang and H. J. Li, Electrochemical oxidation-induced benzylic C(sp3)–H functionalization towards the atom-economic synthesis of oxazole heterocycles, Green Chem., 2023, 25, 5128–5133 RSC.
- S. Jana, A. Ashokan, S. Kumar, A. Verma and S. Kumar, Copper-catalyzed trifluoromethylation of alkenes: synthesis of trifluoromethylated benzoxazines, Org. Biomol. Chem., 2015, 13, 8411–8415 RSC.
-
(a) C. Zhu, N. W. J. Ang, T. H. Meyer, Y. Qiu and L. Ackermann, Organic Electrochemistry: Molecular Syntheses with Potential, ACS Cent. Sci., 2021, 7, 415–431 CrossRef CAS PubMed;
(b) G. M. Martins, G. C. Zimmer, S. R. Mendes and N. Ahmed, Electrifying green synthesis: recent advances in electrochemical annulation reactions, Green Chem., 2020, 22, 4849–4870 RSC;
(c) N. E. S. Tay, D. Lehnherr and T. Rovis, Photons or Electrons? A Critical Comparison of Electrochemistry and Photoredox Catalysis for Organic Synthesis, Chem. Rev., 2022, 122, 2487–2649 CrossRef CAS PubMed;
(d) K.-J. Jiao, Y.-K. Xing, Q.-L. Yang, H. Qiu and T.-S. Mei, Site-Selective C–H Functionalization via Synergistic Use of Electrochemistry and Transition Metal Catalysis, Acc. Chem. Res., 2020, 53, 300–310 CrossRef CAS PubMed;
(e) P. Xiong and H.-C. Xu, Chemistry with Electrochemically Generated N-Centered Radicals, Acc. Chem. Res., 2019, 52, 3339–3350 CrossRef CAS PubMed;
(f) Y. Yuan and A. Lei, Electrochemical Oxidative Cross-Coupling with Hydrogen Evolution Reactions, Acc. Chem. Res., 2019, 52, 3309–3324 CrossRef CAS PubMed;
(g) S. D. Minteer and P. Baran, Electrifying Synthesis: Recent Advances in the Methods, Materials, and Techniques for Organic Electrosynthesis, Acc. Chem. Res., 2020, 53, 545–546 CrossRef CAS PubMed;
(h) Y. Jiang, K. Xu and C. Zeng, Use of Electrochemistry in the Synthesis of Heterocyclic Structures, Chem. Rev., 2018, 118, 4485–4540 CrossRef CAS PubMed;
(i) A. Wiebe, T. Gieshoff, S. Möhle, E. Rodrigo, M. Zirbes and S. R. Waldvogel, Electrifying Organic Synthesis, Angew.
Chem., Int. Ed., 2018, 57, 5594–5619 CrossRef CAS PubMed;
(j) M. Yan, Y. Kawamata and P. S. Baran, Synthetic Organic Electrochemical Methods Since 2000: On the Verge of a Renaissance, Chem. Rev., 2017, 117, 13230–13319 CrossRef CAS PubMed;
(k) B. A. Frontana-Uribe, R. D. Little, J. G. Ibanez, A. Palma and R. Vasquez-Medrano, Organic electrosynthesis: a promising green methodology in organic chemistry, Green Chem., 2010, 12, 2099–2119 RSC.
- G. He, J. Ma, J. Zhou, C. Li, H. Liu and Y. Zhou, A metal-free method for the facile synthesis of indanones via the intramolecular hydroacylation of 2-vinylbenzaldehyde, Green Chem., 2021, 23, 1036–1040 RSC.
- G. He, Y. Li, S. Zhou, X. Yang, A. Shang, Y. Wang, H. Liu and Y. Zhou, A Facile Electrochemical Strategy for the Azidation of Benzylic C(sp3)–H Bonds, Eur. J. Org Chem., 2022, 2022, e202201041 CrossRef CAS.
- X. Chen, J. Jiang, X.-J. Huang and W.-M. He, Electrochemical oxidative radical cascade reactions for the synthesis of difluoromethylated benzoxazines, Org. Chem. Front., 2023, 10, 3898–3902 RSC.
-
(a) L. Eberson, M. P. Hartshorn, F. Radner and O. Persson, Persistent radical cation solutions from the reaction between aromatics and bromine, chlorine or iodine chloride in 1,1,1,3,3,3-hexafluoropropan-2-ol at room temperature, Chem. Commun., 1996, 215–216 RSC;
(b) B. Elsler, A. Wiebe, D. Schollmeyer, K. M. Dyballa, R. Franke and S. R. Waldvogel, Source of Selectivity in Oxidative Cross-Coupling of Aryls by Solvent Effect of 1,1,1,3,3,3-Hexafluoropropan-2-ol, Chem.–Eur. J., 2015, 21, 12321–12325 CrossRef CAS PubMed;
(c) L. Schulz, M. Enders, B. Elsler, D. Schollmeyer, K. M. Dyballa, R. Franke and S. R. Waldvogel, Reagent- and Metal-Free Anodic C-C Cross-Coupling of Aniline Derivatives, Angew. Chem., Int. Ed., 2017, 56, 4877–4881 CrossRef CAS PubMed;
(d) L. Schulz and S. R. Waldvogel, Solvent Control in Electro-Organic Synthesis, Synlett, 2019, 30, 275–286 CrossRef CAS.
-
(a) S. Zhang, L. Li, J. Zhang, J. Zhang, M. Xue and K. Xu, Electrochemical fluoromethylation triggered lactonizations of alkenes under semi-aqueous conditions, Chem. Sci., 2019, 10, 3181–3185 RSC;
(b) V. A. Vil, V. M. Merkulova, A. I. Ilovaisky, S. A. Paveliev, G. I. Nikishin and A. O. Terent'ev, Electrochemical Synthesis of Fluorinated Ketones from Enol Acetates and Sodium Perfluoroalkyl Sulfinates, Org. Lett., 2021, 23, 5107–5112 CrossRef CAS PubMed;
(c) F. Lu, J. Xu, H. Li, K. Wang, D. Ouyang, L. Sun, M. Huang, J. Jiang, J. Hu, H. Alhumade, L. Lu and A. Lei, Electrochemical oxidative radical cascade cyclization of olefinic amides and thiophenols towards the synthesis of sulfurated benzoxazines, oxazolines and iminoisobenzofurans, Green Chem., 2021, 23, 7982–7986 RSC;
(d) A. Claraz, T. Courant and G. Masson, Electrochemical Intramolecular Oxytrifluoromethylation of N-Tethered Alkenyl Alcohols: Synthesis of Functionalized Morpholines, Org. Lett., 2020, 22, 1580–1584 CrossRef CAS PubMed.
|
This journal is © The Royal Society of Chemistry 2024 |