DOI:
10.1039/D3FO03801G
(Review Article)
Food Funct., 2024,
15, 62-78
Non-starch polysaccharides from kidney beans: comprehensive insight into their extraction, structure and physicochemical and nutritional properties
Received
8th September 2023
, Accepted 15th November 2023
First published on 29th November 2023
Abstract
Kidney beans (Phaseolus vulgaris L.) are an important legume source of carbohydrates, proteins, and bioactive molecules and thus have attracted increasing attention for their high nutritional value and sustainability. Non-starch polysaccharides (NSPs) in kidney beans account for a high proportion and have a significant impact on their biological functions. Herein, we critically update the information on kidney bean varieties and factors that influence the physicochemical properties of carbohydrates, proteins, and phenolic compounds. Furthermore, their extraction methods, structural characteristics, and health regulatory effects, such as the regulation of intestinal health and anti-obesity and anti-diabetic effects, are also summarized. This review will provide suggestions for further investigation of the structure of kidney bean NSPs, their relationships with biological functions, and the development of NSPs as novel plant carbohydrate resources.
1. Introduction
In recent years, plant-based foods have gained worldwide attention due to their high nutritive value, sustainability, and ethical considerations.1 Kidney beans (Phaseolus vulgaris L.), also known as common beans, have been highlighted as an important source of legume proteins, dietary fiber, and bioactive compounds.2 Statistics show that the global production of kidney beans reached up to 27.72 million tons in 2021,3 which stands second to that of soybeans.4 Kidney beans belong to the subfamily of Pteridophyta, originating from Mexico and Argentina, and have been widely introduced throughout the world.5 The main cultivated types of kidney beans include white, red, haricot, pinto, navy, black, and light-spotted beans.6 They differ in the seed color, size, and shape in terms of morphological characteristics, and usually exhibit differences in nutrient distribution, physicochemical properties, and health regulatory effects.7–9
Increasing evidence has shown the regulatory effects of a kidney bean extract on catabolic enzymes, with a potential to improve the metabolic health and overweight syndrome.10,11 The extracts are mainly rich in kidney bean proteins, such as phaseolin and α-amylase inhibitors.12,13 Besides being an important protein source, kidney beans are an abundant source of carbohydrates.14,15 Particularly, non-starch polysaccharides (NSPs) have been increasingly investigated in recent years. NSPs are an important component in the cell wall of kidney beans, which mainly contain the insoluble dietary fiber cellulose and soluble dietary fiber pectin and hemicellulose.16 Many studies have successfully proven that kidney bean NSPs can improve intestinal health by shaping the gut microbiota,17,18 thereby alleviating the symptoms of colon cancer, obesity, and diabetes.19–21
However, these beneficial functions are highly dependent on the purity and structural characteristics of NSPs for valid reasons.22,23 NSPs extracted from kidney beans usually contain the residues of proteins or polyphenols in the form of glycoproteins16,24 or cross-linked as pectin–cation–phytate.25 In this case, the release of nutrients is restricted,26 and the stability of NSP emulsions is affected with the development of hard-to-cook (HTC) defect.27,28 Therefore, it is important to understand the interactions between NSPs and other bioactive molecules in kidney beans. In addition, the extraction yield and structural properties of kidney bean NSPs differ among cultivars, which may guide the development of plant-based novel functional components. However, review articles on kidney beans in recent years mainly focused on the following aspects: (1) nutritional and functional properties of kidney beans together with other legumes from the regions of India,29 Mexico,30 Europe,31 and Africa;32 (2) food processing technologies;33–36 (3) potential strategies to improve metabolic health with a white kidney bean extract,10 prevent cardiovascular diseases with kidney bean bioactive compounds,37 inhibit noncommunicable chronic diseases,38 and alleviate diabetes and obesity with kidney bean α-amylase inhibitors.39 To the best of our knowledge, there is no report, to date, comprehensively reviewing kidney bean NSPs, including their extraction, structural characterization, biological function, and interaction with other nutrients.
In this review, we first briefly introduce the kidney bean varieties and the relationships between their morphological characters and cooking as well as their nutritional properties. Next, we discuss the factors that influence the content and composition of kidney bean carbohydrates, proteins, and phenolic compounds. More importantly, the interactions between kidney bean NSPs and proteins, phenolic compounds, and minerals of Ca2+ are reviewed. Finally, we critically review the current insight into the extraction techniques, structural properties, and health regulatory functions of kidney bean NSPs. This knowledge may contribute to the development of novel legume-derived NSPs in the field of carbohydrate nutrition.
2. Varieties of kidney beans
A large amount of kidney bean varieties is available in the market, differing in seed size, shape, and color (Table 1 and Fig. 1).40,41 Most kidney beans are characterized as elongated in shape, besides round or elliptical in shape. It is worth noting that the size of kidney beans is correlated to some extent with the distribution of starches, i.e., a greater average number of starch granules embedded in a thinner protein matrix area was detected in smaller-sized seeds, whereas large-sized seeds showed a thicker protein matrix decorated with less starch granules.42 These differences in starch distribution among different kidney bean varieties may contribute to their diverse starch digestibility, thermal, and pasting properties.40,42 Besides genotype effects, kidney bean powder size was correlated to some extent with the starch component, i.e., the smaller the size of the kidney bean powder, the higher the content of rapidly digestible starch (RDS), and the lower the content of slowly digestible starch (SDS) and resistant starch (RS), which is attributed to their larger surface area relative to weight.40
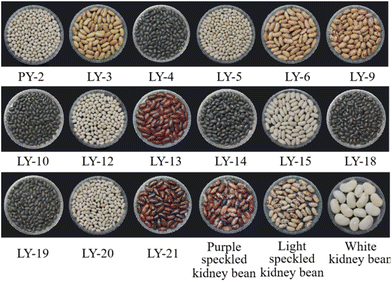 |
| Fig. 1 Kidney beans vary in seed color, shape, and size. PY, Pinyun and LY, Longyun. | |
Table 1 A comparison of appearance characteristics, nutritional composition, processing adaptability, and physiological functions among different kidney bean cultivars
Cultivars |
Appearance characteristics |
Nutritional characteristics |
Processing adaptability or biological activity |
Place of origin |
Ref. |
Color |
Shape |
Size |
SDS: slowly digestible starch; RS: resistant starch; RDS: rapidly digestible starch; FV: final viscosity; V1: viscosity at the beginning of the holding period; SB: setback; ETC: easy to cook; HTC: hard to cook. |
25 varieties of edible dry beans |
White, light brown-pink, brown-red, light red, dark red, black |
Elongated to round shape: dark red, light red, and white kidney beans > pinto > navy and black market beans |
Dark red, light red and white kidney beans > navy > blue and black market beans |
The size is positively correlated with SDS and RS, and negatively correlated with RDS |
Higher SB values and protein for gluten-free pasta; lower SB values for soft baking, such as bread; higher V1 and FV for thickening agents |
Michigan, USA |
40
|
26 varieties of kidney beans |
White, brownish red, red, black |
Slender shape |
— |
Darker beans contain more total phenolic content, tannins, and anthocyanins, with more Ca and Zn, but less Cu, Mn, and Fe |
— |
Shandong and Jiangxi Provinces, China |
43
|
Red kidney bean, black-eyed pea, red bean and black beans |
Red, black |
— |
— |
Red kidney bean extract has the highest content of phenols and flavonoids |
Red kidney bean seed coat is a good source of anti-diabetic drugs |
Setiawan, Malaysia |
46
|
9 varieties (EC-590329, EC-572723, EC-590326, Pi-244719, Pi-312296, Pi-249554, PLB-10-1, PLB-14-1 and IC-382213) |
The color varies from light yellow-white, yellow-gray to dark red |
The shape varies from spherical to elliptical |
— |
Dry matter digestibility (14.60–47.20%), RS content (32.00–50.50%) |
Water absorption capacity (1.70–2.70 g g−1), fat absorption capacity (1.40–1.70 g g−1), emulsification activity index (50.10–70.10 m2 g−1), foaming capacity (70.80–98.30%) and foam stability (82.40–91.30%) |
Shimla, India |
47
|
Pinto beans, yellow kidney beans and red haricot beans |
Red, yellow |
— |
— |
Yellow kidney beans have a high protein content |
Yellow kidney beans can be used to produce high-protein milk |
Nairobi, Kenya |
49
|
Barlotto bean, white kidney bean (P. vulgaris L.) |
White |
— |
16.43 mm of length, 8.50 mm of width |
— |
Both atmospheric and high-pressure cooking can increase RDS. High-pressure cooking induce a higher RS level while a lower SDS level than atmospheric pressure |
Mersin, Turkey |
48
|
Owing to the genotype variance of kidney bean varieties, different color phenotypes have been developed, which are associated with their nutritional property, in particular pigmented nutrients. For example, non-white beans contain more anthocyanins, proanthocyanidins, and phenolics, while these compounds are hardly detected in white beans. These compounds may contribute to the higher anti-oxidant activity of non-white kidney beans compared to white kidney beans.43,44 Interestingly, non-white kidney beans are usually found to have HTC defect. Evidence for this was provided by the conversion of low-molecular-weight phenolics to higher molecular weight tannins, which have a darker color.44 Alternatively, the crosslink between phenolics and pectins may also contribute to the HTC defect, given that the pectins of HTC kidney beans are less soluble, less acetylated, and contain more branched pectins (i.e., arabinans).45
These differences in seed characteristics and nutritional property among the varieties of kidney beans make them candidates for the preparation of different foods to meet personalized nutrient demands. For example, in a comparative study of four types of kidney beans, namely, black-eyed pea, red bean, black bean, and red kidney bean, the red kidney bean extract showed the highest phenolic and flavonoid content. In addition, red kidney bean exhibited stronger antioxidant activity and stronger inhibition of α-glucosidase and α-amylase, which suggest that it is a natural alternative to anti-diabetic drugs for the treatment of diabetes.46 Also, the redness of kidney bean flour is positively corrected with the content of NSPs. Although the content of NSPs was negatively correlated with the foaming and emulsifying capacities, owing to the inhibition of protein adsorption on the interfacial film, they showed stronger oil binding capacity, reduced breakdown viscosity, and contributed to the stability of bean flour during pasting.47 However, the physicochemical and nutritional properties of raw materials can be altered by food processing. For example, atmospheric-pressure cooking and high-pressure cooking can both reduce the lightness and increase the redness and yellowness of light-colored beans, while decrease the redness and yellowness of dark varieties.48 Although kidney beans are rich in proteins, this concentration changes during the preparation of plant milk. In a study including yellow kidney, red haricot, and pinto beans, the protein level of the yellow kidney beans was significantly higher and showed the highest protein level as in milk, which makes it the best choice for the high plant protein milk industry.49 In general, heating treatment during preparation of plant milk causes the denaturation of proteins, resulting in a lower protein content in the milk. Thus, the extraction of kidney bean protein in milk among the different varieties needs to be further investigated.
3. Nutritional components of kidney beans
3.1 Carbohydrates
Carbohydrates constitute the main nutrient in kidney beans, with an abundance in the range of 58.64% to 70.90% of the dry weight (dw) (Table 2).47,49–52 These carbohydrates are dominated by dietary fibers, among which water insoluble dietary fibers (IDF) are mainly found in the seed coat of kidney beans, whereas water soluble dietary fibers (SDF), resistant starch (RS), oligosaccharides, and slowly digested starch (SDS) are mainly found in the cotyledons.53–55 Kan et al. reported that the concentration of DF in 26 varieties from China ranged from 29.32% (MGWJD cultivar, Meiguowujiadou) to 46.77% (LLYHJD cultivar, Lvlongyihaojiadou), and the concentration of RS was in the range of 9.16% (LLS cultivar, Laolaishao) to 18.09% (CJJDW cultivar, Chaojijiadouwang).55 As observed by Fatema et al., the DF content in kidney beans in Sylhet was only about 2.08% to 2.46%, was higher than that in the Bandarban regions from Bangladesh.56 These results imply the importance of the genetic background for carbohydrate accumulation in kidney beans. Besides, geographical locations may serve as another main reason for the divergence of carbohydrate content. The DF content in red kidney beans from Japan was 15.90% higher than that from Bangladesh, but lower than the level in China.11,55,56 Comparatively, a much higher average RS content of 45.23% was observed in the Córdoba region compared to the 30.74% in the Cabrela region in 106 kidney bean accessions from Portugal.57 Heat stress exposure in Córdoba may contribute to the higher RS content than the mild climate environment in Cabrela. The above-mentioned observations suggest that both the genotype and planting environment influence the carbohydrate content and distribution, and particularly heat stress contributes to RS accumulation during seed filling.
Table 2 The composition and content of carbohydrates in different cultivars of kidney beans
Cultivars |
Carbohydrates composition and content (%) |
Parts of kidney beans |
Biological activity or processing adaptability |
Place of origin |
Ref. |
DF: dietary fiber; GOS: galacto-oligosaccharides; SDF: soluble dietary fiber; IDF: insoluble dietary fiber; RFO: raffinose oligosaccharides; TDF: total dietary fiber; NRS: non-resistant starch. |
Pinto, yellow kidney beans and red haricot beans |
63.00–66.00 |
Whole bean |
— |
Nairobi, Kenya |
49
|
Black beans (Phaseolus vulgaris L.) |
RS (6.53), DF (20.17), α-GOS (0.50) |
Cotyledon |
Carbohydrate extract as a thickener for pudding, soy sauce, cream or dairy products |
Zacatecas, Mexico |
58
|
18 germplasms |
57.96–63.39 |
Whole bean |
— |
Sylhet and Bandarban, Bangladesh |
56
|
Common beans (P. vulgaris L.) cv. Negro 8025 |
SDF (3.34), IDF (25.07), RS (33.00), oligosaccharides (5.00) |
Whole bean |
Fermentation to produce short-chain fatty acids |
Guanajuato, Mexico |
50
|
26 varieties |
TDF (29.32–46.77), RS (9.16–18.09), NRS (1.57–6.76), starch (11.36–23.44) |
Whole bean |
Dietary source of RS for various food applications and has good antioxidant activity |
Shandong and Jiangxi Provinces, China |
55
|
9 varieties (EC-590329, EC-572723, EC-590326, Pi-244719, Pi-312296, Pi-249554, PLB-10-1, PLB-14-1 and IC-382213) |
Total carbohydrate (66.40–70.90), starch (34.00–56.00) |
Whole bean |
Non-starch carbohydrates reduce the digestibility of starch and dry matter |
Shimla, India |
47
|
5 varieties (Mona, Alamo, Flaforte, Igołomska, and Laponia) |
Total soluble sugars (2.45–3.18), total RFO (0.62–1.32) |
Whole bean |
— |
Krakow, Poland |
59
|
Red kidney bean (Taisho kintoki) |
DF (15.90), RS (34.10) |
Whole bean |
Prevention of liver injury; both roasting and boiling reduce the RS content (27.6% and 3.2%) |
Hokkaido, Japan |
11
|
In addition, processing methods should also be considered to evaluate the carbohydrate contents and digestibility available for human nutrition by kidney beans. Dry-heat treatment can better preserve the RS content than heat-moisture treatment in kidney beans. For example, although both roasting (180 °C for 10 min) and boiling (50 min) could reduce the RS content in red kidney beans, where roasting with a shorter cooking time caused less changes than boiling. This is attributed to the fact that roasting treatment well preserved the starch granules, while boiling aggravated starch gelatinization and damaged the integrity of the starch granules.11 The integrity of starch granules seems to be the crucial factor in the content of RS. Similarly, under the same cooking time, autoclaving at a higher temperature of 121 °C (26.60%) led to a greater reduction in RC than at 100 °C (14.40%), whereas extrusion treatment caused the highest RS reduction of 54.20%. The shear and high temperature during extrusion converted the smooth starch granules to a flattened appearance, leading the greater loss of RS. Besides, α-galacto-oligosaccharides (α-GOS) was almost completely lost with extrusion treatment but not autoclaving, which is attributed to the hydrolysis of GOS to disaccharides and monosaccharides.58 Other oligosaccharides in kidney beans, mainly the raffinose family of oligosaccharides (RFOs), were also significantly reduced under boiling and sterilization.59 Significantly, for some kidney bean varieties, these prebiotic carbohydrates including RS and RFOs may further be lowered after cooling and reheating.60 Therefore, it is of critical importance to understand the alteration in the different types of carbohydrates during processing in kidney beans. Mechanically, an extended thermal processing time may increase the cell permeability, allowing more access to pancreatic α-amylase, and thus increasing the digestibility of the enclosed starch in kidney beans.24
3.2 Proteins
Kidney beans are an important source of plant proteins, with a content in the range of 21.70–32.63% (Table 3).47,52,55 As reported, dark-colored kidney beans usually contain a higher amount of protein than light-colored beans. A comparative study conducted by Wang et al. showed that dark-red kidney beans had the highest protein content (27.06 g per 100 g dw), whereas spotted beans (22.40 g per 100 g dw) had the lowest protein content.61 The protein content in red kidney beans (26.00 g per 100 g dw) was also higher than that of yellow kidney beans (22.30 g per 100 g dw).62 Black kidney beans had a protein content of 25.90 g per 100 g dw, which was higher than that of white kidney beans in the range of 21.44–22.85 g per 100 g dw. An exception was found in red kidney beans, which achieved a similar protein content of 19.80 and 20.09 g per 100 g dw.63,64 According to these results, the direct correlation between protein content and seed color remains controversial, and other factors may function as co-factors that influence the protein content in kidney beans.
Table 3 The composition and content of protein in different cultivars of kidney beans
Cultivars |
Content (%) |
Parts of kidney beans |
Processing adaptability |
Place of origin |
Ref. |
Common black beans (Phaseolus vulgaris L.) |
23.10 |
Whole bean |
Good emulsification stability and antioxidant activity |
Pelotas, Brazil |
67
|
Red kidney beans (Phaseolus vulgaris) |
— |
Whole bean |
Kidney bean protein isolate, foaming properties, and emulsifying properties |
India |
14
|
10 common beans (P. vulgaris L.) |
19.50–31.30 |
Whole bean |
Nutrient enrichment applications, prevention and treatment of diet-dependent diseases |
Cabrela (near Sintra, Portugal) |
63
|
Navy beans (P. vulgaris) |
23.10 |
Whole bean |
Good digestibility and solubility |
New Zealand |
66
|
Beans (P. vulgaris) |
21.00 |
— |
Strong potential for antioxidation, anti-genotoxicity, and chemical prevention in vivo |
San Juan del Rio, Mexico |
38
|
26 varieties of kidney beans |
22.06–32.63 |
Whole bean |
— |
Shandong and Jiangxi Province, China |
55
|
Dark red kidney beans (Phaseolus vulgaris) |
28.31 |
Whole bean |
Antioxidant or food additive |
Sylhet, Bangladesh |
52
|
Nine KB (EC-590329, EC-572723, EC-590326, Pi-244719, Pi-312296, Pi-249554, PLB-10-1, PLB-14-1 and IC-382213) |
21.70–26.90 |
Whole bean |
Hydration ability |
Shimla, India |
47
|
3 cultivars (P. vulgaris L. cv. French Yellow, Contendor, Master Bean) |
21.80–26.20 |
Whole bean |
— |
Shalimar, India |
62
|
7 kidney beans (P. vulgaris L.) |
— |
Whole bean |
Good water holding capacity and can be used as food packaging material |
Heilongjiang and Guizhou Province, China |
9
|
Rainfall and temperature can affect the protein content in kidney beans, for example, it varied annually due to climatic parameters, with the levels in 2014 (22.00–31.30 g per 100 g dw) being significantly higher than that in 2013 (19.50–24.80 g per 100 g dw).63 More specifically, the protein content showed an increase under drought conditions and heat stress. For example, Hummel et al. showed that the protein content in 20 cultivars of kidney beans grown under drought stress was 12.13% higher than that grown in a rainfed field. The difference caused by environmental condition was demonstrated to be higher than that caused by the kidney bean genotype.65 Therefore, the protein content in kidney beans grown in the Cabrela region, which has a mild climate, was 11.85% lower than in the Córdoba region, which is susceptible to heat stress.57
The proteins in kidney beans are classified as prolamins, globulins, legumin, and alcohol-soluble glutenins.9,66 The crude prolamins extracted from kidney beans have a higher sulfhydryl content, water holding capacity, and stronger hydrophobicity, suggesting their potential as food packaging materials.9 However, the properties of kidney bean proteins are influenced by their processing methods. For example, under conventional heat treatment, the abundance of kidney bean proteins significantly decreased (58.50%), most of which were the high-molecular-weight protein isomers globulin, legumin, and lipoxygenase. Meanwhile, the abundance of low-molecular-weight protein isomers, i.e., lysinoalanine significantly increased (47.00%) correspondingly.66 As an alternative treatment to heat, increasing the pressure to a moderate level, i.e., 600 MPa, could induce protein unfolding and exposure of water binding sites, thus increasing the water holding capacity and emulsification of kidney bean proteins. Meanwhile, the increase in pressure partially denatured the molecular structure of the protein, resulting in a significant decrease in foaming capacity.14 However, enzymatic treatment was reported to improve the kidney bean protein qualities but it was dependent on the enzyme source. Pepsin-treated kidney bean protein hydrolysates showed a higher hydrolysis degree than Alcalase digestion. However, Alcalase treatment resulted in the formation of smaller droplets, reducing the coalescence of the droplets to improve the stability of the emulsion. Moreover, the increase in protein flexibility and exposure of the aromatic site by Alcalase treatment may induce higher surface hydrophobicity, leading to the stronger binding capacity of ABTS radicals than pepsin treatment.67
3.3 Phenolic compounds
Phenolic compounds are the main contributor to antioxidant activity, which are mainly found in the seed coats of kidney beans, with a range of 0.25–5.43 mg gallic acid (GAE) per gram (Table 4).55,68,69 The antioxidant activity of phenolic compounds is correlated with the total phenolic content (TPC).69 In general, white kidney beans have a lower TPC compared to colored beans.70 In a comparative study of 27 types of kidney beans, the TPC of red kidney beans ranked as the highest, with value of up to 5.43 mg GAE per g dw.69 In the case of the same color, the variety of kidney beans also has an impact on their TPC. Orak et al. found the highest TPC of 0.63 and 0.61 mg GAE per g for Göynük and Göksun among 10 white kidney beans, while the lowest TPC of 0.34 and 0.33 for Akman and Zülbiye, respectively.71 Therefore, the seed color and kidney bean cultivars are both important in the selection of candidates with high TPC.
Table 4 The composition and content of phenolic compounds in different cultivars of kidney beans
Kidney bean cultivars |
Composition and content |
Parts of kidney beans |
Antioxidant activity |
Place of origin |
Ref. |
GAE (gallic acid equivalents); CE (catechin equivalents); C3G (cynidin-3-glucoside); RT (rutin); QE (quercetin equivalent); TE (trolox equivalent); TEAC (TE antioxidant capacity). |
26 varieties of kidney beans |
Polyphenol (27.7–127.0 mg GAE per g), flavonoids (5.9–21.5 mg CE per g) |
Seed coat |
132.5–1021.7 μmol ETrolox per g |
Oaxaca, Guerrero, Puebla, Tlaxcala and Estado de Mexico, Mexico |
68
|
Polyphenol (1.3–5.4 mg GAE per g), flavonoids (0.1–0.78 mg CE per g), anthocyanin (0.02–9.07 mg C3G per g) |
Whole bean |
0.65–32.4 μmol ETrolox per g |
54 native bean populations and 5 improved varieties of kidney beans |
Polyphenol (1.0–182.9 mg GAE per g), flavonoids (0.18–20.77 mg CE per g), anthocyanin (0.00–6.71 C3G per g) |
Seed coat |
19.8–1569.9 μmol Trolox per g |
Oaxaca, Mexico |
75
|
Polyphenol (1.21–2.77 mg GAE per g), flavonoids (0.25–2.34 mg CE per g) |
Cotyledon |
3.7–73.1 μmol Trolox per g |
26 varieties of kidney beans |
Polyphenol (0.25–3.79 mg GAE per g), flavonoids (0.19–4.35 mg RT per g) |
Whole bean |
12.08–99.36 μmol Fe(II) equivalent per g |
Shandong and Jiangxi Province, China |
43
|
31 different traditional common bean varieties |
Polyphenol (1.38–5.12 mg GAE per g), flavonoids (0.14–2.01 mg CE per g), total proanthocyanidins (0.02–0.70 mg CE per g) |
Whole bean |
37.35–154.83 μmol TEAC per g |
Coimbra, Portugal |
70
|
Polyphenol (0.04–3.77 mg GAE per g), flavonoids (0.01–2.36 mg CE per g), total proanthocyanidins (0.01–2.60 mg CE per g) |
Seed coat |
— |
Polyphenol (0.87–1.06 mg GAE per g), flavonoids (0.15–0.23 mg CE per g) |
Cotyledon |
— |
10 varieties of seeds from white bean (Phaseolus vulgaris L.) |
Polyphenol (0.33–0.63 mg GAE per g) |
Whole bean |
3.50–5.17 μmol Trolox per g |
Tekirdağ, Turkey |
71
|
White common bean (Cultivar Longquanji-uli) |
Polyphenol (6.57 mg GAE per g) |
Whole bean |
— |
Harbin, China |
107
|
4 varieties of P. vulgaris L. (kidney bean, pinto bean, black bean and borlotti bean) |
Polyphenol (0.58–1.36 mg GAE per g), flavonoids (0.24–0.69 mg CE per g) |
Whole bean |
4.47–10.37 μmol TE per g |
Portuguese |
74
|
The phenolic compounds in kidney beans mainly include phenolic acids, flavonoids, and anthocyanins.41,68 Flavonoids were mainly found in the seed coats and phenolic acids were distributed in the cotyledons.70 In a comparative study, red kidney beans showed the highest level of flavonoids at 1325.00 μg catechin equivalent (CE) per g, while the lowest level of flavonoids at 106.00 μg CE per g was detected in white kidney beans, and black kidney beans showed the highest level at anthocyanins of 440.00 μg cyanidin-3-O-glucoside equivalent (C3G) per g compared to other kidney beans.72 As reported, the content and composition of phenolic compounds are largely influenced by the processing methods, such as soaking and heating. Although soaking usually causes the loss of phenolic compounds to the water because of the leaching process, the overall content of TPC in soaked beans remained higher than the non-soaked bean flour, which is possibly due to the breakdown of the phenolic–protein interaction.70 Nevertheless, the heating effects still remain controversial. For example, Hernández-Saavedra et al. found that heat treatment significantly decreased the TPC, especially tannins and anthocyanins in kidney beans. This can be explained by the fact that these substances form insoluble complexes with proteins, making them impossible to extract.73 However, in most studies, heat treatment increased the TPC, including mainly the gallic acid, catechin, and kaempferol content.74
Furthermore, other factors including geographical location and growing environment should also be considered. In a comparative study of phenolic compounds in kidney beans from five states and regions in Mexico, Oaxaca, and Puebla were found to have higher levels of anthocyanins.68 The kidney beans grown in Sierra Norte had the highest level of polyphenols (104.70 mg GAE per g) and anthocyanins (1.80 mg C3G per g), while the anthocyanin level in the kidney beans grown in Mixteca was only 0.93 mg C3G per g. In addition, the average level of polyphenols and anthocyanins was higher in the kidney beans grown in 2014 (96.70 mg GAE per g and 1.36 mg C3G per g) than in 2015 (73.70 mg GAE per g and 1.26 mg C3G per g), whereas the flavonoid content was higher in 2015 (12.10 mg CE per g dw).75
4. Interactions between kidney bean NSPs and other molecules
Considering complete food, it is important to understand the interactions between food nutritional molecules (Fig. 2).76 In general, extracted kidney bean NSPs also contain a low amount of protein (9.00–28.70%), and the protein is bound to the NSP chains in the form of glycoproteins.16,24 However, the existing interactions between NSPs and proteins affect their physicochemical properties.54 As suggested, GalA and Ara of kidney bean NSPs may interact with proteins to form a stabilizing layer that prevents protein aggregation, thus significantly improving the stability of NSP emulsions.16 NSP and protein complexes could also constitute a barrier enclosing starch granules to decrease starch digestion.77 In addition, through hydrophobic interactions, the addition of kidney bean NSPs together with ultrasonic treatment could increase the gel strength and water holding capacity of duck myofibrillar proteins.78
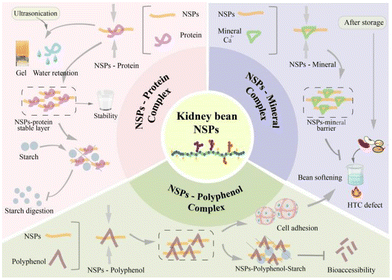 |
| Fig. 2 Interactions between kidney bean NSPs and other molecules. Kidney bean NSP–protein interactions enhance the emulsion stability and form a barrier to slow starch digestion, and ultrasound-assisted treatment enhances the gel strength and water holding capacity. NSP–polyphenol interactions enhance cellular adhesion and drive the development of the HTC defect. NSP–polyphenol–starch interactions inhibit the bioaccessibility of polyphenols. NSP–mineral interactions form a barrier that leads to the HTC defect, which is strengthened with storage. | |
Kidney bean NSPs do not only interact with proteins, they can also form complexes with polyphenols through covalent, hydrogen, and hydrophobic interactions, or cross linked by ester bonds.79 Evidence has shown that polyphenols can be detected in both raw and cooked bean NSPs, accounting for approximately 3.70–23.80% of the NSPs fraction, with hydroxybenzoic acid being the most abundant.80 As reported, arabinan and galactan residues of pectic NSPs may contribute to their interaction with polyphenols, such as ferulic acid.81 The cross linkages between NSPs and polyphenols can mutually influence their physicochemical and nutritional properties.82 The study by Perez-Hernandez et al. indicated that the bioaccessibility of polyphenols is restricted due to their interaction with NSPs and starches. After only the hydrolysis of NSPs and digestion of starches, the greater release of polyphenols was observed.83 In kidney beans, the interaction between pectin and polyphenols may also enhance the cell adhesion and contribute to the hardening process during storage, thereby leading to the development of HTC defects.28
During the development of HTC defects in kidney beans, the interactions between NSPs and minerals cannot be excluded, in particular Ca2+. Increasing evidence suggests that the cross linkages of pectin–cation–phytate may be responsible for the development of HTC defects.6,84 The main phenomenon of HTC defects is delayed softening during thermal cooking of kidney beans. Koriyama et al. showed that kidney bean NSPs of hemicellulose, cellulose, and cell wall polysaccharides would interact with Ca2+, thus delaying the softening process of the beans.85 Rousseau et al. also demonstrated that kidney bean pectins may covalently be cross-linked with minerals and preferentially bind to Ca2+, thus forming a strong barrier.86 These interactions are more significant with storage, which is attributed to the increased ratio of divalent cation pectins and free carboxyl groups, suggesting the stronger HTC defect after storage.87
5. NSP extraction and structural properties of kidney beans
5.1 Extraction, isolation and purification of kidney bean NSPs
As mentioned above, the NSPs in kidney beans mainly consist of IDF such as cellulose and SDF such as pectins.16 Ratnawati et al. investigated the content of NSPs in soybeans, mung beans, and red kidney beans, among which, red kidney beans exhibited the highest NSP content of 23.80%.15 However, the content of NSPs in kidney beans varies among cultivars. Kan et al. reported that the NSP content in 26 species of kidney beans was in the range of 29.32–46.77%, of which the MGWJD cultivar had the lowest NSP content of 29.32%, while the LLYHJD cultivar achieved the highest NSP content of 46.77%.55 Campos-Vega et al. extracted NSPs from four kidney bean cultivars, including Negro 8025, Pinto Durango, Bayo Madero, and Azufrado Higuera. Negro 8025 had the highest NSP content of 37.60%, whereas Bayo Madero had the lowest NSP content of 25.60%. Furthermore, cooking could significantly improve the content of NSPs, and the percentage observed for Bayo Madero was the highest, which totaled up to 55.00%.88
Besides considering the cultivars, the extraction methods also influence the yield and molecular weight of kidney bean NSPs (Table 5). Currently, hot water extraction and ethanol precipitation are the most common NSP extraction methods, with the advantages of low cost, no contamination, and simple and safe operation. For example, Patra et al. used continuous hot water immersion for 12 h and ethanol precipitation to extract water-soluble pectin polysaccharides from kidney bean pods, which exhibited a molecular weight of 180 kDa.89 To reduce the extraction time, acidic or alkaline conditions are also optimized to release intracellular polysaccharides.23 Lower pH values can result in a higher yield under acidic extraction. This can be attributed to the breakdown of the glycosidic bonds to allow the greater release of polysaccharides with more hydrogen ions.90 Although alkaline extraction results in a higher yield than acidic, it usually requires higher temperature. For example, an optimal pH value of pH 9 was chosen in successive pH values from pH 3–11, with the optimized temperature of 120 °C for 30 min.16 Meanwhile, given that kidney beans are rich in starches and proteins, enzymatic approaches are applied to remove these confounding factors.23 Thermostable α-amylase is usually applied, followed by protease or papain, glucoamylase or amyloglucosidase.20,88,91 However, to improve the purity of NSPs, the pigment substances of kidney beans with different colors should not be ignored, which need further investigation.
Table 5 The extraction, purification methods, yield, and chemical structure of kidney bean NSPs
Kidney bean cultivars |
Extraction methods |
Purification methods |
NSP yield (%) |
NSP purity/content |
NSP molecular weight |
Monosaccharide composition |
Structural characterization method |
Place of origin |
Ref. |
GLC-MS: gas–liquid chromatograph-mass; NMR: nuclear magnetic resonance; HPSEC-MALLS-RID: high-performance size exclusion chromatography (with a multi-angle laser light scattering and refractive index); FT-IR: Fourier-transform infrared; GC-MS: gas chromatography-mass spectrometry; HPAEC: high performance anion exchange chromatography; HPLC: high performance liquid chromatography; SEC-MALS: size exclusion chromatography (with a multi-angle laser light scattering detector); HPGPC: high-performance gel permeation chromatography; HPAEC-PAD: high-performance anion-exchange chromatography with pulsed amperometric detection; SEM: scanning electron microscope. |
Green bean (P. vulgaris L.) |
Water extraction and alcohol deposition |
Gel column (Sepharose 6B) |
— |
Pectin speculation |
180–200 kDa |
GalA : Gal : Ara = 2 : 2 : 1 (molar ratio) |
GLC, GLC-MS, NMR |
West Bengal, India |
89
|
White common bean |
Ethanol, enzyme-assisted water extraction |
DEAE Sepharose Fast Flow column |
1.36 |
96.45% |
2910 kDa |
Glc (62.00%), Gal (29.00%), a few of Ara and Xyl |
HPSEC-MALLS-RID, FT-IR, GC-MS |
Daqing, China |
97
|
95.88% |
4570 kDa |
Gal (52.00%), Ara (30.00%), a few of Glc and Xyl |
Negro 8025, Pinto Durango, Bayo Madero, Azufrado Higuera |
Hot water, chemically assisted enzymatic extraction |
— |
48.00, 36.50, 55.00, 42.20 |
55.00%, 54.30%, 52.50%, 64.30% |
— |
— |
— |
Celaya, Mexico |
88
|
Common beans (P. vulgaris L., cv. Carioca-Perola) |
Chemically assisted enzymatic extraction |
Anion exchange column (Q-Sepharose Fast Flow) |
— |
Pectin 7.4%, cellulose 2.3% |
1576–3505 kDa |
Ara (42.00%), UA (13.00%), Man (13.00%), Gal (11.00%), Xyl (11.00%), Glc (7.00%), Rhm (2.00%) and Fuc (0.60%) |
HPAEC, HPLC, GC-MS |
Brazil |
99
|
White kidney beans |
Enzyme-assisted hot water extraction |
— |
26.40 |
— |
2530 kg mol−1 |
Ara (67.20%), GalA (15.60%), Gal (7.60%), Xyl (6.10%), Rha (3.50%) |
HPLC, GC-FID/MS, SEC-MALS |
Canada |
16
|
White kidney beans |
Enzyme-assisted hot water extraction |
Sevag reagent (chloroform/n-butanol (4 : 1, v/v)) |
— |
Crude NSP |
13 kDa |
Fuc : Ara : Gal : Glc : Man : Fru : GalA = 0.52 : 0.30 : 5.70 : 12.49 : 1.95 : 1.28 : 0.25 |
HPGPC, HPAEC-PAD |
Shanxi Province, China |
20
|
Red kidney beans |
1322 kDa |
Fuc : Ara : Gal : Glc : Xyl : Man : GalA = 0.28 : 7.00 : 5.12 : 9.16 : 2.33 : 2.20 : 0.96 |
White kidney beans |
Enzyme-assisted hot water extraction |
DEAE Sepharose Fast Flow column |
11.00 |
Pectin speculation |
12 kg mol−1 |
Ara (10.50%), Xyl (13.10%), Rha (2.50%), Glu (2.40%), Gal (71.40%) |
HPGPC, HPAEC-PAD, FT-IR, SEM, HPSEC-MALLS-RI, GC-MS, NMR |
Shanxi Province, China |
91
|
10.50 |
40 kg mol−1 |
Ara (30.00), Xyl (18.90), Rha (3.10), Glu (1.40), Gal (46.70) |
10.80 |
450 kg mol−1 |
Ara (51.30%), Xyl (7.00%), Rha (4.80%), Gal (36.20%) |
Pinto bean (P. vulgaris cv. Pinto) |
Citric acid–phosphate buffer extraction |
— |
38.50 |
— |
270.60 kDa |
Man (2.50%), GalA (15.00%), Rha (4.00%), Glu (9.00%), Gal (62.20%), Xyl (2.90%) and Ara (4.30%), trace amount of Rib and Fuc |
— |
Penang, Malaysia |
90
|
Common beans (P. vulgaris cv. Rose coco) |
Enzyme-assisted ethanol extraction |
80% ethanol (2 times) and acetone (1 time) |
— |
10.71% pectin, 6.24% H + S |
— |
Xyl (69.40–79.30 mg g−1), GalA (57.3–68.8 mg g−1), Ara (21.3–28.2 mg g−1) |
HPAEC, HPAEC-PAD, HPSEC, MALLS |
Kenya |
87
|
— |
10.56% pectin, 6.69% H + S |
— |
9.30% pectin, 12.77% H + S |
Ara (83.0–108.5 mg g−1), GalA (31.5–50.85 mg g−1), Gal (22.6–32.8 mg g−1), Xyl (22.1–36.1 mg g−1) |
— |
11.27 pectin, 15.29% H + S |
5.2 Structural characteristics of kidney bean NSPs
Besides the yield of NSPs, it is very important to understand their structural characteristics due to their critical role in the food industry and physiological functions. As mentioned before, SDF in kidney beans is dominated by pectins, which are mainly distributed in the cell wall.16 In general, kidney bean pectins have homogalacturonan (HG), xylogalacturonan (XGA), and rhamnogalacturonan-I (RG-I) in their backbone, and arabinose (Ara) or galactose (Gal) as side chains.92 HG and XGA are composed of linear polymers of α-1,4-linked galacturonic acid, which is partially methylated at C-6.16 HG and XGA are typically referred to as the “smooth region” due to their linear structure,92,93 while RG-I consists of a backbone composed of D-galacturonic acid and L-rhamnose, while belonging to the “hairy region” and containing abundant side chains including D-Gal and L-Ara (Fig. 3).91 The proportions of HG and RG-I in kidney bean pectins were 60.00% and 15.00–30.00%, respectively.91 Nevertheless, these proportions of HG and RG-I in pectins varied depending on the source. For instance, apple pectins contained proportions of 53.00% of HG and 26.94% of RG-I,94 while potato pectins exhibited a substantial proportion of RG-I region of 75.00% but a relatively low HG region of 20.00%.95
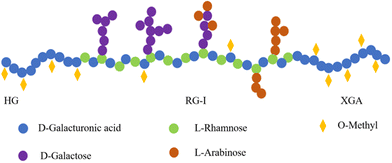 |
| Fig. 3 A schematic representation of the structure of kidney bean NSPs. | |
The structural characteristics of kidney bean NSPs are highly influenced by the extraction and purification methods (Table 5). For example, Yi et al. found that NSPs in red kidney bean cell walls extracted by water and chelator were dominated by GalA, while that extracted using Na2CO3 was Xyl-dominated.87 Interestingly, Chen et al. performed sequential pectin extractions from red kidney beans using each of the above-mentioned methods and found that all three pectins were Ara-dominated, with the highest pectin content (9.54%) in water extract, which was mainly RG-I pectin.96 When the hot water extraction method assisted with enzymes was employed, NSPs were mainly composed of Ara (67.20%) and GalA (15.60%), with a mixed structure of a multi-branched structure and a less branched straight chain structure.16 Tang et al. isolated three water-soluble pectic polysaccharides, namely, WKBP-P2, P3, and P4, from white kidney beans using the ion exchange and particle size exclusion methods, and found that all three pectins consisted of structural regions of HG, XGA, and RG-I. Notably, the molecular sizes and molar ratios of three pectins differed significantly, with P2 being dominated by HG and containing small amounts of RG-I and XGA, P3 mainly containing XGA and HG, and small amounts of RG-I, while P4 being dominated by RG-I, with the largest amount of Ara (51.30%).91 The choice of purification column also affected the structural characteristics of kidney bean NSPs.97
The yield and structural characteristics of NSPs from different parts of kidney beans are also different. Chigwedere et al. applied water, chelator, and sodium carbonate to extract pectins (WEP, CEP and NEP, respectively) from kidney bean cotyledons and seed coats. The CEP content in the cotyledons was only 58% of that in the seed coats, and the NEP of the seed coats was 78% of that in the cotyledons, while the WEP content in the cotyledons and seed coats remained similar. The pectin in the cotyledons showed a higher degree of methylation (DM) than that in the seed coats, and was more branched.98 Similar observations were also reported in CEP and alcohol insoluble residue of kidney beans by Yi et al. Despite of the extraction methods, Yi also reported that kidney bean NSPs isolated from cotyledons mainly contained Ara with a content in the range of 83.0–108.5 mg g−1, followed by GalA, Gal, and Xyl.87 However, NSPs from seed coat showed a contrast composition, which mainly contained 69.40–79.30 mg g−1 of Xyl, followed by GalA and Ara. In the case of the enzymatic-assisted extraction of kidney bean NSPs, the cotyledons contained high amounts of Ara, GalA, and β-glucan, while the seed coat contained more 1,4-linked Xyl and 1,5-linked Ara.99
6. Nutritional properties of kidney bean NSPs
6.1 Intestinal health
As mentioned before, legume NSPs have attracted attention worldwide due to their regulatory and protective effects on the gut microbiota (Fig. 4).100 For example, kidney bean NSPs (33.60 g kg−1 mice weight) could increase the relative abundance of the Actinobacteria and Bacteroidetes phyla in the intestine of mice, while the ratio of Firmicutes/Bacteroidetes decreased, promoting the production of short chain fatty acids (SCFAs) (Table 6).101 A similar study also showed that red kidney bean NSPs (1% w/v, NSPs/medium) significantly promoted beneficial intestinal flora, especially Bifidobacterium and Lactobacillus, and increased the concentration of SCFAs, while inhibiting the formation of E. coli biofilm by 79%.17 The differences in the growth performance between beneficial and enteric bacteria by kidney bean NSPs may rely on the production of glycolytic enzymes. Compared to enteric bacteria, beneficial bacteria possess stronger glycolytic activity, leading to the higher breakdown of the glycoside bonds in NSPs and greater utilization of NSPs.102 Furthermore, the utilization extent of NSPs by beneficial bacteria seems to be associated with the integrity of the bean cells. In an in vitro fermentation study, Rovalino-Córdova et al. found NSPs (26.66% w/w, NSPs/diet) isolated from red kidney bean intact cotyledon cells and mechanically damaged cells, both tremendously increasing the abundance of Bifidobacterium species. Alternatively, the fermentation of mechanically damaged cell-derived NSPs exhibited higher amounts of SCFAs.103 Besides, the origin of NSPs also impact their utilization by the gut microbiota. Wu et al. found that the fermentation of NSPs in black kidney beans produced more SCFAs from Lactobacillus plantarum than red kidney beans and speckled kidney beans. The monosaccharide composition, molecular weight, and glycosidic linkages may all contribute to the fact that the carbohydrate utilization of kidney bean NSPs is cultivar dependent.103,104
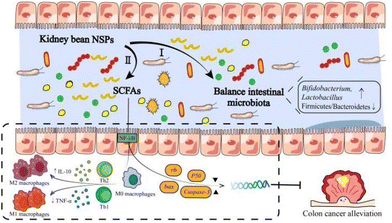 |
| Fig. 4 The modulatory effects of kidney bean NSPs on human intestinal health. I: Kidney bean NSPs can modulate the composition of the intestinal microbiota and significantly promote the growth of beneficial intestinal microbiota, especially Bifidobacterium and Lactobacillus, while decreasing the growth of Firmicutes/Bacteroidetes. II: Kidney bean NSPs can be fermented by the intestinal microbiota to produce SCFAs, mainly acetate and butyrate. Through fermentation, kidney bean NSPs affect the expression of colon cancer-related genes, i.e. bax, caspase-3, and rb, meanwhile alleviating inflammation by enhancing anti-inflammatory cytokine IL-10 and decreasing pro-inflammatory cytokine TNF-α responses. In addition, they play a positive role in improving the functioning of the intestinal barrier. | |
Table 6 Nutritional characterization of kidney bean NSPs
Kidney bean cultivars |
Application form |
NSP component |
Applied dosage |
Nutritional properties |
Ref. |
Brown bean (P. vulgaris L. var. Katja) |
NSP fraction incorporated in mice mouse experimental diets |
Ara (36.00%), Glc (29.00%), UA (13.00%), Xyl (10.00%), Gal (7.00%), Fuc (3.00%) |
33.60 g kg−1 mice weight |
Prebiotics |
101
|
Red kidney bean (P. vulgaris L.) |
NSP extract |
— |
1% w/v (NSPs/medium) |
Prebiotics and anti-pathogens |
17
|
Red kidney bean |
NSP extract |
Fuc (0.98%), Ara (12.13%), Rha (0.40%), Gal (2.44%), Glc (21.59%), Xyl (4.41%), Man (1.04%), UA (1.46%) |
26.66% w/w (NSPs/medium) |
Prebiotics |
103
|
Common bean (P. vulgaris L.) |
NSP fraction incorporated in rat experimental diets |
— |
1.84 g kg−1 rats weight |
Anti-colon cancer and prebiotics |
50
|
Common bean (P. vulgaris) |
NSP extract |
— |
1% w/v (NSPs/medium) |
Anti-obesity and prebiotics |
19
|
Black bean (P. vulgaris) |
Whole bean as NSP source for human breakfast meal |
— |
19.26 g NSPs per meal |
Increase satiety and anti-obesity |
109
|
White kidney beans |
NSP extract |
— |
0.1 g kg−1, 0.2 g kg−1 rats weight |
Anti-diabetic and attenuate liver damage |
110
|
White kidney bean, red kidney bean (P. vulgaris) |
NSP fraction incorporated in mice rat experimental diets |
Neutral sugar (53.16–54.73%), UA (6.35–6.78%) |
0.5 g kg−1 mice weight |
Anti-diabetic |
20
|
Red kidney bean |
NSP fraction incorporated in mice rat experimental diets |
— |
0.1 g kg−1, 0.2 g kg−1, 0.4 g kg−1 rats weight |
Anti-diabetic and improve liver function |
113
|
Besides the regulatory effects on the gut microbiota composition by kidney bean NSPs, there are also many studies showing the colon cancer alleviation effect through the production of SCFAs (Fig. 4). For example, Feregrino-Perez et al. found that kidney bean NSPs (1.84 g kg−1 rats weight) can be fermented by the gut microbiota to produce SCFAs, dominated by butyrate. Through fermentation, kidney bean NSPs inhibited the increase in abnormal crypt foci and affected the expression of colon cancer-related genes, i.e., bax, caspase-3, and rb, which implies the effect of butyrate on the apoptotic process mediated by mitochondria mechanisms.50 Campos-Vega et al. also found that the expression of 72 of the 84 p53 signaling genes was significantly altered in HT-29 cells by in vitro fermentation of kidney bean NSPs, in addition to the production of butyrate during colonic fermentation.105 Furthermore, the colon cancer alleviation effect may extend to the non-digestible fraction of fermented kidney beans.105 Compared to the sole function of SCFAs produced by NSP fermentation, the synergistic effect with phenolic compounds and biopeptides exerted stronger effects in regulating the expression of the p53 pathway anti-proliferative and pro-apoptotic genes in HT-29 cells, which implies a mechanism by which other nutritional components are integrated with NSPs.106
6.2 Anti-obesity
Kidney bean NSPs can inhibit obesity in several ways, including gut microbiota fermentation (Fig. 5).107 Many studies have shown that obesity is usually associated with an increased ratio of Firmicutes/Bacteroidetes, accompanied by dysbiosis of the gut microbiota metabolism.108 By serving as food for the gut microbiota, kidney bean-derived NSPs can regulate gut microbial metabolites, inhibit lipid accumulation, and enhance energy expenditure. For example, in an in vitro study, Lu et al. found that the fermentation of kidney bean NSPs (1% w/v, NSPs/medium) by healthy mouse fecal inoculum enhanced the concentration of SCFAs and increased the production of acetate in particular. However, the fermentation product mixture but not the acetate or NSPs were later proven to suppress the differentiation of 3T3-L1 adipocytes and maturation by inhibiting the expression of CCAAT/enhancer binding protein α (C/EBPα) and peroxisome proliferator-activated receptor γ (PPARγ) and the expression of fatty acid binding protein 4 (FABP4) after adipocyte maturation, and enhance energy expenditure by activating PPARδ and mitochondrial uncoupling protein 2 (UCP2).19 All these results indicate that the anti-obesity effect of kidney bean NSPs is dependent on gut microbiota fermentation.
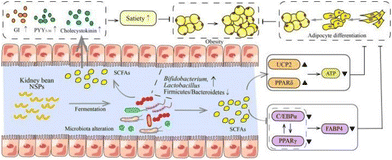 |
| Fig. 5 The anti-obesity effects of kidney bean NSPs. Kidney bean NSPs can inhibit obesity by regulating the intestinal microbiota and microbial metabolites, i.e. SCFAs. SCFAs can inhibit adipocyte differentiation through the activation of the energy expenditure pathway and the inhibition of C/EBPα, PPARγ, and FABP4, thereby inhibiting obesity. In addition, kidney bean NSPs can inhibit obesity by enhancing satiety through the up-regulation of the GI hormone, PYY3-36, and cholecystokinin production. | |
Kidney bean NSPs may also inhibit obesity by promoting satiety. Reverri et al. evaluated the effects of black P. vulgaris as the only source of intrinsic fiber, with added fiber as the extrinsic fiber (19.26 g NSPs per meal) on satiety in obese adults with metabolic syndrome. Although extrinsic fiber suppressed prospective consumption, GI hormone secretion was not influenced, suggesting that other neuroendocrine peptides may be involved. Intrinsic fiber supplementation resulted in higher GI hormone and peptide tyrosine (PYY3-36) response, and higher beneficial metabolites, i.e., cholecystokinin production, demonstrating the role of the whole kidney bean as an intrinsic fiber source in losing weight.109 The effects were demonstrated to be mainly achieved through the fermentation products of SCFAs by the gut microbiota in the colon.110
6.3 Anti-diabetes
Supplementation of kidney bean NSPs as a therapeutic strategy for diabetic patients has attracted significant attention. Meng et al. conducted a study on the impact of white kidney bean NSPs (0.1 g kg−1, 0.2 g kg−1 rats weight) on type 2 diabetes mellitus (T2DM) in rats. They found that kidney bean NSPs can ameliorate hyperglycemia, hyperlipidemia, and liver damage (Fig. 6). This is primarily attributed to the beneficial effects of kidney bean NSPs in promoting insulin production and reducing the blood glucose levels.111 The increased insulin induced by dietary polysaccharides may be captured by the insulin receptor substrate (IRS), inducing the activation of the phosphoinositide 3-kinase (PI3K)/threonine-specific protein kinase (AKT) pathway, followed by the inhibition of glycogen synthase kinase 3 (GSK3), and alleviate diabetes by facilitating gluconeogenesis.112
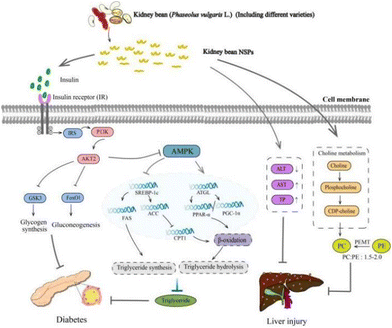 |
| Fig. 6 The anti-diabetic effects of kidney bean NSPs. Kidney bean NSPs can inhibit diabetes by regulating insulin levels. The insulins can be captured by insulin receptors, followed by the activation of PI3K and AKT, and increase in gluconeogenesis. Kidney bean NSPs can also inhibit diabetes by reducing triglyceride through the inhibition of SREBP-1c to lower triglyceride synthesis and the activation of ATGL to increase triglyceride hydrolysis. In addition, NSPs can repair liver injuries by restoring serum ALT, AST, ALB, and TP levels, as well as achieving a balanced PC : PE ratio. | |
The anti-diabetes of kidney bean NSPs may depend on their cultivars. In a study, the anti-diabetic potentials of six plant NSPs, i.e., soybean, white kidney bean, red kidney bean, small black bean, field bean, and lentil, were compared. Among them, the red kidney bean-derived NSPs (0.5 g kg−1 mice weight) showed the greatest anti-diabetic effects in T2DM mice, which significantly reduced fasting blood glucose (FBG), and regulated dyslipidemia by lowering the total cholesterol (TC), total triglyceride (TG), and low-density lipoprotein cholesterol (LDL-c), and also reduced the serum insulin concentrations.20 The difference was explained by the unique fraction with a large molecular weight of 1322 kDa in the red kidney bean NSPs. Furthermore, the unique monosaccharide composition of xylose may also contribute to this anti-diabetes effect. This is different from the observation by Meng et al. that serum insulin was elevated by white kidney bean NSPs, indicating a different regulatory mechanism.111 In general, the reduction in TG levels can be attributed to the increased TG hydrolysis and decreased synthesis followed by the inhibition of the AMPK pathway.113,114 Evidence can be found by the regulatory effects of NSPs on the activation of adipose triglyceride lipase (ATGL) for TG hydrolysis and inhibition of sterol regulatory element binding protein-1c (SREBP-1c) for TG synthesis, which eventually reduced the concentration of triglyceride.115 This regulatory pathway for NSPs to lower TG in diabetic patients needs to be further verified for that from kidney beans.
In addition to anti-diabetes functions, kidney bean NSPs also have a restorative effect on the liver, pancreas, and kidney injuries. Bai et al. investigated the protective effects of red kidney bean NSPs and small black soybean NSPs on hepatic dysfunction in T2DM mice. Both crude NSPs showed protective effects on liver function by restoring serum alanine aminotransferase (ALT), aspartate aminotransferase (AST), albumin (ALB), and total protein (TP).116 Alternatively, the purified red kidney bean NSPs administered at a high dosage of 0.4 g kg−1 rats weight also induced the pathway of glycerophospholipid metabolism in the liver by restoring the ratio of phosphatidylcholine (PC): phosphatidylethanolamine (PE) in T2DM rats. In the liver, PC can be synthesized from choline metabolism or partially produced by PE via the phosphatidylethanolamine N-methyltransferase (PEMT) pathway.117,118 A balanced ratio of PC and PE is the key regulator of mitochondria function, cell membrane integrity, and has been linked with hepatic disorders including non-alcoholic fatty liver disease and non-alcoholic steatohepatitis.119 The observations may suggest a dose dependent manner of kidney bean NSPs.
7. Conclusions
Many varieties of kidney beans have been cultivated worldwide, exhibiting differences in their morphological characteristics including color, shape, and seed size. Thus, a better understanding of the association between varieties and nutrient distribution will guide the application of kidney beans in the food industry and give advice for personalized dietary intervention. To date, research on kidney beans has been focused on proteins, enzyme inhibitors, and polyphenols, while NSPs are less studied. As a major nutritional compound in kidney beans, the beneficial functions of NSPs on regulating the gut microbiota and alleviating obesity and diabetes have been documented. However, many questions about kidney bean NSP extraction, structural identification, and structure/function relationships still remain unanswered. The interactions between NSPs and other bioactive molecules also need to be explored to provide potential design ideas for novel food development on a nutritional basis. Thus, more research is needed to evaluate the biological functions of kidney bean NSPs, and in particular to extend their structure/function relationship, which has not been investigated.
Author contributions
Conceptualization, Chunli Kong, Zheng Yan and Sumei Zhou; formal analysis, Caiping Duan, Yixuan Zhang and YiYing Wang; funding acquisition, Chunli Kong and Zheng Yan; project administration, Zheng Yan and Sumei Zhou; supervision, Chunli Kong and Sumei Zhou; visualization, Chunli Kong, Caiping Duan and Yixuan Zhang; writing – original draft, Chunli Kong and Caiping Duan; writing – review & editing, Chunli Kong, Caiping Duan, Yixuan Zhang, YiYing Wang, Zheng Yan and Sumei Zhou. All authors have read and agreed to the published version of the manuscript.
Conflicts of interest
There are no conflicts to declare.
Acknowledgements
This research was financially supported by the National Natural Science Foundation of China (32201954), National Key Research and Development Program of China (2021YFD1600604), the Program Foundation for Graduate Research Capability Enhancement 2023, the Research Foundation for Youth Scholars of Beijing Technology and Business University (QNJJ2022-12), and Beijing Polytechnic (2020Z005-KXZ).
Notes and references
- J. Aschemann-Witzel, R. F. Gantriis, P. Fraga and F. J. A. Perez-Cueto, Plant-based food and protein trend from a business perspective: markets, consumers, and the challenges and opportunities in the future, Crit. Rev. Food Sci. Nutr., 2021, 61, 3119–3128 CrossRef CAS PubMed.
- F. G. B. Los, A. A. F. Zielinski, J. P. Wojeicchowski, A. Nogueira and I. M. Demiate, Beans (Phaseolus vulgaris L.): whole seeds with complex chemical composition, Curr. Opin. Food Sci., 2018, 19, 63–71 CrossRef.
- FAO, Food and Agriculture Organization of the United Nations, 2021.
- M. Ziarno, D. Zareba, M. Maciejak and A. L. Veber, The impact of dairy starter cultures on selected qualitative properties of functional fermented beverage prepared from germinated white kidney beans, J. Food Nutr. Res., 2019, 58, 167–176 CAS.
- A. Ariani, Y. T. J. C. Berny Mier and P. Gepts, Spatial and Temporal Scales of Range Expansion in Wild Phaseolus vulgaris, Mol. Biol. Evol., 2018, 35, 119–131 CrossRef CAS PubMed.
- I. Wainaina, R. Lugumira, E. Wafula, C. Kyomugasho, D. Sila and M. Hendrickx, Insight into pectin-cation-phytate theory of hardening in common bean varieties with different sensitivities to hard-to-cook, Food Res. Int., 2022, 151, 110862 CrossRef CAS.
- M. T. El-Saadony, M. E. Abd El-Hack, A. A. Swelum, S. I. Al-Sultan, W. R. El-Ghareeb, E. O. S. Hussein, H. A. Ba-Awadh, B. A. Akl and M. M. Nader, Enhancing quality and safety of raw buffalo meat using the bioactive peptides of pea and red kidney bean under refrigeration conditions, Ital. J. Anim. Sci., 2021, 20, 762–776 CrossRef CAS.
- E. S. Neil, J. N. McGinley, V. K. Fitzgerald, C. A. Lauck, J. A. Tabke, M. R. Streeter-McDonald, L. Yao, C. D. Broeckling, T. L. Weir, M. T. Foster and H. J. Thompson, White Kidney Bean (Phaseolus Vulgaris L.) Consumption Reduces Fat Accumulation in a Polygenic Mouse Model of Obesity, Nutrients, 2019, 11, 2780 CrossRef CAS.
- Y. Yang, S. He, Y. Zhang, X. Li, H. Liu, Q. Li, X. Cao, Y. Ye and H. Sun, Comparison of crude prolamins from seven kidney beans (Phaseolus vulgaris L.) based on composition, structure and functionality, Food Chem., 2021, 357, 129748 CrossRef CAS.
- R. Nolan, O. M. Shannon, N. Robinson, A. Joel, D. Houghton and F. C. Malcomson, It’s No Has Bean: A Review of the Effects of White Kidney Bean Extract on Body Composition and Metabolic Health, Nutrients, 2020, 12, 1398 CrossRef.
- M. Tanaka, Y. Honda, S. Miwa, R. Akahori and K. Matsumoto, Comparison of the Effects of Roasted and Boiled Red Kidney Beans (Phaseolus vulgaris L.) on Glucose/Lipid Metabolism and Intestinal Immunity in a High-Fat Diet-Induced Murine Obesity Model, J. Food Sci., 2019, 84, 1180–1187 CrossRef CAS PubMed.
- S. Wang, L. Chen, H. Yang, J. Gu, J. Wang and F. Ren, Regular intake of white kidney beans extract (Phaseolus vulgaris L.) induces weight loss compared to placebo in obese human subjects, Food Sci. Nutr., 2020, 8, 1315–1324 CrossRef CAS.
- Z. Shi, Y. Zhu, C. Teng, Y. Yao, G. Ren and A. Richel, Anti-obesity effects of alpha-amylase inhibitor enriched-extract from white common beans (Phaseolus vulgaris L.) associated with the modulation of gut microbiota composition in high-fat diet-induced obese rats, Food Funct., 2020, 11, 1624–1634 RSC.
- J. Ahmed, N. Al-Ruwaih, M. Mulla and M. H. Rahman, Effect of high pressure treatment on functional, rheological and structural properties of kidney bean protein isolate, LWT, 2018, 91, 191–197 CrossRef CAS.
- L. Ratnawati, D. Desnilasari, D. N. Surahman and R. Kumalasari, Evaluation of Physicochemical, Functional and Pasting Properties of Soybean, Mung Bean and Red Kidney Bean Flour as Ingredient in Biscuit, IOP Conf. Ser.: Earth Environ. Sci., 2019, 251, 012026 Search PubMed.
- A. Nakamura, H. Ohboshi, M. Sakai, K. Nomura, S. Nishiyama and H. Ashida, Extraction of water-soluble polysaccharides from kidney beans and examination of their protein dispersion and stabilization properties under acidic conditions, Food Res. Int., 2021, 144, 110357 CrossRef CAS.
- J. Jayamanohar, P. B. Devi, D. Kavitake, V. B. Priyadarisini and P. H. Shetty, Prebiotic potential of water extractable polysaccharide from red kidney bean (Phaseolus vulgaris L.), LWT, 2019, 101, 703–710 CrossRef CAS.
- Y. Ge, S. Ahmed, W. Yao, L. You, J. Zheng and K. Hileuskaya, Regulation effects of indigestible dietary polysaccharides on intestinal microflora: An overview, J. Food Biochem., 2021, 45, e13564 CrossRef CAS.
- H. Y. Lu, H. W. Zeng, L. Zhang, J. M. Porres and W. H. Cheng, Fecal fermentation products of common bean-derived fiber inhibit C/EBPα and PPARγ expression and lipid accumulation but stimulate PPARδ and UCP2 expression in the adipogenesis of 3T3-L1 cells, J. Nutr. Biochem., 2018, 60, 9–15 CrossRef CAS.
- Z. Bai, J. Meng, X. Huang, G. Wu, S. Zuo and S. Nie, Comparative study on antidiabetic function of six legume crude polysaccharides, Int. J. Biol. Macromol., 2020, 154, 25–30 CrossRef CAS PubMed.
- C. Chavez-Mendoza and E. Sanchez, Bioactive Compounds from Mexican Varieties of the Common Bean (Phaseolus vulgaris): Implications for Health, Molecules, 2017, 22, 1360 CrossRef PubMed.
- N. Larsen, C. Bussolo de Souza, L. Krych, T. Barbosa Cahu, M. Wiese, W. Kot, K. M. Hansen, A. Blennow, K. Venema and L. Jespersen, Potential of Pectins to Beneficially Modulate the Gut Microbiota Depends on Their Structural Properties, Front. Microbiol., 2019, 10, 223 CrossRef PubMed.
- H. Liu, J. Xu, X. Xu, Z. Yuan, H. Song, L. Yang and D. Zhu, Structure/function relationships of bean polysaccharides: A review, Crit. Rev. Food Sci. Nutr., 2023, 63, 330–344 CrossRef PubMed.
- A. P. Pallares, B. A. Miranda, N. Q. A. Truong, C. Kyomugasho, C. M. Chigwedere, M. Hendrickx and T. Grauwet, Process-induced cell wall permeability modulates the in vitro starch digestion kinetics of common bean cotyledon cells, Food Funct., 2018, 9, 6544–6554 RSC.
- L. Zhu, A. Mukherjee, C. Kyomugasho, D. Chen and M. Hendrickx, Calcium transport and phytate hydrolysis during chemical hardening of common bean seeds, Food Res. Int., 2022, 156, 111315 CrossRef CAS PubMed.
- M. M. Grundy, C. H. Edwards, A. R. Mackie, M. J. Gidley, P. J. Butterworth and P. R. Ellis, Re-evaluation of the mechanisms of dietary fibre and implications for macronutrient bioaccessibility, digestion and postprandial metabolism, Br. J. Nutr., 2016, 116, 816–833 CrossRef CAS.
- E. Dickinson, Interfacial structure and stability of food emulsions as affected by protein-polysaccharide interactions, Soft Matter, 2008, 4, 932–942 RSC.
- D. Chen, U. T. T. Pham, A. Van Loey, T. Grauwet, M. Hendrickx and C. Kyomugasho, Microscopic evidence for pectin changes in hard-to-cook development of common beans during storage, Food Res. Int., 2021, 141, 110115 CrossRef CAS.
- P. Semwal, S. Painuli, J. P. S. Begum, A. Jamloki, A. Rauf, A. Olatunde, M. Mominur Rahman, N. Mukerjee, A. Ahmed Khalil, A. S. M. Aljohani, W. Al Abdulmonem and J. Simal-Gandara, Exploring the nutritional and health benefits of pulses from the Indian Himalayan region: A glimpse into the region's rich agricultural heritage, Food Chem., 2023, 422, 136259 CrossRef CAS.
- R. Escutia-Gutierrez, A. Sandoval-Rodriguez, M. Galicia-Moreno, R. Rosas-Campos, M. Almeida-Lopez, A. Santos and J. Armendariz-Borunda, Mexican Ancestral Foods (Theobroma cacao, Opuntia ficus indica,
Persea americana and Phaseolus vulgaris) Supplementation on Anthropometric, Lipid and Glycemic Control Variables in Obese Patients: A Systematic Review and Meta-Analysis, Foods, 2023, 12, 1177 CrossRef CAS.
- M. Przeor, Some Common Medicinal Plants with Antidiabetic Activity, Known and Available in Europe (A Mini-Review), Pharmaceuticals, 2022, 15, 65 CrossRef CAS PubMed.
- J. O. Popoola, O. B. Ojuederie, O. S. Aworunse, A. Adelekan, A. S. Oyelakin, O. L. Oyesola, P. A. Akinduti, S. O. Dahunsi, T. T. Adegboyega, S. U. Oranusi, M. S. Ayilara and C. A. Omonhinmin, Nutritional, functional, and bioactive properties of african underutilized legumes, Front. Plant Sci., 2023, 14, 1105364 CrossRef.
- A. Alfaro-Diaz, A. Escobedo, D. A. Luna-Vital, G. Castillo-Herrera and L. Mojica, Common beans as a source of food ingredients: Techno-functional and biological potential, Compr. Rev. Food Sci. Food Saf., 2023, 22, 2910–2944 CrossRef PubMed.
- A. Karlund, C. Gomez-Gallego, J. Korhonen, O. M. Palo-Oja, H. El-Nezami and M. Kolehmainen, Harnessing Microbes for Sustainable Development: Food Fermentation as a Tool for Improving the Nutritional Quality of Alternative Protein Sources, Nutrients, 2020, 12, 1020 CrossRef CAS.
- I. Wainaina, E. Wafula, D. Sila, C. Kyomugasho, T. Grauwet, A. Van Loey and M. Hendrickx, Thermal treatment of common beans (Phaseolus vulgaris L.): Factors determining cooking time and its consequences for sensory and nutritional quality, Compr. Rev. Food Sci. Food Saf., 2021, 20, 3690–3718 CrossRef.
- Z. Zhang, C. Liu, S. Wu and T. Ma, The Non-Nutritional Factor Types, Mechanisms of Action and Passivation Methods in Food Processing of Kidney Bean (Phaseolus vulgaris L.): A Systematic Review, Foods, 2023, 12, 3697 CrossRef CAS.
- L. Rodriguez, D. Mendez, H. Montecino, B. Carrasco, B. Arevalo, I. Palomo and E. Fuentes, Role of Phaseolus vulgaris L. in the Prevention of Cardiovascular Diseases-Cardioprotective Potential of Bioactive Compounds, Plants, 2022, 11, 186 CrossRef CAS PubMed.
- B. de Fatima Garcia, M. de Barros and T. de Souza Rocha, Bioactive peptides from beans with the potential to decrease the risk of developing noncommunicable chronic diseases, Crit. Rev. Food Sci. Nutr., 2021, 61, 2003–2021 CrossRef CAS.
- S. Peddio, A. Padiglia, F. B. Cannea, R. Crnjar, W. Zam, J. Sharifi-Rad, A. Rescigno and P. Zucca, Common bean (Phaseolus vulgaris L.) alpha-amylase inhibitors as safe nutraceutical strategy against diabetes and obesity: An update review, Phytother. Res., 2022, 36, 2803–2823 CrossRef CAS PubMed.
- C. Cappa, J. D. Kelly and P. K. W. Ng, Seed characteristics and physicochemical properties of powders of 25 edible dry bean varieties, Food Chem., 2018, 253, 305–313 CrossRef CAS PubMed.
- Q.-Q. Yang, R.-Y. Gan, Y.-Y. Ge, D. Zhang and H. Corke, Polyphenols in Common Beans (Phaseolus vulgaris L.): Chemistry, Analysis, and Factors Affecting Composition, Compr. Rev. Food Sci. Food Saf., 2018, 17, 1518–1539 CrossRef CAS.
- S. Sharma, N. Singh, A. S. Virdi and J. C. Rana, Himalayan kidney bean germplasm: Grain-flour characteristics, structural-functional properties and in-vitro digestibility of starches, Food Res. Int., 2015, 77, 498–505 CrossRef CAS.
- L. Kan, S. Nie, J. Hu, Z. Liu and M. Xie, Antioxidant activities and anthocyanins composition of seed coats from twenty-six kidney bean cultivars, J. Funct. Foods, 2016, 26, 622–631 CrossRef CAS.
- N. Parmar, N. Singh, A. Kaur and S. Thakur, Comparison of color, anti-nutritional factors, minerals, phenolic profile and protein digestibility between hard-to-cook and easy-to-cook grains from different kidney bean (Phaseolus vulgaris) accessions, J. Food Sci. Technol., 2017, 54, 1023–1034 CrossRef CAS PubMed.
- D. M. Njoroge, P. K. Kinyanjui, A. O. Makokha, S. Christiaens, A. Shpigelman, D. N. Sila and M. E. Hendrickx, Extraction and characterization of pectic polysaccharides from easy-
and hard-to-cook common beans (Phaseolus vulgaris), Food Res. Int., 2014, 64, 314–322 CrossRef CAS.
- K. A. Adekola, A. Salleh, U. Zaidan, A. Azlan, E. Chiavaro, M. Paciulli and J. M. N. Marikkar, Total Phenolic Content, Antioxidative and Antidiabetic Properties of Coconut (Cocos Nucifera L.) Testa and Selected Bean Seed Coats, Ital. J. Food Sci., 2017, 29, 741–753 CAS.
- K. Shevkani, R. Kaur, N. Singh and D. P. Hlanze, Colour, composition, digestibility, functionality and pasting properties of diverse kidney beans (Phaseolus vulgaris) flours, Curr. Res. Food Sci., 2022, 5, 619–628 CrossRef CAS PubMed.
- D. Guzel and S. Sayar, Effect of cooking methods on selected physicochemical and nutritional properties of barlotto bean, chickpea, faba bean, and white kidney bean, J. Food Sci. Technol., 2012, 49, 89–95 CrossRef PubMed.
- C. Anino, A. N. Onyango, S. Imathiu, J. Maina and F. Onyangore, Chemical composition of the seed and ‘milk’ of three common bean (Phaseolus vulgaris L) varieties, J. Food Meas. Charact., 2019, 13, 1242–1249 CrossRef.
- A. A. Feregrino-Perez, L. C. Berumen, G. Garcia-Alcocer, R. G. Guevara-Gonzalez, M. Ramos-Gomez, R. Reynoso-Camacho, J. A. Acosta-Gallegos and G. Loarca-Pina, Composition and chemopreventive effect of polysaccharides from common beans (Phaseolus vulgaris L.) on azoxymethane-induced colon cancer, J. Agric. Food Chem., 2008, 56, 8737–8744 CrossRef CAS.
- R. R. Kotha, J. W. Finley and D. L. Luthria, Determination of Soluble Mono, Di, and Oligosaccharide Content in 23 Dry Beans (Phaseolus vulgaris L.), J. Agric. Food Chem., 2020, 68, 6412–6419 CrossRef CAS.
- M. Roy, A. Sarker, M. A. K. Azad, M. R. Shaheb and M. M. Hoque, Evaluation of antioxidant and antimicrobial properties of dark red kidney bean (Phaseolus vulgaris) protein hydrolysates, J. Food Meas. Charact., 2019, 14, 303–313 CrossRef.
- S. Punia, S. B. Dhull, K. S. Sandhu, M. Kaur and S. S. Purewal, Kidney bean (Phaseolus vulgaris) starch: A review, Legume Sci., 2020, 2, e52 CrossRef CAS.
- B. Singh, J. P. Singh, K. Shevkani, N. Singh and A. Kaur, Bioactive constituents in pulses and their health benefits, J. Food Sci. Technol., 2017, 54, 858–870 CrossRef CAS.
- L. Kan, S. Nie, J. Hu, S. Wang, S. W. Cui, Y. Li, S. Xu, Y. Wu, J. Wang, Z. Bai and M. Xie, Nutrients, phytochemicals and antioxidant activities of 26 kidney bean cultivars, Food Chem. Toxicol., 2017, 108, 467–477 CrossRef CAS PubMed.
- R. Fatema, J. Rahman, H. B. Shozib, M. I. Nazrul and K. Fatima, Genetic Diversity and Nutritional Components Evaluation of Bangladeshi Germplasms of Kidney Bean (Phaseolus vulgaris L.), J. Genet. Resour., 2019, 5, 83–96 Search PubMed.
- F. A. Mendes, S. T. Leitao, V. Correia, E. Mecha, D. Rubiales, M. R. Bronze and M. C. Vaz Patto, Portuguese Common Bean Natural Variation Helps to Clarify the Genetic Architecture of the Legume’s Nutritional Composition and Protein Quality, Plants, 2021, 11, 26 CrossRef PubMed.
- A. Escobedo, G. Loarca-Pina, M. Gaytan-Martinez, I. Orozco-Avila and L. Mojica, Autoclaving and extrusion improve the functional properties and chemical composition of black bean carbohydrate extracts, J. Food Sci., 2020, 85, 2783–2791 CrossRef CAS.
- J. Slupski and P. Gebczynski, Changes due to cooking and sterilization in low molecular weight carbohydrates in immature seeds of five cultivars of common bean, Int. J. Food Sci. Nutr., 2014, 65, 419–425 CrossRef CAS PubMed.
- N. Siva, P. Thavarajah and D. Thavarajah, Prebiotic carbohydrate concentrations of common bean and chickpea change during cooking, cooling, and reheating, J. Food Sci., 2020, 85, 980–988 CrossRef CAS.
- N. Wang, D. W. Hatcher, R. T. Tyler, R. Toews and E. J. Gawalko, Effect of cooking on the composition of beans (Phaseolus vulgaris L.) and chickpeas (Cicer arietinum L.), Food Res. Int., 2010, 43, 589–594 CrossRef CAS.
- I. A. Wani, D. S. Sogi, A. A. Wani and B. S. Gill, Physical and cooking characteristics of some Indian kidney bean (Phaseolus vulgaris L.) cultivars, J. Saudi Soc. Agric. Sci., 2017, 16, 7–15 Search PubMed.
- B. Carbas, N. Machado, D. Oppolzer, L. Ferreira, M. Queiroz, C. Brites, E. A. Rosa and A. I. Barros, Nutrients, Antinutrients, Phenolic Composition, and Antioxidant Activity of Common Bean Cultivars and their Potential for Food Applications, Antioxidants, 2020, 9, 186 CrossRef CAS PubMed.
- L. Kan, S. Nie, J. Hu, S. Wang, Z. Bai, J. Wang, Y. Zhou, J. Jiang, Q. Zeng and K. Song, Comparative study on the chemical composition, anthocyanins, tocopherols and carotenoids of selected legumes, Food Chem., 2018, 260, 317–326 CrossRef CAS.
- M. Hummel, B. F. Hallahan, G. Brychkova, J. Ramirez-Villegas, V. Guwela, B. Chataika, E. Curley, P. C. McKeown, L. Morrison, E. F. Talsma, S. Beebe, A. Jarvis, R. Chirwa and C. Spillane, Reduction in nutritional quality and growing area suitability of common bean under climate change induced drought stress in Africa, Sci. Rep., 2018, 8, 16187 CrossRef PubMed.
- S. Deb-Choudhury, J. Cooney, D. Brewster, S. Clerens, S. O. Knowles, M. M. Farouk, A. Grosvenor and J. M. Dyer, The effects of blanching on composition and modification of proteins in navy beans (Phaseolus vulgaris), Food Chem., 2021, 346, 128950 CrossRef CAS PubMed.
- J. A. D. Evangelho, N. L. Vanier, V. Z. Pinto, J. J. Berrios, A. R. G. Dias and E. D. R. Zavareze, Black bean (Phaseolus vulgaris L.) protein hydrolysates: Physicochemical and functional properties, Food Chem., 2017, 214, 460–467 CrossRef CAS.
- E. Aquino-Bolaos, Y. GarcaDaz, J. ChavezServia, J. CarrilloRodrguez, A. VeraGuzman and E. HerediaGarcia, Anthocyanins, polyphenols, flavonoids and antioxidant activity in common bean (Phaseolus vulgaris L.) landraces, Emir. J. Food Agric., 2016, 28, 581–588 CrossRef.
- B. Parikh and V. H. Patel, Total phenolic content and total antioxidant capacity of common Indian pulses and split pulses, J. Food Sci. Technol., 2018, 55, 1499–1507 CrossRef CAS PubMed.
- E. Mecha, S. T. Leitao, B. Carbas, A. T. Serra, P. M. Moreira, M. M. Veloso, R. Gomes, M. E. Figueira, C. Brites, M. C. Vaz Patto and M. R. Bronze, Characterization of Soaking Process’ Impact in Common Beans Phenolic Composition: Contribute from the Unexplored Portuguese Germplasm, Foods, 2019, 8, 296 CrossRef CAS.
- H. Orak, M. Karamać, A. Orak and R. Amarowicz, Antioxidant Potential and Phenolic Compounds of Some Widely Consumed Turkish White Bean (Phaseolus vulgaris L.) Varieties, Pol. J. Food Nutr. Sci., 2016, 66, 253–260 CrossRef CAS.
- R. R. Madrera, A. C. Negrillo, B. S. Valles and J. J. F. Fernandez, Phenolic Content and Antioxidant Activity in Seeds of Common Bean (Phaseolus vulgaris L.), Foods, 2021, 10, 864 CrossRef CAS.
- D. Hernández-Saavedra, M. Mendoza-Sanchez, H. L. Hernandez-Montiel, H. S. Guzman-Maldonado, G. F. Loarca-Pina, L. M. Salgado and R. Reynoso-Camacho, Cooked common beans (Phaseolus vulgaris) protect against beta-cell damage in streptozotocin-induced diabetic rats, Plant Foods Hum. Nutr., 2013, 68, 207–212 CrossRef.
- C. I. Teixeira-Guedes, D. Oppolzer, A. I. Barros and C. Pereira-Wilson, Impact of cooking method on phenolic composition and antioxidant potential of four varieties of Phaseolus vulgaris L. and Glycine max L, LWT, 2019, 103, 238–246 CrossRef CAS.
- Y. D. García-Díaz, E. N. Aquino-Bolaños, J. L. Chávez-Servia, A. M. Vera-Guzmán and J. C. Carrillo-Rodríguez, Bioactive compounds and antioxidant activity in the common bean are influenced by cropping season and genotype, Chil. J. Agric. Res., 2018, 78, 255–265 CrossRef.
- J. Zhang, F. Li, S. Shen, Z. Yang, X. Ji, X. Wang, X. Liao and Y. Zhang, More simple, efficient and accurate food research promoted by intermolecular interaction approaches: A review, Food Chem., 2023, 416, 135726 CrossRef CAS PubMed.
- A. P. Pallares, B. Loosveldt, S. N. Karimi, M. Hendrickx and T. Grauwet, Effect of process-induced common bean hardness on structural properties of in vivo generated boluses and consequences for in vitro starch digestion kinetics, Br. J. Nutr., 2019, 122, 388–399 CrossRef.
- Y. Wu, Q. Du, X. Fan, C. Zhou, J. He, Y. Sun, Q. Xia and D. Pan, Interaction between Kidney-Bean Polysaccharides and Duck Myofibrillar Protein as Affected by Ultrasonication: Effects on Gel Properties and Structure, Foods, 2022, 11, 3998 CrossRef CAS PubMed.
- A. Sieminska-Kuczer, M. Szymanska-Chargot and A. Zdunek, Recent advances in interactions between polyphenols and plant cell wall polysaccharides as studied using an adsorption technique, Food Chem., 2022, 373, 131487 CrossRef CAS PubMed.
- M. Dueñas, T. Sarmento, Y. Aguilera, V. Benitez, E. Mollá, R. M. Esteban and M. A. Martín-Cabrejas, Impact of cooking and germination on phenolic composition and dietary fibre fractions in dark beans (Phaseolus vulgaris L.) and lentils (Lens culinaris L.), LWT – Food Sci. Technol., 2016, 66, 72–78 CrossRef.
- P. A. R. Fernandes, C. Le Bourvellec, C. Renard, D. F. Wessel, S. M. Cardoso and M. A. Coimbra, Interactions of arabinan-rich pectic polysaccharides with polyphenols, Carbohydr. Polym., 2020, 230, 115644 CrossRef CAS PubMed.
- L. Jakobek, Interactions of polyphenols with carbohydrates, lipids and proteins, Food Chem., 2015, 175, 556–567 CrossRef CAS PubMed.
- L. M. Perez-Hernandez, K. Nugraheni, M. Benohoud, W. Sun, A. J. Hernandez-Alvarez, M. R. A. Morgan, C. Boesch and C. Orfila, Starch Digestion Enhances Bioaccessibility of Anti-Inflammatory Polyphenols from Borlotti Beans (Phaseolus vulgaris), Nutrients, 2020, 12, 295 CrossRef CAS PubMed.
- D. Perera, L. Devkota, G. Garnier, J. Panozzo and S. Dhital, Hard-to-cook phenomenon in common legumes: Chemistry, mechanisms and utilisation, Food Chem., 2023, 415, 135743 CrossRef CAS.
- T. Koriyama, Y. Sato, K. Iijima and M. Kasai, Influences of Soaking Temperature and Storage Conditions on Hardening of Soybeans (Glycine max) and Red Kidney Beans (Phaseolus vulgaris), J. Food Sci., 2017, 82, 1546–1556 CrossRef CAS PubMed.
- S. Rousseau, A. Pallares Pallares, F. Vancoillie, M. Hendrickx and T. Grauwet, Pectin and phytic acid reduce mineral bioaccessibility in cooked common bean cotyledons regardless of cell wall integrity, Food Res. Int., 2020, 137, 109685 CrossRef CAS PubMed.
- J. Yi, D. M. Njoroge, D. N. Sila, P. K. Kinyanjui, S. Christiaens, J. Bi and M. E. Hendrickx, Detailed analysis of seed coat and cotyledon reveals molecular understanding of the hard-to-cook defect of common beans (Phaseolus vulgaris L.), Food Chem., 2016, 210, 481–490 CrossRef CAS PubMed.
- R. Campos-Vega, R. Reynoso-Camacho, G. Pedraza-Aboytes, J. A. Acosta-Gallegos, S. H. Guzman-Maldonado, O. Paredes-Lopez, B. D. Oomah and G. Loarca-Pina, Chemical composition and in vitro polysaccharide fermentation of different beans (Phaseolus vulgaris L.), J. Food Sci., 2009, 74, T59–T65 CrossRef CAS PubMed.
- P. Patra, D. Das, B. Behera, T. K. Maiti and S. S. Islam, Structure elucidation of an immunoenhancing pectic polysaccharide isolated from aqueous extract of pods of green bean (Phaseolus vulgaris L.), Carbohydr. Polym., 2012, 87, 2169–2175 CrossRef CAS.
- F. Kamarudin and C. Y. Gan, Molecular structure, chemical properties and biological activities of Pinto bean pod polysaccharide, Int. J. Biol. Macromol., 2016, 88, 280–287 CrossRef CAS PubMed.
- W. Tang, A. Q. Li, J. Y. Yin and S. P. Nie, Structural characteristics of three pectins isolated from white kidney bean, Int. J. Biol. Macromol., 2021, 182, 2151–2161 CrossRef CAS PubMed.
- G. Mao, D. Wu, C. Wei, W. Tao, X. Ye, R. J. Linhardt, C. Orfila and S. Chen, Reconsidering conventional and innovative methods for pectin extraction from fruit and vegetable waste: Targeting rhamnogalacturonan I, Trends Food Sci. Technol., 2019, 94, 65–78 CrossRef CAS.
- J. Huang, Z. Hu, L. Hu, G. Li, Q. Yao and Y. Hu, Pectin-based active packaging: A critical review on preparation, physical properties and novel application in food preservation, Trends Food Sci. Technol., 2021, 118, 167–178 CrossRef CAS.
- S. Xu, J. A. Lane, J. Chen, Y. Zheng, H. Wang, X. Fu, Q. Huang, S. Dhital, F. Liu and B. Zhang, In Vitro Infant Fecal Fermentation Characteristics of Human Milk Oligosaccharides Were Controlled by Initial Microbiota Composition More than Chemical Structure, Mol. Nutr. Food Res., 2022, 66, e2200098 CrossRef PubMed.
- Q. Q. Yang, R. Y. Gan, Y. Y. Ge, D. Zhang and H. Corke, Polyphenols in Common Beans (Phaseolus vulgaris L.): Chemistry, Analysis, and Factors Affecting Composition, Compr. Rev. Food Sci. Food Saf., 2018, 17, 1518–1539 CrossRef CAS PubMed.
- D. Chen, K. Hu, L. Zhu, M. Hendrickx and C. Kyomugasho, Cell wall polysaccharide changes and involvement of phenolic compounds in ageing of Red haricot beans (Phaseolus vulgaris) during postharvest storage, Food Res. Int., 2022, 162, 112021 CrossRef CAS PubMed.
- Y. Zhu, J. Li, X. Feng, Z. Shi, Y. Yao and R. Shen, Structural characterisation of two polysaccharides from white common bean (Phaseolus vulgaris L.) and the application in microencapsulation of probiotics, Int. J. Food Sci. Technol., 2022, 57, 7184–7193 CrossRef CAS.
- C. M. Chigwedere, C. M. Nkonkola, S. Rai, C. Kyomugasho, Z. J. Kermani, A. Pallares Pallares, A. M. Van Loey, T. Grauwet and M. E. Hendrickx, Cotyledon pectin molecular interconversions explain pectin solubilization during cooking of common beans (Phaseolus vulgaris), Food Res. Int., 2019, 116, 462–470 CrossRef CAS.
- T. M. Shiga and F. M. Lajolo, Cell wall polysaccharides of common beans (Phaseolus vulgaris L.)—composition and structure, Carbohydr. Polym., 2006, 63, 1–12 CrossRef CAS.
- J. Xie, Q. Song, Q. Yu, Y. Chen, Y. Hong and M. Shen, Dietary polysaccharide from Mung bean [Vigna radiate (Linn.) Wilczek] skin modulates gut microbiota and short-chain fatty acids in mice, Int. J. Food Sci. Technol., 2021, 57, 2581–2589 CrossRef.
- J. Liu, M. E. Hefni, C. M. Witthoft, M. Bergstrom, S. Burleigh, M. Nyman and F. Hallenius, Effects of Whole Brown Bean and Its Isolated Fiber Fraction on Plasma Lipid Profile, Atherosclerosis, Gut Microbiota, and Microbiota-Dependent Metabolites in Apoe(−/−) Mice, Nutrients, 2022, 14, 937 CrossRef CAS PubMed.
- Z. Zdunczyk, J. Jankowski, A. Rutkowski, E. Sosnowska, A. Drazbo, P. Zdunczyk and J. Juskiewicz, The composition and enzymatic activity of gut microbiota in laying hens fed diets supplemented with blue lupine seeds, Anim. Feed Sci. Technol., 2014, 191, 57–66 CrossRef CAS.
- A. M. Rovalino-Córdova, V. Fogliano and E. Capuano, Effect of bean structure on microbiota utilization of plant nutrients: An in-vitro study using the simulator of the human intestinal microbial ecosystem (SHIME®), J. Funct. Foods, 2020, 73, 104087 CrossRef.
- D. Wu, J. Wan, W. Li, J. Li, W. Guo, X. Zheng, R. Y. Gan, Y. Hu and L. Zou, Comparison of Soluble Dietary Fibers Extracted from Ten Traditional Legumes: Physicochemical Properties and Biological Functions, Foods, 2023, 12, 2352 CrossRef CAS PubMed.
- R. Campos-Vega, R. G. Guevara-Gonzalez, B. L. Guevara-Olvera, B. Dave Oomah and G. Loarca-Piña, Bean (Phaseolus vulgaris L.) polysaccharides modulate gene expression in human colon cancer cells (HT-29), Food Res. Int., 2010, 43, 1057–1064 CrossRef CAS.
- R. K. Cruz-Bravo, R. G. Guevara-Gonzalez, M. Ramos-Gomez, B. D. Oomah, P. Wiersma, R. Campos-Vega and G. Loarca-Pina, The fermented non-digestible fraction of common bean (Phaseolus vulgaris L.) triggers cell cycle arrest and apoptosis in human colon adenocarcinoma cells, Genes Nutr., 2014, 9, 359 CrossRef CAS PubMed.
- Z. Shi, X. Zhang, Y. Zhu, Y. Yao and G. Ren, Natural Extracts from White Common Bean (Phaseolus vulgaris L.) Inhibit 3T3-L1 Adipocytes Differentiation, Appl. Sci., 2021, 11, 167 CrossRef CAS.
- F. Magne, M. Gotteland, L. Gauthier, A. Zazueta, S. Pesoa, P. Navarrete and R. Balamurugan, The Firmicutes/Bacteroidetes Ratio: A Relevant Marker of Gut Dysbiosis in Obese Patients?, Nutrients, 2020, 12, 1474 CrossRef CAS PubMed.
- E. J. Reverri, J. M. Randolph, C. T. Kappagoda, E. Park, I. Edirisinghe and B. M. Burton-Freeman, Assessing beans as a source of intrinsic fiber on satiety in men and women with metabolic syndrome, Appetite, 2017, 118, 75–81 CrossRef PubMed.
- E. S. Chambers, D. J. Morrison and G. Frost, Control of appetite and energy intake by SCFA: what are the potential underlying mechanisms?, Proc. Nutr. Soc., 2015, 74, 328–336 CrossRef CAS PubMed.
- J. Meng, Z. Bai, W. Huang, Y. Liu, P. Wang, S. Nie and X. Huang, Polysaccharide from white kidney bean can improve hyperglycemia and hyperlipidemia in diabetic rats, Bioact. Carbohydr. Diet. Fibre, 2020, 24, 100222 CrossRef CAS.
- K. Ganesan and B. Xu, Anti-Diabetic Effects and Mechanisms of Dietary Polysaccharides, Molecules, 2019, 24, 2556 CrossRef CAS PubMed.
- S. Kovacic, C. L. Soltys, A. J. Barr, I. Shiojima, K. Walsh and J. R. Dyck, Akt activity negatively regulates phosphorylation of AMP-activated protein kinase in the heart, J. Biol. Chem., 2003, 278, 39422–39427 CrossRef CAS.
- L. Jia, W. Li, J. Li, Y. Li, H. Song, Y. Luan, H. Qi, L. Ma, X. Lu and Y. Yang, Lycium barbarum polysaccharide attenuates high-fat diet-induced hepatic steatosis by up-regulating SIRT1 expression and deacetylase activity, Sci. Rep., 2016, 6, 36209 CrossRef CAS.
- Q. Wu, Q. Wang, J. Fu and R. Ren, Polysaccharides derived from natural sources regulate triglyceride and cholesterol metabolism: a review of the mechanisms, Food Funct., 2019, 10, 2330–2339 RSC.
- Z. Bai, X. Huang, G. Wu, Y. Zhou, X. Deng, J. Yang, J. Yin and S. Nie, Hepatic metabolism-related effects of polysaccharides from red kidney bean and small black soybean on type 2 diabetes, Food Chem., 2023, 403, 134334 CrossRef CAS PubMed.
- D. Liu, J. Wang, H. Zeng, F. Zhou, B. Wen, X. Zhang, Y. Luo, W. Wu, J. Huang and Z. Liu, The metabolic regulation of Fuzhuan brick tea in high-fat diet-induced obese mice and the potential contribution of gut microbiota, Food Funct., 2022, 13, 356–374 RSC.
- T. Wu, X. Zheng, M. Yang, A. Zhao, M. Li, T. Chen, J. Panee, W. Jia and G. Ji, Serum lipid alterations identified in chronic hepatitis B, hepatitis B virus-associated cirrhosis and carcinoma patients, Sci. Rep., 2017, 7, 42710 CrossRef CAS PubMed.
- J. N. van der Veen, J. P. Kennelly, S. Wan, J. E. Vance, D. E. Vance and R. L. Jacobs, The critical role of phosphatidylcholine and phosphatidylethanolamine metabolism in health and disease, Biochim. Biophys. Acta, Biomembr., 2017, 1859, 1558–1572 CrossRef CAS PubMed.
|
This journal is © The Royal Society of Chemistry 2024 |