DOI:
10.1039/D3SC04268E
(Edge Article)
Chem. Sci., 2023,
14, 12034-12040
Nitrenium ions as new versatile reagents for electrophilic amination†
Received
15th August 2023
, Accepted 25th September 2023
First published on 30th September 2023
Abstract
Herein we report the utilization of N-heterocyclic nitrenium ions – easily prepared, bench-stable and non-oxidating nitrogen sources for the efficient electrophilic amination of aliphatic and aromatic organometallic nucleophiles, towards the facile and general preparation of primary amines. To this end, a plethora of abundant organolithium and organomagnesium reagents were combined with nitrenium salts to generate a variety of previously unexplored N-alkyl and N-aryl triazanes. Through the simple hydrogenolysis of these relatively stable triazanes, we have prepared a diverse scope of primary amines, including linear and branched aliphatic as well as (hetero)aromatic amines possessing various stereo-electronic substituents. Furthermore, we present the facile synthesis of valuable 15N-labelled primary amines from easily prepared 15N-labelled nitrenium salts, as well as a one-pot approach to biologically relevant primary amines. Finally, a recyclable variant of the nitrenium precursor was prepared and a simple recovery protocol was developed to improve the atom-economy of this procedure.
Introduction
Amines in general, and primary amines in particular, play a crucial role in chemical enterprise due to a plethora of valuable applications. They are found in a wide range of natural products and synthetic materials, and have diverse employment in pharmaceuticals, agrochemicals, polymers, and materials science.1 The unique chemical properties of organic amines, such as their ability to form relatively strong hydrogen bonds and to act as nucleophiles in chemical reactions, make them essential building blocks in many chemical processes. Therefore, development of new selective and versatile methods for the synthesis of primary amines represents a highly important yet challenging goal.
Representative classical methods include alkylation of ammonia with alkyl halides, reduction of nitriles, the Gabriel synthesis, and reductive amination.2 All these nucleophilic amination approaches are restricted to aliphatic amines and have their own well-known limitations, e.g., overalkylation and/or limitation to sterically non-congested alkyl halides. Aromatic primary amines can be prepared, for example, via reduction of nitroarenes.3 Modern methods encompass the Buchwald–Hartwig transition metal catalyzed amination4,5 of aryl electrophiles with ammonia-equivalent reagents.6,7 This highly useful reaction usually employs an expensive ligand–metal catalyst and is challenging for the amination of sterically congested organic skeletons.8
An attractive alternative approach to the synthesis of amines is electrophilic amination, in which organometallic nucleophilic precursors undergo C–N bond formation via their reaction with electrophilic N-species (Scheme 1a). This approach has attracted the continuous interest of chemists, thus several types of electrophilic aminating reagents have been developed, such as haloamines, O-substituted hydroxylamines, oxaziridines, oximes, organic azides and diazonium salts (Scheme 1b).9–15 Nonetheless, many of these reagents are only moderately stable, possess oxidizing properties, require tedious preparation protocols or are specific for certain types of nucleophiles.
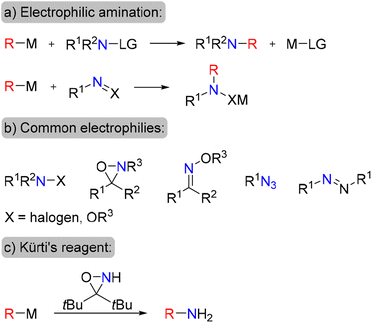 |
| Scheme 1 Electrophilic amination reactions and reagents. | |
Moreover, only a few of the available electrophilic amination reagents are suitable for the preparation of primary amines.16,17 Recently, the electrophilic amination approach has received a boost due to an elegant design of specific bulky oxaziridines for the amination of organic nucleophiles18,19 (Scheme 1c) as well as development of transition-metal-catalyzed amination procedures.20–22 Undoubtedly, development of a new robust and stable electrophilic aminating agent, applicable for the synthesis of both aliphatic and aromatic amines is of high value.
We have recently established a program on the chemistry of N-heterocyclic nitrenium ions (NHNs). These ions are N-based isostructural and isoelectronic analogs of the ubiquitous N-heterocyclic carbenes (Scheme 2a).23 We and others demonstrated that such species can serve as cationic ligands to a plethora of transition metals, being poor σ-donors and considerable π-acceptors.24–28 This is due to a substantial contribution of the resonance form A to the electronic structure of the nitrenium ions. This form demonstrates a nitrogen non-octet character in these ions, which possess a relatively low LUMO and a free p-orbital on the central N-atom. These unique properties of NHNs allowed us and others to demonstrate that NHNs can act as nitrogen-based Lewis acids,29–33 activators of strong bonds through frustrated Lewis pair chemistry,30,34 catalytic Lewis acids for several reactions,35–38 photocatalysts39–41 and a platform for stable nitrogen-based radicals.42,43
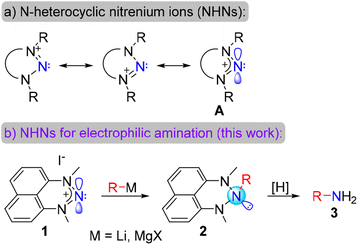 |
| Scheme 2 Structure of NHNs and their use in amination. | |
Exploring this Lewis acidity, we previously demonstrated that NHN 1 reacts with phenyllithium or n-butyllithium to furnish cyclic triazanes 2 (Scheme 2b).29 While such triazanes possess an unusual molecular structure bearing three saturated nitrogen atoms in row, their intriguing chemistry is previously unexplored. We envision that the N–N bonds in these compounds should be relatively weak and could be cleaved under standard reduction conditions, providing primary amines via N-atom deletion from the triazane ring (Scheme 2b). Interestingly, carbon and nitrogen atom deletion from heterocycles for synthetic purposes,44–47 including carbon atom deletion from N-heterocyclic carbenes,48 have gained much current interest. If this hypothesis is successful, the NHN would serve as a novel robust and stable electrophilic aminating agent. Moreover, the first step of the nucleophile addition would furnish a protected primary amine. This form is essentially stable (vide infra) and can be tolerated during the synthesis.
In this article we report on the preparation and full characterization of a broad range of N-alkyl and N-aryl triazanes, which properties, to the best of our knowledge, were not previously explored. Most importantly, we demonstrate that the nitrenium salt – an air-stable, non-oxidative and easily prepared compound – can serve as a novel efficient electrophilic aminating agent. Utilizing nitrenium, we have prepared a broad range of primary amines. This approach is truly general: linear and branched aliphatic amines as well as aromatic amines bearing substituents with variable stereo-electronic parameters on the aromatic ring can be efficiently prepared by this reaction. Moreover, 15N-labeled amines can be easily prepared by our procedure since we provide a facile approach to the 15N-labeled bench-stable nitrenium salts. We also reveal a straightforward protocol for the recycling of the generated co-product diaminonaphthalene to the starting aminating nitrenium, to address the atom-economy of the method.
Results and discussion
Exploring organometallic nucleophiles in reaction with NHN 1, we were pleased to observe that a broad scope of both organolithium and organomagnesium reagents selectively react with the nitrenium cation to provide the corresponding triazanes 2 (Scheme 3). Moreover, the generality of this reaction is substantial since the reaction was accomplished using numerous alkyl and aryl nucleophiles possessing various stereo-electronic characteristics. We were able to produce triazanes comprising primary (2a–c), secondary (2d–f), and tertiary (2g–h) alkyl groups bonded to the central N-atom, including cyclic alkyl motifs (2f, 2h). Furthermore, triazanes resulting from the combination of 1 with variously substituted aryl-M (M = Li or MgX), including electron-donating and electron-withdrawing groups in the para-, meta- and ortho-positions (2i–w), were successfully generated. Most of these triazanes were sufficiently stable at room temperature to be isolated and characterized by multinuclear NMR.
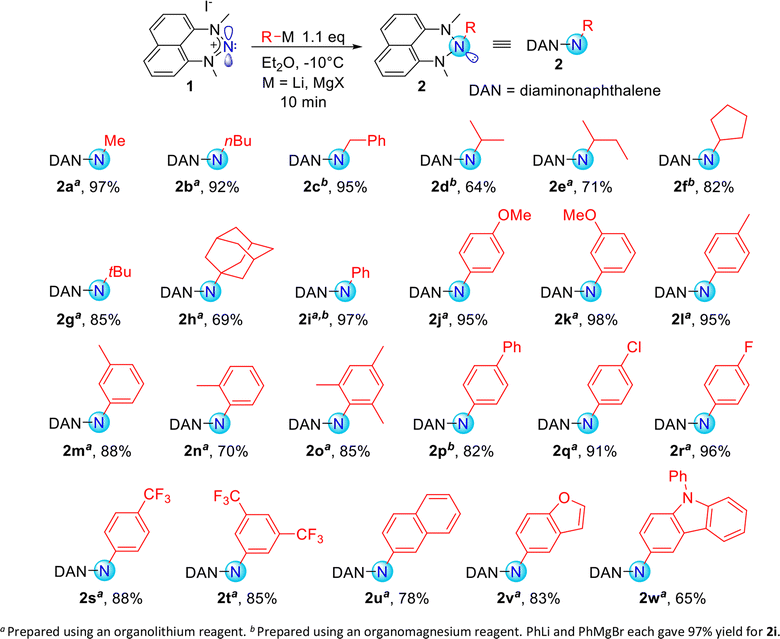 |
| Scheme 3 Scope of triazanes; reaction scale: 0.3 mmol (0.08 M); yields are of isolated products. | |
The molecular structures of these triazanes were confirmed, for the first time, by single-crystal X-ray crystallography. Some representative structures (2a, 2d, 2i, 2p) are shown in Fig. 1. Contrary to the planar nitrenium species, the central N-atoms in all the triazanes are located outside the plane of the naphthalene moiety. This occurs since the central nitrogen atoms undergo pyramidalization as they transform from the sp2 hybridization in the nitrenium precursor to the sp3 hybridization in the triazane product, upon occupation of the vacant pπ orbital on the central N-atom by the σ-donation from the corresponding nucleophile.
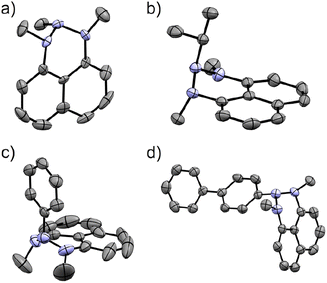 |
| Fig. 1 POV-ray depictions of the X-ray structures of selected triazanes. (a) 2a; (b) 2d; (c) 2i; (d) 2p. Anisotropic displacement ellipsoids are set at 50% probability. H atoms are omitted for clarity. | |
Having a wide range of triazanes in hand, we envisioned that the formal reduction of these structures would lead to the N–N bonds cleavage and deletion of the central nitrogen atom from the triazane ring, generating the desired primary amine (Table 1). To investigate this hypothesis, we subjected triazane 2i to 1 bar of H2 at 40 °C, in the presence of 10 mol% of Pd/C catalyst, utilizing several different solvents (at room temperature, the reaction was slower – Table 1, entry 1).
Table 1 Optimization of triazane hydrogenolysis
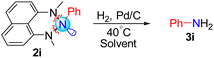
|
Entry |
Solvent |
Time (h) |
Yielda (%) |
Measured by 1H NMR.
Performed at 25 °C.
2 bar of H2 used.
|
1 |
Toluene-d8b |
6 |
100 |
2 |
Toluene-d8 |
2 |
100 |
3 |
Toluene-d8 |
1 |
92 |
4 |
Methanol-d4 |
2 |
36 |
5 |
Methanol-d4c |
2 |
100 |
6 |
Benzene-d6 |
2 |
100 |
7 |
Acetonitrile-d3 |
2 |
50 |
8 |
Chloroform-d |
2 |
25 |
9 |
DCM-d2 |
2 |
100 |
10 |
DMSO-d6 |
2 |
45 |
Gratifyingly, many of the examined solvents were suitable for this hydrogenolysis reaction, generating the desired aniline in quantitative yield (Table 1). Applying these conditions for all the triazanes we synthesized, using DCM as the solvent (CD2Cl2 was used for amines 2a-b, 2d–g), generated the corresponding primary amines in good to excellent yields (Scheme 4).
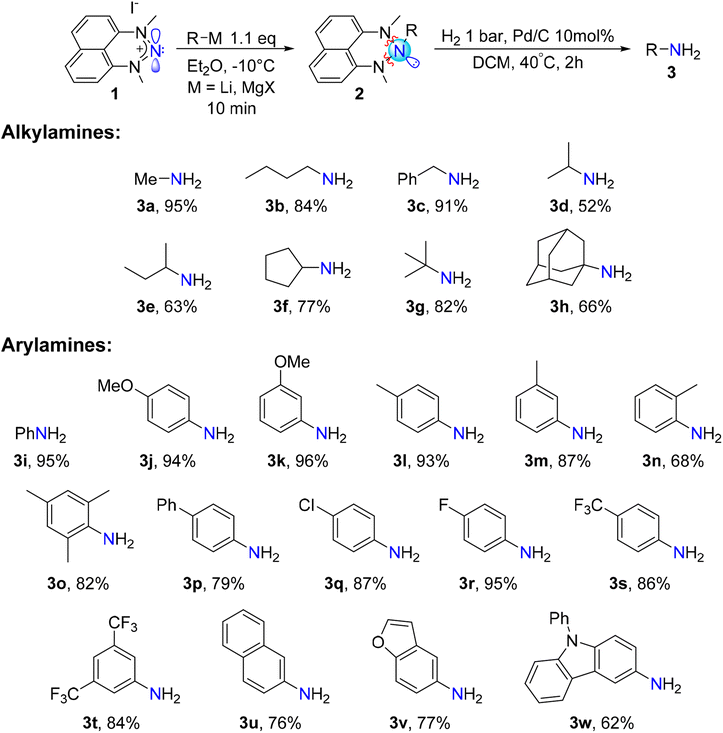 |
| Scheme 4 Scope of amines produced by the hydrogenolysis of triazanes. Reaction scale: 0.3 mmol (0.08 M); for 3i, the reaction was scaled up to 0.2 g of 1 (92% yield). Yields are from 1 to 3; yields of 2a-b, 2d–g were calculated by 1HNMR; all other yields are for the isolated product. | |
As can be seen on Scheme 4, primary amines featuring linear and branched alkyl skeletons were successfully synthesized. This includes the installment of the NH2 unit on primary (3a–c), secondary (3d–f) and tertiary (3g-h) alkyl groups. Examples of primary amines incorporating cyclic (3f) and polycyclic (3h) alkyl groups were also attained. In addition, our electrophilic amination method proved suitable for the synthesis of primary arylamines featuring electron-withdrawing and electron-donating groups on the rings. This includes various substituents at the para-, meta-, and ortho positions of the aromatic ring. Even a bulky arylamine bearing methyl substituents at two ortho positions (3o) can be prepared with an isolated yield of 82%. Such sterically congested arylamines are challenging to prepare by the Buchwald–Hartwig cross-coupling reaction and require specific conditions.8 We were also able to synthesize primary amines comprising heterocyclic aryl groups such as benzofuran (3v) and 9-phenylcarbazole (3w).
Markedly, 15N-labelled primary amines are easily accessible via our protocol since the 15N-labelled NHN precursor 1′ is selectively and facilely prepared using the commercially available, isotopically labelled Na15NO2.29 As an example, we have prepared aniline-15N 3i′ with a yield as high as 95%. Additionally, the dyskinesia medication amantadine (1-adamantylamine, 3h)49 was prepared with 66% isolated yield. These examples demonstrate the potential of this procedure for the synthesis of valuable isotopically labelled and biologically active compounds.
To prepare amines utilizing our approach, it is not necessary to isolate the R-N-triazane intermediate. The whole procedure, including the preparation of R-Li, its amination with a nitrenium salt and the final “deprotection” to the desired primary amine can be done in a one-pot process. As an interesting example, we generated in situ an enolate of ester 4 by its treatment with lithium diisopropylamide (LDA) at −78 °C and quenched it with the aminating agent 1 (Scheme 5a). The resulting triazane 2x was directly, without isolation, treated with hydrogen gas in the presence of a catalytic amount of Pd/C to furnish the amino acid precursor phenylalanine ethyl ester 3x. This further demonstrates the capability of our electrophilic amination procedure to produce compounds of well-established biological importance in a one-pot protocol.
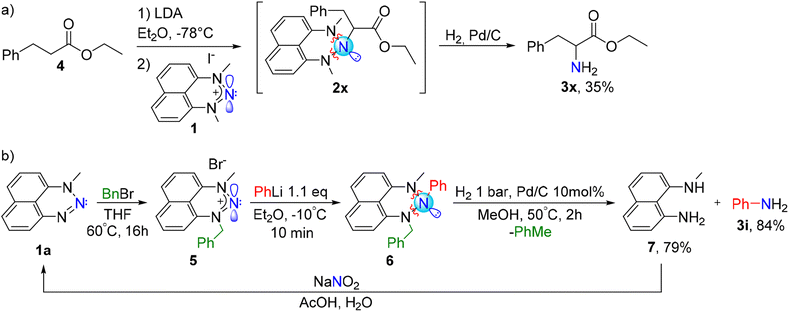 |
| Scheme 5 (a) A one-pot procedure for the synthesis of phenylalanine ethyl ester 3x (isolated yield); (b) a recyclable variant for improving the atom-economy of the NHN-based electrophilic amination. Yields are for the isolated products, from NHN 5 to products 3i + 7. | |
Notably, we have developed this method driven by our fundamental interest in the properties of novel triazanes. NHN 1 can serve not only as a versatile aminating agent, but also, in the triazane form 2, as a protecting group for primary amines (a masked RNH2), which can be deprotected at the late stage of the synthesis. However, we recognize that potential scale-up protocols would be concerned by the formation of the diaminonaphthalene co-product. To improve the atom-economy of this procedure, we devised a method enabling the recycling of the residual diaminonaphthalene. To this end, we modified the NHN precursor by replacing one of the flanking methyl groups with a benzyl group, by reacting N-methyl-naphthotriazine 1a with benzyl bromide (Scheme 5b). We combined the newly produced NHN 5 with phenyllithium, using the same conditions as with the precursor 1, and generated the corresponding triazane 6. Gratifyingly, the subsequent hydrogenolysis of 6 (using 2 bar of H2 and 10 mol% of Pd/C, in methanol at 50 °C, over 4 hours) directly results in a clean formation of the desired amine 3i along with the N-methyl-1,8-diaminonaphthalene 7. Notably, diamine 7 can be utilized as a starting material for the preparation of NHN 5; therefore, it can be recycled by a reaction with NaNO2 – our standard approach to the synthesis of nitrenium substrates – to regenerate triazine 1a.
Conclusions
In summary, we demonstrated a new efficient and selective method for the synthesis of primary amines via electrophilic amination, utilizing easily prepared, non-oxidative and bench-stable nitrenium salts as the electrophilic nitrogen source. This robust and general procedure makes use of abundant organolithium and organomagnesium reagents as the nucleophilic partners. The method is suitable for the preparation of linear and branched aliphatic and aromatic amines with various stereo-electronic properties. Biologically relevant and 15N-labelled counterparts were easily prepared, displaying the capability and viability of this procedure to obtain these valuable compounds. Moreover, we also produced a recyclable variant of the nitrenium precursor to increase the atom economy of our method. We demonstrated that the N-R-triazanes, whose crystallographic characterization and properties were revealed here for the first time, represent isolable intermediates in the amination process. Other nucleophiles (organozinc, organoboron, etc.) for electrophilic amination using nitrenium salts are under investigation in our labs.
Data availability
Further experimental details, synthetic procedures, characterization data, copies of NMR spectra and X-ray crystallographic data are available in the ESI.†
Author contributions
I. A. and K. S. preformed the experiments and the NMR characterization. N. F. performed the X-ray crystallography characterization. I. A. and M. G. co-wrote the manuscript with feedback from K. S. and N. F. M. G. conceived the idea and directed the project.
Conflicts of interest
There are no conflicts to declare.
Acknowledgements
We are thankful for the Israel Science Foundation (ISF personal grant 1449/19 and ISF-NSFC grant 3673/21) for financial support.
Notes and references
-
S. A. Lawrence, Amines: Synthesis, Properties and Applications, Cambridge University Press, Cambridge, U.K., 2004 Search PubMed.
-
A. Ricci and L. Bernardi, Methodologies in Amine Synthesis: Challenges and Applications, Wiley-VCH Verlag GmbH & Co. KGaA, Weinheim, Germany, 2021 Search PubMed.
- M. Orlandi, D. Brenna, R. Harms, S. Jost and M. Benaglia, Recent Developments in the Reduction of Aromatic and Aliphatic Nitro Compounds to Amines, Org. Process Res. Dev., 2018, 22, 430–445 CrossRef CAS.
- M. M. Heravi, Z. Kheilkordi, V. Zadsirjan, M. Heydari and M. Malmir, Buchwald–Hartwig reaction: An overview, J. Organomet. Chem., 2018, 861, 17–104 CrossRef CAS.
- R. Dorel, C. P. Grugel and A. M. Haydl, The Buchwald–Hartwig Amination After 25 Years, Angew. Chem., Int. Ed., 2019, 58, 17118–17129 CrossRef CAS PubMed.
- J. P. Wolfe, J. Åhman, J. P. Sadighi, R. A. Singer and S. L. Buchwald, An Ammonia Equivalent for the Palladium-Catalyzed Amination of Aryl Halides and Triflates, Tetrahedron Lett., 1997, 38, 6367–6370 CrossRef CAS.
- S. Lee, M. Jørgensen and J. F. Hartwig, Palladium-Catalyzed Synthesis of Arylamines from Aryl Halides and Lithium Bis(trimethylsilyl)amide as an Ammonia Equivalent, Org. Lett., 2001, 3, 2729–2732 CrossRef CAS PubMed.
- S. M. Raders, J. N. Moore, J. K. Parks, A. D. Miller, T. M. Leißing, S. P. Kelley, R. D. Rogers and K. H. Shaughnessy, Trineopentylphosphine: A Conformationally Flexible Ligand for the Coupling of Sterically Demanding Substrates in the Buchwald–Hartwig Amination and Suzuki–Miyaura Reaction, J. Org. Chem., 2013, 78, 4649–4664 CrossRef CAS PubMed.
- M. Corpet and C. Gosmini, Recent Advances in Electrophilic Amination Reactions, Synthesis, 2014, 46, 2258–2271 CrossRef CAS.
- P. Starkov, T. F. Jamison and I. Marek, Electrophilic Amination: The Case of Nitrenoids, Chem. – Eur. J., 2015, 21, 5278–5300 CrossRef CAS PubMed.
- X. Dong, Q. Liu, Y. Dong and H. Liu, Transition-Metal-Catalyzed Electrophilic Amination: Application of O-Benzoylhydroxylamines in the Construction of the C–N Bond, Chem. – Eur. J., 2017, 23, 2481–2511 CrossRef CAS PubMed.
- P. V. Kattamuri, J. Yin, S. Siriwongsup, D.-H. Kwon, D. H. Ess, Q. Li, G. Li, M. Yousufuddin, P. F. Richardson, S. C. Sutton and L. Kürti, Practical Singly and Doubly Electrophilic Aminating Agents: A New, More Sustainable Platform for Carbon–Nitrogen Bond Formation, J. Am. Chem. Soc., 2017, 139, 11184–11196 CrossRef CAS PubMed.
- Z. Zhou and L. Kürti, Electrophilic Amination: An Update, Synlett, 2019, 30, 1525–1535 CrossRef CAS.
- L. G. O'Neil and J. F. Bower, Electrophilic Aminating Agents in Total Synthesis, Angew. Chem., Int. Ed., 2021, 60, 25640–25666 CrossRef PubMed.
-
Z. Zhou and L. Kürti, in Methodologies in Amine Synthesis, 2021, pp. 1–30 Search PubMed.
- L. Legnani, B. N. Bhawal and B. Morandi, Recent Developments in the Direct Synthesis of Unprotected Primary- Amines, Synthesis, 2017, 49, 776–789 CAS.
- J. Liu, X. Qiu, X. Huang, X. Luo, C. Zhang, J. Wei, J. Pan, Y. Liang, Y. Zhu, Q. Qin, S. Song and N. Jiao, From alkylarenes to anilines via site-directed carbon–carbon amination, Nat. Chem., 2019, 11, 71–77 CrossRef CAS PubMed.
- Z. Zhou, Z. Ma, N. E. Behnke, H. Gao and L. Kürti, Non-Deprotonative Primary and Secondary Amination of (Hetero)Arylmetals, J. Am. Chem. Soc., 2017, 139, 115–118 CrossRef CAS PubMed.
- N. E. Behnke, R. Kielawa, D.-H. Kwon, D. H. Ess and L. Kürti, Direct Primary Amination of Alkylmetals with NH-Oxaziridine, Org. Lett., 2018, 20, 8064–8068 CrossRef CAS PubMed.
- L. Legnani, G. Prina-Cerai, T. Delcaillau, S. Willems and B. Morandi, Efficient access to unprotected primary amines by iron-catalyzed aminochlorination of alkenes, Science, 2018, 362, 434–439 CrossRef CAS PubMed.
- S. Zhang, J. del Pozo, F. Romiti, Y. Mu, S. Torker and A. H. Hoveyda, Delayed catalyst function enables direct enantioselective conversion of nitriles to NH2-amines, Science, 2019, 364, 45–51 CrossRef CAS.
- V. C. M. Gasser, S. Makai and B. Morandi, The advent of electrophilic hydroxylamine-derived reagents for the direct preparation of unprotected amines, Chem. Commun., 2022, 58, 9991–10003 RSC.
- G. Boche, P. Andrews, K. Harms, M. Marsch, K. S. Rangappa, M. Schimeczek and C. Willeke, Crystal and Electronic Structure of Stable Nitrenium Ions. A Comparison with Structurally Related Carbenes, J. Am. Chem. Soc., 1996, 118, 4925–4930 CrossRef CAS.
- Y. Tulchinsky, M. A. Iron, M. Botoshansky and M. Gandelman, Nitrenium ions as ligands for transition metals, Nat. Chem., 2011, 3, 525–531 CrossRef CAS PubMed.
- Y. Tulchinsky, S. Kozuch, P. Saha, M. Botoshansky, L. J. W. Shimon and M. Gandelman, Cation–cation bonding in nitrenium metal complexes, Chem. Sci., 2014, 5, 1305–1311 RSC.
- F. Heims, F. F. Pfaff, S.-L. Abram, E. R. Farquhar, M. Bruschi, C. Greco and K. Ray, Redox Non-Innocence of a N-Heterocyclic Nitrenium Cation Bound to a Nickel–Cyclam Core, J. Am. Chem. Soc., 2014, 136, 582–585 CrossRef CAS PubMed.
- Y. Tulchinsky, S. Kozuch, P. Saha, A. Mauda, G. Nisnevich, M. Botoshansky, L. J. W. Shimon and M. Gandelman, Coordination Chemistry of N-Heterocyclic Nitrenium-Based Ligands, Chem. – Eur. J., 2015, 21, 7099–7110 CrossRef CAS PubMed.
- H. Levy Vahav, A. Pogoreltsev, Y. Tulchinsky, N. Fridman, A. Börner and M. Gandelman, Synthesis and Characteristics of Iridium Complexes Bearing N-Heterocyclic Nitrenium Cationic Ligands, Organometallics, 2019, 38, 2494–2501 CrossRef CAS.
- A. Pogoreltsev, Y. Tulchinsky, N. Fridman and M. Gandelman, Nitrogen Lewis Acids, J. Am. Chem. Soc., 2017, 139, 4062–4067 CrossRef CAS PubMed.
- J. Zhou, L. L. Liu, L. L. Cao and D. W. Stephan, Nitrogen-Based Lewis Acids: Synthesis and Reactivity of a Cyclic (Alkyl)(Amino)Nitrenium Cation, Angew. Chem., Int. Ed., 2018, 57, 3322–3326 CrossRef CAS PubMed.
- J. Zhou, L. L. Liu, L. L. Cao and D. W. Stephan, An umpolung of Lewis acidity/basicity at nitrogen by deprotonation of a cyclic (amino)(aryl)nitrenium cation, Chem. Commun., 2018, 54, 4390–4393 RSC.
- D. Zhu, Z.-W. Qu, J. Zhou and D. W. Stephan, The Reactivity of Isomeric Nitrenium Lewis Acids with Phosphines, Carbenes, and Phosphide, Chem. – Eur. J., 2021, 27, 2861–2867 CrossRef CAS PubMed.
- I. Avigdori, K. Singh, A. Pogoreltsev, A. Kaushansky, N. Fridman and M. Gandelman, Nitrenium Salts in Intramolecular Lewis Pairs, Z. Anorg. Allg. Chem., 2023, 649, e202200326 CrossRef CAS.
- I. Avigdori, A. Pogoreltsev, A. Kaushanski, N. Fridman and M. Gandelman, Frustrated Lewis Pairs Comprising Nitrogen Lewis Acids for Si–H Bond Activation, Angew. Chem., Int. Ed., 2020, 59, 23476–23479 CrossRef CAS PubMed.
- M. Mehta and J. M. Goicoechea, Nitrenium Salts in Lewis Acid Catalysis, Angew. Chem., Int. Ed., 2020, 59, 2715–2719 CrossRef CAS PubMed.
- K. Singh, I. Avigdori, A. Kaushansky, N. Fridman, D. Toledano and M. Gandelman, New Generation of Nitrenium Salts: Catalytic Hydrosilylation of Imines and a Mechanism of Action of Nitrogen Lewis Acids, ACS Catal., 2022, 12, 6831–6839 CrossRef CAS.
- D. Ranolia, I. Avigdori, K. Singh, A. Koronatov, N. Fridman and M. Gandelman, Triazolium Salts as Lewis Acid Catalysts, Org. Lett., 2022, 24, 3915–3919 CrossRef CAS PubMed.
- T. Danelzik, S. Joseph, C. Mück-Lichtenfeld, C. G. Daniliuc and O. García Mancheño, Benzotriazolium Salts: Emergent Readily Accessible Bench-Stable Lewis Acid Catalysts, Org. Lett., 2022, 24, 6105–6110 CrossRef CAS PubMed.
- K.-Q. Chen, B.-B. Zhang, Z.-X. Wang and X.-Y. Chen, N-Heterocyclic Nitreniums Can Be Employed as Photoredox Catalysts for the Single-Electron Reduction of Aryl Halides, Org. Lett., 2022, 24, 4598–4602 CrossRef CAS PubMed.
- X.-D. Su, Z.-S. Yang, W. Gong, Z.-X. Wang and X.-Y. Chen, Additive free, N-heterocyclic nitrenium catalyzed photoreduction of cycloketone oxime esters, Org. Chem. Front., 2023, 10, 1160–1165 RSC.
- L. Bao, Z.-X. Wang and X.-Y. Chen, Photoinduced N-Heterocyclic Nitrenium-Catalyzed Single Electron Reduction of Acyl Fluorides for Phenanthridine Synthesis, Org. Lett., 2023, 25, 565–568 CrossRef CAS PubMed.
- W. Liu, A. Vianna, Z. Zhang, S. Huang, L. Huang, M. Melaimi, G. Bertrand and X. Yan, Mesoionic carbene-Breslow intermediates as super electron donors: Application to the metal-free arylacylation of alkenes, Chem Catal., 2021, 1, 196–206 CrossRef CAS.
- A. Koronatov, A. Mauda, B. Tumansky, A. Kaushansky, N. Fridman, D. Bravo-Zhivotovskii and M. Gandelman, Multimodal Reactivity of N–H Bonds in Triazanes and Isolation of a Triazinyl Radical, J. Am. Chem. Soc., 2022, 144, 23642–23648 CrossRef CAS PubMed.
- G. L. Bartholomew, F. Carpaneto and R. Sarpong, Skeletal Editing of Pyrimidines to Pyrazoles by Formal Carbon Deletion, J. Am. Chem. Soc., 2022, 144, 22309–22315 CrossRef CAS PubMed.
- J. Woo, A. H. Christian, S. A. Burgess, Y. Jiang, U. F. Mansoor and M. D. Levin, Scaffold hopping by net photochemical carbon deletion of azaarenes, Science, 2022, 376, 527–532 CrossRef CAS PubMed.
- S. H. Kennedy, B. D. Dherange, K. J. Berger and M. D. Levin, Skeletal editing through direct nitrogen deletion of secondary amines, Nature, 2021, 593, 223–227 CrossRef CAS PubMed.
- B. A. Wright, A. Matviitsuk, M. J. Black, P. García-Reynaga, L. E. Hanna, A. T. Herrmann, M. K. Ameriks, R. Sarpong and T. P. Lebold, Skeletal Editing Approach to Bridge-Functionalized Bicyclo[1.1.1]pentanes from Azabicyclo[2.1.1]hexanes, J. Am. Chem. Soc., 2023, 145, 10960–10966 CrossRef CAS PubMed.
- M. Kamitani, B. Nakayasu, H. Fujimoto, K. Yasui, T. Kodama and M. Tobisu, Single-carbon atom transfer to α,β-unsaturated amides from N-heterocyclic carbenes, Science, 2023, 379, 484–488 CrossRef CAS PubMed.
- L. V. Metman, P. Del Dotto, P. van den Munckhof, J. Fang, M. M. Mouradian and T. N. Chase, Amantadine as treatment for dyskinesias and motor fluctuations in Parkinson's disease, Neurology, 1998, 50, 1323 CrossRef CAS PubMed.
Footnotes |
† Electronic supplementary information (ESI) available. CCDC 2221687–2221690. For ESI and crystallographic data in CIF or other electronic format see DOI: https://doi.org/10.1039/d3sc04268e |
‡ These authors contributed equally to this work. |
|
This journal is © The Royal Society of Chemistry 2023 |