DOI:
10.1039/D3RA07567B
(Paper)
RSC Adv., 2023,
13, 34510-34519
High-efficiency energy transfer in the strong orange-red-emitting phosphor CeO2:Sm3+, Eu3+
Received
6th November 2023
, Accepted 17th November 2023
First published on 24th November 2023
Abstract
High-efficiency energy transfer (ET) from Sm3+ to Eu3+ leads to dominant red emission in Sm3+, Eu3+ co-doped single-phase cubic CeO2 phosphors. In this work, a series of Sm3+ singly and Sm3+/Eu3+ co-doped CeO2 cubic phosphors was successfully synthesized by solution combustion followed by heat treatment at 800 °C in air. The crystal structure, morphology, chemical element composition, and luminescence properties of the obtained phosphors were investigated using X-ray diffraction, scanning electron microscopy, energy-dispersive X-ray spectroscopy, and photoluminescence analysis. Under 360 nm excitation, the Sm3+ singly doped CeO2 phosphor emitted strong yellow-red light at 573 nm (4G5/2–6H5/2) and 615 nm (4G5/2–6H7/2). Meanwhile, the CeO2:Sm3+, Eu3+ phosphors showed the emission characteristic of both Sm3+ and Eu3+, with the highest emission intensity at 631 nm. The emission intensity of Sm3+ decreased with increasing Eu3+ content, suggesting the ET from Sm3+ to Eu3+ in the CeO2:Sm3+, Eu3+ phosphors. The decay kinetics of the 4G5/2–6H5/2 transition of Sm3+ in the CeO2:Sm3+, Eu3+ phosphors were investigated, confirming the high-efficiency ET from Sm3+ to Eu3+ (reached 84%). The critical distance of energy transfer (RC = 13.7 Å) and the Dexter theory analysis confirmed the ET mechanism corresponding to the quadrupole–quadrupole interaction. These results indicate that the high-efficiency ET from Sm3+ to Eu3+ in CeO2:Sm3+, Eu3+ phosphors is an excellent strategy to improve the emission efficiency of Eu3+.
1. Introduction
White-light emitting diodes (WLEDs) have been extensively used in many fields of application, such as in solid lighting, display devices, and optoelectronic devices, because of their high luminous efficiency, long lifetime, energy saving, and environment friendliness.1–3 A popular method for manufacturing WLEDs is combining tricolor phosphor powder (blue, green, and red phosphors) with an ultraviolet (UV) InGaN chip.4,5 However, these WLEDs present a high correlated color temperature and low color rendering index due to the lack of a red component.6,7 To overcome these drawbacks, scholars should explore new red phosphors for WLED applications.
The europium trivalent ion (Eu3+) is an important rare-earth (RE) ion that has been widely used as an activator in red-emitting phosphors for WLEDs.8–10 The red emission of Eu3+ is originally from electric dipole transitions. Notably, Eu3+-doped phosphors typically exhibit relatively narrow absorption in UV and near-UV regions because of the spin-forbidden transition of Eu3+, resulting in low emission efficiency.11,12 This defect can be compensated by introducing sensitizing ions, such as Tb3+, Bi3+, Gd3+, and Sm3+,13–15 which can absorb excitation energy efficiently and transfer it to Eu3+. Sm3+ is a popular sensitizer for improving the efficiency emission of Eu3+ ion due to the small energy difference between the 4G5/2 level of Sm3+ and the 5D0 level of Eu3+ (about 600 cm−1), leading to easy phonon-assisted energy transfer (ET).13 Hence, the energy transfer between Sm3+ and Eu3+ ions was widely investigated in a variety of host lattices.12,16,17 J. Wu et al.16 found that the ET efficiency from Sm3+ to Eu3+ up to 65% in YPO4:Sm3+, Eu3+ phosphor corresponds to the electric dipole–electric dipole interaction mechanism. Y. Li et al.17 reported that ET efficiency from Sm3+ to Eu3+ was 13.7% in La2CaB10O19:Sm3+, Eu3+ phosphor, further confirmed by Judd–Ofelt theory. Meanwhile, X. Zhang et al.12 developed Ca2GdNbO6:Sm3+, Eu3+ phosphor with high quantum yield (82.7%), excellent thermal stability, and up to 28.6% ET efficiency. In addition, the LED device fabricated based on this phosphor emits bright white light, and CCT = 5400 K, Ra = 92.8. Clearly, the addition of two or more RE ions into a luminescent material can effectively control multi-color luminescence and achieve ET, thereby improving the luminous efficiency of the phosphors.7,11,13,18 Besides, several parameters, including the degree of crystallinity, the dopant concentration, and the host material, influence the emission efficiency of the phosphors. In this case, the host lattice selection is important since it influences the luminescent efficiency and the application potential of the material.
Cerium oxide (CeO2) host lattice exhibits low phonon energy, high thermal stability, low toxicity, and excellent physicochemical properties.19–21 It is an attractive UV-excited host material due to its strong light absorption through the O2−–Ce4+ charge transfer band. Therefore, RE-doped CeO2 was widely investigated for many applications, such as catalysis, sensors, optoelectronic devices, and UV-LEDs.20,22 The small difference between the ionic radius of Ce4+ and RE3+ suggests that it can provide favorable sites for introducing RE ions into the CeO2 host lattice. Furthermore, the spectral overlap of the CeO2 host's charge transfer band and the 4f–4f transitions of the RE ions leads to highly efficient energy transfer from host to RE ions.23,24 G. Vimal et al.23 have observed the energy transfer from the CeO2 host to Eu3+ in CeO2:Sm3+, Eu3+ phosphor leading to improved efficiency emission of the systems. Meanwhile, an intense red emission of Eu3+ was achieved in CeO2:Eu3+, Bi3+,24 and CeO2:Eu3+, M+ (M: Na, K, Li)25 phosphors through change the symmetry of the host and charge compensation mechanism, respectively. However, achieving a high-efficiency energy transfer in orange-red-emitting phosphor CeO2:Sm3+, Eu3+ synthesized by solution combustion and its energy transfer mechanism investigation have not been well documented.
In this work, a series of Sm3+ singly and Sm3+/Eu3+ co-doped CeO2 phosphors was prepared using solution combustion method. The crystal structure and chemical element composition were explored using X-ray diffraction and energy-dispersive X-ray spectroscopy analyses. The luminescence properties and ET mechanisms were systematically investigated. The phosphors emitted orange to red emissions with an enhancement of Eu3+ doping because the efficiency of ET from Sm3+ to Eu3+ reached 84%. Furthermore, the ET mechanism between Sm3+ and Eu3+ was discussed in detail.
2. Experimental procedure
A series of CeO2:xSm3+ (x = 0.02, 0.04, 0.06, 0.08, 0.10, and 0.12 mol%), CeO2:0.04Eu, and CeO2:0.04Sm3+, yEu3+ (y = 0, 0.02, 0.04, 0.06, 0.08, and 0.10 mol%) phosphors was prepared using solution combustion followed by heat treatment at 800 °C for 4 h in air. Ce(NO3)3·6H2O (Sigma-Aldrich, 99.9%), Sm(NO3)3·6H2O (Sigma-Aldrich, 99.99%), urea, and Eu2O3 (Sigma-Aldrich, 99.99%) were used as raw materials. These materials were exactly weighed and dissolved in deionized water and HNO3 solution (Eu2O3) under magnet-stirred conditions to obtain aqueous solutions Ce3+ (0.5 M), Sm3+ (0.5 M), and Eu3+ (0.5 M), respectively. The stoichiometric amounts of the solutions Ce3+, Sm3+, and Eu3+ were added together to get 0.5 M aqueous solution and stirred for 30 min at room temperature. After that, the 20 mol% urea (compared with the Ce3+ content) was added to the system under magnetic stirring for 3 h at 80 °C. Next, the system was transferred to a corundum crucible (200 ml) for combustion reaction at 400 °C for 4 h. Then, the powder was heat-treated at 800 °C for 4 h in air, with a heating rate of 3 °C per minute. Finally, obtained phosphor powder was naturally cooled to room temperature and characterized. The crystal structure and chemical element composition of the phosphors were determined by X-ray diffraction (Bruker D8 Advance) and energy-dispersive X-ray spectroscopy (JEM 1010, JEOL Technique, Tokyo, Japan) analyses. The luminescence properties, lifetime, and ET mechanisms were investigated using a NANOLOG spectrophotometer (Horiba, USA). Decay curves of the investigated phosphors were also evaluated.
3. Results and discussion
3.1. XRD analysis
Fig. 1a presents the XRD patterns of CeO2:0.04Sm3+ phosphors without and co-doped with Eu3+. All the diffraction peaks are consistent with the standard card (PDF # 01-075-8371), confirming the formation of single-phase cubic fluorite-type CeO2 with space group Fm3m and no impurity phase is present. The crystal structure of CeO2 includes Ce4+ sites with eight coordinates (including eight nearest-neighbor oxygen anions) and O2− sites with four coordinates (surrounded by four nearest-neighbor cerium cations).26 High-intensity diffraction peaks were observed corresponding to typical planes of cubic phase CeO2 including (111), (200), (220), (311), (222), and (400). Significantly, the diffraction peaks shifted toward a lower 2θ angle (Fig. 1b) after being doped with a larger ionic radius Eu3+ (1.06 Å) since the ionic radius of Ce4+ is 0.97 Å, leading to a lattice expansion,18 confirming that Eu3+ ions have been incorporated in the Ce4+ cites of the CeO2 host. Furthermore, the crystallite size of all investigated phosphors was determined using the Scherrer equation:27 |
 | (1) |
where D is crystallite size (nm), 0.9 is Scherrer constant, λ is the wavelength of the X-ray sources (0.15406 nm), β (radians) and θ (radians) are full width at half maximum (FWHM) and peak position, respectively. The diffraction peak corresponding to the (111) plane of CeO2 was chosen to calculate crystallite size, as shown in Table 1. Notably, the crystallite size slightly increases with increasing Eu3+ doping, suggesting a successful introduction of Eu3+ in the systems. These results confirm that the presence of Eu3+ in the systems leads to enhanced crystallinity and crystallite size, which could improve the emission efficiency of the phosphors.
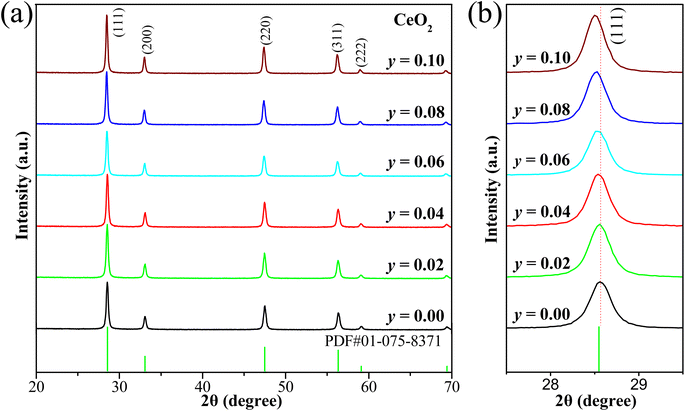 |
| Fig. 1 (a) XRD patterns of the investigated samples CeO2:0.04Sm3+, yEu3+ (y = 0.00, 0.02, 0.04, 0.06, 0.08, and 0.10), and (b) XRD patterns at 2θ angle of 27.5° to 29.5° of all samples. | |
Table 1 The crystallite size of samples with different Eu3+ doping content
CeO2:0.04Sm3+, yEu3+ |
FWHM |
2θ° |
Cos(θ) |
Crystal size (nm) |
y = 0.00 |
0.005655 |
28.60 |
0.969016 |
25.3 |
y = 0.02 |
0.005498 |
28.58 |
0.969059 |
26.1 |
y = 0.04 |
0.005236 |
28.54 |
0.969145 |
27.3 |
y = 0.06 |
0.004992 |
28.52 |
0.969188 |
28.6 |
y = 0.08 |
0.004817 |
28.52 |
0.969209 |
29.7 |
y = 0.10 |
0.004643 |
28.50 |
0.969231 |
30.8 |
3.2. Morphology and chemical element composition analysis
Fig. 2a and b show the SEM images of CeO2:0.04Sm3+ and CeO2:0.04Sm3+, 0.04Eu3+, respectively. The un-doped sample (CeO2:0.04Sm3+) shows the agglomeration of spherical-like nanoparticles with an average size of approximately 40.5 nm (inset of Fig. 2a). Meanwhile, the doped sample (CeO2:0.04Sm3+, 0.04Eu3+) shows uniform grains, with boundary distribution and an average particle size of about 46.5 nm (inset of Fig. 2b). This finding suggests the high crystallinity of the obtained phosphors. However, the morphology of the samples did not significantly change, indicating that it is not a main parameter that can influence luminescent properties. Chemical element composition was analyzed using EDS to confirm the presence of ions doped into the CeO2 host. Fig. 2c shows the presence of Ce, O, and Sm, which are attributed to the host lattice and doping ion. Meanwhile, Fig. 2d indicates the presence of Ce, O, Eu, and Sm with percent composition shown in the inset of Fig. 2d. Impurity elements were not observed, thereby confirming the high purity of the obtained phosphors.
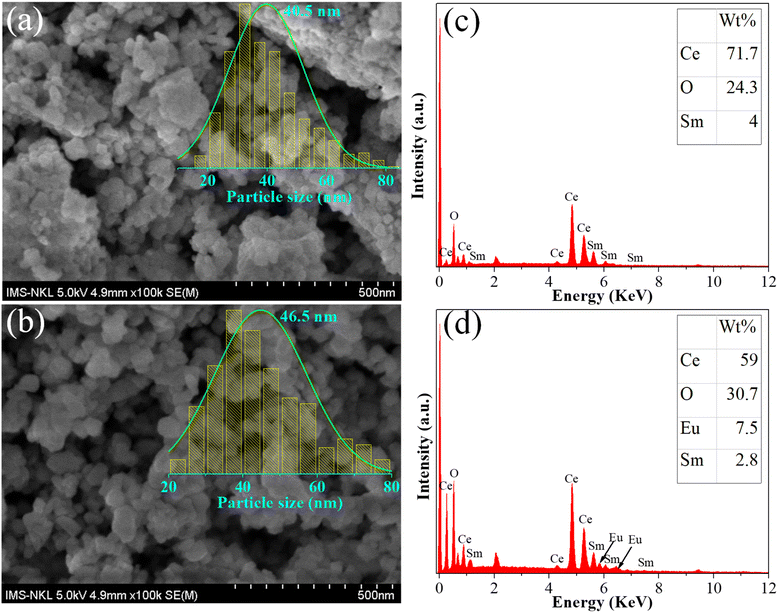 |
| Fig. 2 SEM image of CeO2:0.04Sm3+ (a) and CeO2:0.04Sm3+, 0.04Eu3+ (b); and EDS spectra of CeO2:0.04Sm3+ (c) and CeO2:0.04Sm3+, 0.04Eu3+ (d). | |
3.3. Luminescence properties
3.3.1. Luminescence properties of CeO2:Sm and CeO2:Sm, Eu phosphors. Fig. 3a shows the photoluminescence excitation (PLE) spectra of CeO2:0.02Sm3+ with monitoring emission wavelengths of 573 and 615 nm. The absorption band from 250 nm to 300 nm is attributed to the charge transfer band from O2− to Ce4+. The highest band absorption with a peak at 360 nm corresponds to the overlap between the 6H5/2–4D3/2 transition of Sm3+ and the charge transfer band O2−–Ce4+/Sm3+. The weak peak absorption at 405 nm corresponds to the 6H5/2–4F7/2 transition of Sm3+. The band absorption with 573 nm emission is higher than that with 615 nm, indicating the dominant orange emission of the phosphors. Meanwhile, Fig. 3b presents the emission spectra of all investigated samples of CeO2:xSm3+ (x = 0.02, 0.04, 0.06, 0.08, 0.10, and 0.12 mol%) monitoring at excited wavelength of 360 nm. Under 360 nm excitation, the obtained phosphor showed characteristic emission of Sm3+, such as at 573 (4G5/2–6H5/2), 631 (4G5/2–6H6/2), and 660 nm (4G5/2–6H9/2). The emission intensity of the samples reached the maximum value of 0.04 mol% Sm3+ doping and then decreased with increasing Sm3+ content due to the concentration quenching effect. The sample CeO2:0.04 Sm3+ showed the highest emission intensity and was selected for synthesis of CeO2:0.04Sm3+, yEu3+ phosphor.
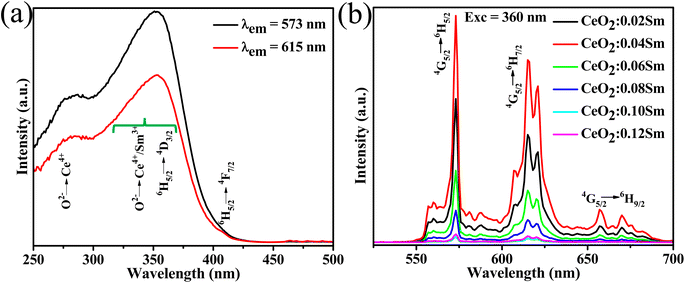 |
| Fig. 3 (a) PLE spectra of CeO2:0.04Sm3+ and (b) PL spectra of CeO2:xSm3+ (x = 0.02, 0.04, 0.06, 0.08, 0.10, and 0.12 mol%). | |
Fig. 4a presents the PLE spectra of samples CeO2:0.04Eu3+ and CeO2:0.04Sm3+, 0.04Eu3+ with monitoring emission wavelength at 631 nm. In the typical two samples, the absorption bands from 250 nm to 300 are attributed to the charge transfer band from O2− to Ce4+, similar to the PLE spectra of CeO2:0.04Sm3+. The highest absorption band around at 360 nm corresponds to the overlap between the 6H5/2–4D3/2 transition of Sm3+28 and the charge transfer band (O2− to Ce4+/Sm3+/Eu3+) of the host lattice (sample CeO2:0.04Sm3+, 0.04Eu3+). Fig. 4a shows typical absorption peaks at 405 nm corresponding to the 6H5/2–4F7/2 transition of Sm3+, while the absorption peak at 395 nm (7F0–5L6), 466 nm (7F0–5D2), 526/532 (7F0–5D1/7F1–5D1) nm and 580/587 nm (7F0–5D0/7F1–5D0) is attributed to the Eu3+. These results confirm the presence of dopant ions in the host lattice and the successful synthesis of the phosphors. In this case the absorption intensity from 250–400 nm of CeO2:0.04Sm3+, 0.04Eu3+ was much higher than the CeO2:0.04Eu3+. Fig. 4b shows the difference in emission spectra between three phosphors (CeO2:0.04Sm3+, CeO2:0.04Eu3+ and CeO2:0.04Sm3+, 0.04Eu3+) under 360 nm excitation. Notably, the CeO2:0.04Sm3+ phosphor emits a dominant peak of yellow light (573 nm), and the CeO2:0.04Eu3+ phosphor emits an orange light (590 nm); however, the 0.04Sm3+/0.04Eu3+ co-doped CeO2 exhibited a dominant peak red emission (631 nm). The photoluminescence spectra of all investigated samples are shown in Fig. 4c. Under 360 nm excitation wavelengths, the CeO2:0.04Sm3+, yEu3+ (y = 0, 0.02, 0.04, 0.06, 0.08, and 0.10) phosphors emitted strong orange-red band at 590 nm (5D0–7F1) and 610–631 nm (5D0–7F2) of Eu3+. Notably, the emission intensities at 573 nm corresponding to the 4G5/2–6H5/2 transition of Sm3+ decreased with increasing Eu3+ doping content. By contrast, the emission intensities at 631 nm depended on Eu3+ content and reached the maximum value at 0.04 mol% Eu3+ doping (Fig. 4d). Hence, the red-emitting phosphors (CeO2:0.04Sm3+, 0.04Eu3+) could be excited by 360 nm commercial LED chip, making it suitable for solid lighting applications. Furthermore, the red/orange (R/O) emission intensity ratios of the CeO2:0.04Sm3+, yEu3+ (y = 0, 0.02, 0.04, 0.06, 0.08, and 0.10) phosphors can estimate the asymmetry around the Eu3+ sites in the host lattice,29 which was calculated as shown in Fig. 4e. The R/O ratio increases with the enhancement of Eu3+ content, confirming the asymmetry of the crystal field increased. These results supported increasing the electric-dipole transition probability of the Eu3+, resulting in dominant red emissions. In addition, the Commission Internationale de I'Eclairage (CIE) chromaticity coordinates of resulted phosphors (Fig. 4f) were calculated from their emission spectra, as shown in Table 2. CeO2:0.04Sm3+, 0.04Eu3+ shows CIE coordinates (0.622, 0.375) close to pure red.14 Furthermore, these CIE coordinates were used for calculating the color purity of the investigated phosphors, which can be described as eqn (2):30
|
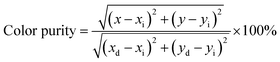 | (2) |
where (
x,
y) and (
xi = 0.3333,
yi = 0.3333) are the color coordinates of the investigated samples and the white light source, respectively; (
xd,
yd) corresponds to the color coordinates of the dominant wavelength.
31 These values (
x,
y), (
xi,
yi), and (
xd,
yd) were plugged into
eqn (2), and the color purity of the resulting phosphors is displayed in
Table 2. The color purity of the samples increased with enhancing Eu
3+ doping content and reached a maximum value of 89.74% (
Table 2). The findings confirm the high efficiency ET from Sm
3+ to Eu
3+ in the systems developed. Furthermore, the correlation of phosphors with color temperature (CCT) was calculated to explore the nature of the emitted light by using McCammys' equation:
32 |
CCT = 5520.33 − 6823n + 3525n2 − 449n3
| (3) |
where (
x,
y) is the CIE coordinates of all samples; (
xe = 0.338,
ye = 0.186) is the chromaticity epicenter;
n = (
x −
xe)/(
y −
ye). The resulting CCT values are shown in
Table 2. The values increased from 1790.8 K to 1973 K with increasing Eu
3+ doping content.
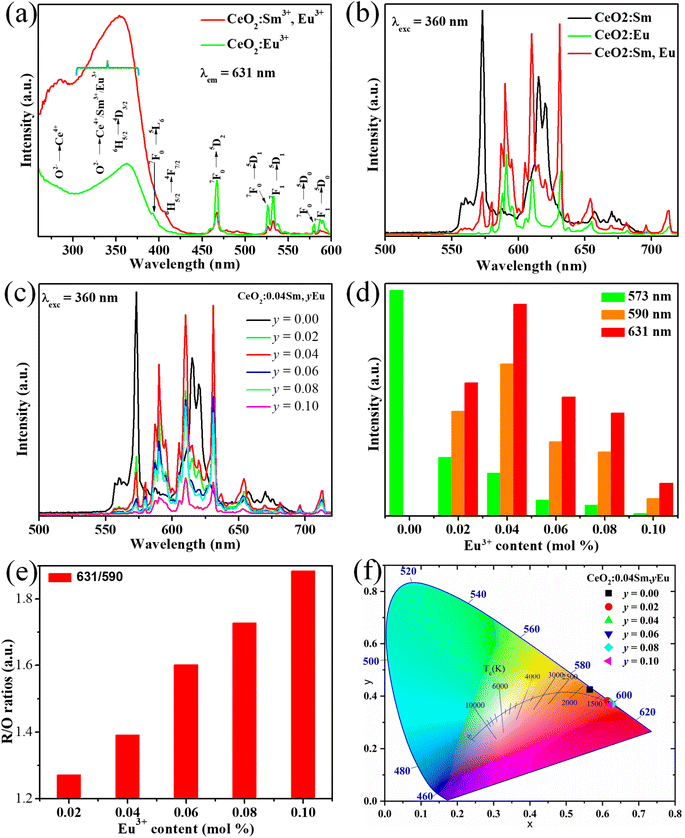 |
| Fig. 4 (a) PLE spectra of samples CeO2:0.04Eu3+ and CeO2:0.04Sm3+, 0.04Eu3+; (b) emission spectra of CeO2:Sm3+, CeO2:Eu3+, and CeO2:Sm3+, Eu3+ phosphors; (c) PL emission spectra of samples CeO2:0.04Sm3+, yEu3+ (y = 0.00, 0.02, 0.04, 0.06, 0.08, and 0.10); (d) dependence of the 4G5/2–6H5/2 (Sm3+) and 5D0–7F1/7F2 (Eu3+) transitions on the Eu3+ doping content; (e) red/orange (R/O) emission intensity ratios of investigated samples as Eu3+ content increased; (f) CIE coordinate chromaticity of CeO2:0.04Sm3+, yEu3+ phosphors (y = 0.00, 0.02, 0.04, 0.06, 0.08, and 0.10). | |
Table 2 CIE chromaticity coordinates, color purity, and CCT values of all investigated samples
Samples |
Chromaticity coordinates (x, y) |
Color purity (%) |
CCT (K) |
CeO2:0.04Sm |
(0.565, 0.425) |
77.60 |
1847 |
CeO2:0.04Sm, 0.02Eu |
(0.614, 0.383) |
86.93 |
1790.8 |
CeO2:0.04Sm, 0.04Eu |
(0.622, 0.375) |
88.95 |
1885.8 |
CeO2:0.04Sm, 0.06Eu |
(0.624, 0.371) |
89.36 |
1936.2 |
CeO2:0.04Sm, 0.08Eu |
(0.626, 0.370) |
89.74 |
1974.8 |
CeO2:0.04Sm, 0.10Eu |
(0.621, 0.366) |
88.10 |
1973.1 |
3.3.2. Decay curves and ET efficiency of all obtained phosphors. The decay curve of Sm3+ in CeO2:0.04Sm3+, yEu3+ phosphor (y = 0.00, 0.02, 0.04, 0.06, 0.08, and 0.10) can be used to study the emission mechanism and confirm the efficiency of ET from Sm3+ to Eu3+. The luminescence decay curves associated with the transition 4G5/2–6H5/2 of Sm3+ of all samples were monitored at the emission wavelength of 573 nm and the excitation wavelength of 360 nm (Fig. 5a). The radiative lifetime (τ) of the samples well fitted by bi-exponential function:33 |
y = y0 + A1e−t/τ1 + A2e−t/τ2
| (4) |
where y is the emission intensity at time t; y0 is the initial emission intensity; A1 and A2 are constants; τ1 and τ2 are decay times. τ1 is the decay component attributed to Sm3+ on the surface or near defect sites. By contrast, τ2 is the decay component associated with Sm3+ sites substituted for Ce4+ ions on the CeO2 host lattice.33 The average lifetimes (τ) can be described as eqn (5):34 |
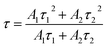 | (5) |
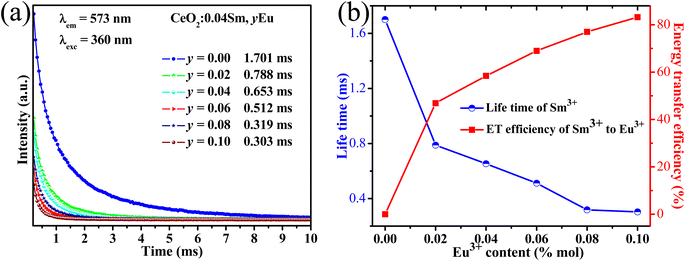 |
| Fig. 5 (a) Decay curve of samples monitored at the excitation wavelength of 360 nm and the emission wavelength of 573 nm. (b) Lifetime of all sample depended on Eu3+ content (blue line) and ET efficiency from Sm3+ to Eu3+ (red line). | |
The τ values are 1.701, 0.788, 0.653, 0.512, 0.319, and 0.303 ms, corresponding to CeO2:0.04Sm3+, yEu3+ phosphors (y = 0, 0.02, 0.04, 0.06, 0.08, and 0.10 mol%, respectively), as shown in Table 3. The average lifetime decreased with increasing Eu3+ doping content, thereby confirming the ET from Sm3+ to Eu3+.12,35 Furthermore, ET efficiency was estimated using eqn (6):36
|
 | (6) |
where
τS and
τS0 are the lifetimes of Sm
3+ with and without Eu
3+, respectively. The calculated ET efficiency values are shown in
Fig. 5b. The ET efficiency from Sm
3+ to Eu
3+ increased with increasing doping content of Eu
3+ and reached the maximum value of 84% with 0.10 mol% Eu
3+. The ET efficiency in this work is significantly higher than in other previous works (
Table 4). These results indicated that the phosphor conferred it as a suitable red-emitting phosphor for WLEDs.
Table 3 Fitting decay curves for CeO2:0.04Sm3+, xEu3+ (x = 0, 0.02, 0.04, 0.06, 0.08 and 0.10 mol%)
CeO2:0.04Sm, yEu |
A1 |
τ1 |
A2 |
τ2 |
τaverage (ms) |
y = 0.00 |
82.25 |
1.11 |
227 |
0.23 |
1.701 |
y = 0.02 |
319.7 |
1.12 |
926 |
0.23 |
0.788 |
y = 0.04 |
932 |
0.21 |
260 |
0.99 |
0.653 |
y = 0.06 |
131 |
0.7 |
743 |
0.17 |
0.512 |
y = 0.08 |
166.9 |
0.85 |
746 |
0.2 |
0.319 |
y = 0.10 |
653.6 |
0.15 |
76.76 |
0.62 |
0.309 |
Table 4 Comparison of ET efficiency from Sm3+ to Eu3+ on the proposed phosphor and other existing phosphors
Phosphors |
Content of Eu3+ (mol%) |
ET efficiency (%) |
References |
CeO2:Sm3+, Eu3+ |
0.04 |
84.00 |
This work |
La2MgTiO6:Sm3+, Eu3+ |
0.20 |
73.50 |
35 |
YPO4:Sm3+, Eu3+ |
0.08 |
65.00 |
16 |
Ca2GdNbO6:Sm3+, Eu3+ |
0.30 |
28.60 |
12 |
Sr7Sb2O12:Sm3+, Eu3+ |
0.09 |
42.40 |
7 |
Sr3In2WO9:Sm3+, Eu3+ |
0.02 |
62.69 |
18 |
Sr3Y(BO3)3:Sm3+, Eu3+ |
0.07 |
55.69 |
33 |
3.3.3. ET mechanism. The exchange interaction or multipolar interaction is responsible for the main ET mechanism in the CeO2:Sm3+, Eu3+, which is estimated by the average critical distance (RC) between Sm3+ and Eu3+. Notably, the multipolar interaction is attributed to the RC value higher 5 Å and when the RC is about 5 Å corresponding to exchange interaction.37 The RC value can be determined by eqn (7):37 |
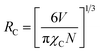 | (7) |
here, the cell volume V is 430.32 Å3; the critical doping content χC is 0.08; N is the available host cations in the cell.38 Putting all these values in the eqn (7), the RC value in CeO2:0.04Sm3+, 0.04Eu3+ was observed to be ∼13.7 Å, thus, the ET mechanism from Sm3+ to Eu3+ occurs by multipolar interaction. In this case, the Dexter theory was explored to evaluate the multipolar interaction between Sm3+ and Eu3+.39 Therefore, the rate of ET from Sm3+ to Eu3+ against the concentration of Eu3+ can be estimated as follows:39 |
 | (8) |
where C is the total doping ion content (Sm3+ and Eu3+). IS0 and IS are the emission intensity of Sm3+ without and with Eu3+ doping. The n is an electric multipolar character equal to 6, 8, and 10, corresponding to dipole–dipole, dipole–quadrupole, and quadrupole–quadrupole interactions.11 Fig. 6 shows the relationship between Cn/3 and IS0/IS, including the values of the fitting factor R2. Previous works18,33,35,40 indicate that the R2 highest value (close to 1) determines the ET mechanism in the system. Therefore, the R2 highest value in this work is 0.986, indicating the ET mechanism in CeO2:Sm3+, Eu3+ corresponding to quadrupole–quadrupole interaction.
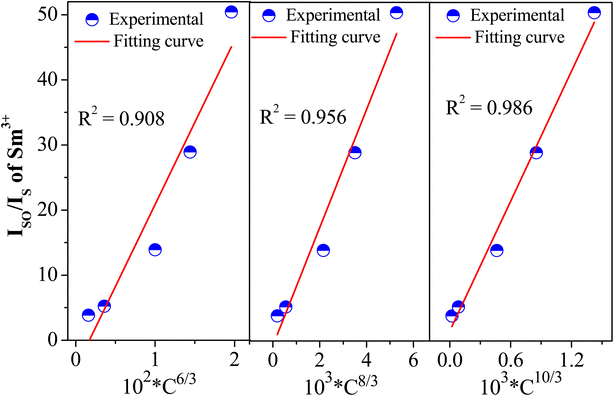 |
| Fig. 6 Dependence of the IS0/IS intensity ratio of Sm3+ on C6/3, C8/3, and C10/3 in CeO2:0.04Sm3+, yEu3+ phosphors (y = 0.00, 0.02, 0.04, 0.06, 0.08, and 0.10). | |
3.3.4. Energy level diagram of the phosphors. Furthermore, the ET process from Sm3+ to Eu3+ can be explained using an energy level diagram (Fig. 7a). Under 360 nm wavelength excitation, the electron at the 6H5/2 state of Sm3+ absorption photons move to the 4D3/2 level. In addition, the population of the 4D3/2 level is enhanced by absorption due to the charge transfer from O2− to Ce4+ of the host lattice. The electrons in the 4D3/2 level relax to the 4G5/2 state through non-radiation transition. Finally, the electrons at the 4G5/2 of Sm3+ transfer energy to the 5D0 state of Eu3+ and then go back to the ground state 7Fj (j = 0, 1, 2, and 4), thereby producing intense red emission band. The ET was confirmed by the spectral overlap between the excitation of Eu3+ and the emission of Sm3+, as shown in Fig. 7b.
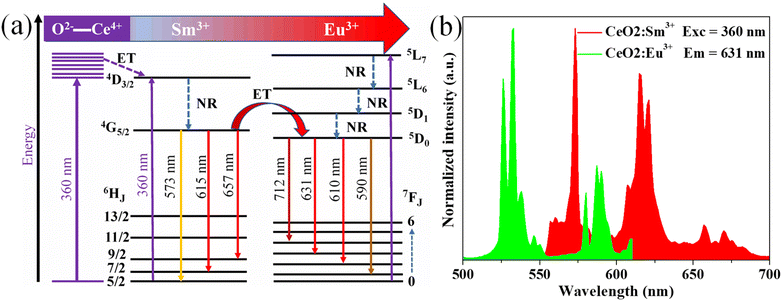 |
| Fig. 7 (a) Schematic energy-level diagram of CeO2:0.04Sm3+, yEu3+ phosphors with excitation, emission, and energy transfer; (b) the overlap between the excitation spectra of sample CeO2:0.04Eu3+ and the emission spectra of sample CeO2:0.04Sm3+. | |
4. Conclusion
CeO2:Sm3+ and CeO2:Sm3+, Eu3+ phosphors were successfully synthesized using solution combustion. The XRD analysis confirmed the formation of single-phase cubic CeO2 with space group Fm3m. Under 360 nm excitation, CeO2:Sm3+ phosphors emitted strong yellow-red band at 573 and 615 nm, corresponding to the 4G5/2–6Hj (j = 5/2, and 6/2) transitions of Sm3+. Meanwhile, CeO2:Sm3+, Eu3+ showed the emission characteristics of Sm3+ (573 nm) and Eu3+ at 590/610–631 nm. The photoluminescence spectra showed the ET from Sm3+ to Eu3+ because of the small energy difference between the 4G5/2 level of Sm3+ and the 5D0 level of Eu3+ ions. Significantly, the decay kinetics of the 4G5/2–6H5/2 transition (Sm3+) in CeO2:Sm3+, Eu3+ confirmed the high-efficiency ET from Sm3+ to Eu3+ (up to 84%). The quadrupole–quadrupole interaction between Sm3+ and Eu3+ was responsible for the ET mechanism. These results indicate that the high-efficiency ET from Sm3+ to Eu3+ in CeO2:Sm3+, Eu3+ phosphors are an excellent strategy to improve the emission efficiency of Eu3+.
Author contributions
Nguyen Van Hai: data curation, format analysis, resources, supervision, writing – original draft. Bui Thi Hoan: investigation, data curation. Nguyen Minh Tu: conceptualization, format analysis. Dinh Thi Hien: methodology, resources. Nguyen Thi Khanh Linh: format analysis, experiment, methodology, data curation. Duy-Hung Nguyen: methodology, data curation. Vu Tuan Anh: format analysis, experiment. Vuong-Hung Pham: format analysis, conceptualization. Hoang Nhu Van: investigation, format analysis, experiment, conceptualization, methodology, writing – original draft, writing – review & editing.
Conflicts of interest
The authors declare that they have no conflict of interest.
Acknowledgements
This work is funded by the Ministry of Education and Training of Vietnam, under the project B2023-SPH-06.
References
- S. Wang, Z. Song and Q. Liu, Recent progress in Ce3+/Eu2+-activated LEDs and persistent phosphors: focusing on the local structure and the electronic structure, J. Mater. Chem. C, 2023, 11, 48–96 RSC.
- M. Iwaki, K. Uematsu, M. Sato and K. Toda, Structure and Luminescence Studies of a Ce3+-Activated Ba5La3MgAl3O15 Green-Emitting Phosphor, Inorg. Chem., 2023, 62, 1250–1256 CrossRef CAS PubMed.
- P. Dang, D. Liu, G. Li, A. A. Al Kheraif and J. Lin, Recent Advances in Bismuth Ion-Doped Phosphor Materials: Structure Design, Tunable Photoluminescence Properties, and Application in White LEDs, Adv. Opt. Mater., 2020, 8, 1901993 CrossRef CAS.
- T. K. Kuttiat, M. Abraham, A. K. Kunti, N. Amador-Mendez, M. Tchernycheva and S. Das, Enriching the Deep-Red Emission in (Mg, Ba)3M2GeO8: Mn4+ (M = Al, Ga) Compositions for Light-Emitting Diodes, ACS Appl. Mater. Interfaces, 2023, 15, 7083–7101 CrossRef PubMed.
- T. Zhuang, Y. Lin, J. Jin, Z. Deng, Y. Peng, L. Gong, Z. Wang, K. Du and X. Huang, A Mechanochemically Synthesized Hybrid Bismuth Halide as Highly Efficient Red Phosphor for Blue Chip-Based WLED, Adv. Opt. Mater., 2023, 11, 2202951 CrossRef CAS.
- G. Wu, J. Xue, X. Li, Q. Bi, M. Sheng and Z. Leng, A novel red-emitting Na5W3O9F5:Eu3+ phosphor with high color purity for blue-based WLEDs, Ceram. Int., 2023, 49, 10615–10624 CrossRef CAS.
- S. Ling, F. B. Xiong, W. B. Yang, H. F. Lin and W. Z. Zhu, Novel Sm3+/Eu3+ co-doped Sr7Sb2O12 red-emitting phosphor for white LED, Inorg. Chem. Commun., 2022, 150, 110365 CrossRef.
- H. N. Van, P. D. Tam and V. H. Pham, Red and Yellow Luminescence of Eu3+/Dy3+ Co-Doped Hydroxyapatite/β-Tricalcium Phosphate Single Phosphors Synthesized Using Coprecipitation Method, J. Appl. Spectrosc., 2018, 85, 738–742 CrossRef CAS.
- H. N. Van, M. T. Y. Thanh, P. H. Vuong, P. V. Huan, V. T. N. Minh, P. A. Tuan and T. D. Hien, Experimental and theoretical studies of red emission enhancing mechanism in Al-doped CaMoO4:Eu phosphor, Opt. Mater., 2022, 132, 112831 CrossRef CAS.
- P. V. Huan, B. T. Hue, B. T. Hoan, N. T. H. Thanh, H. N. Van, C. X. Thang, H. H. Anh, P. D. Tam and P. H. Vuong, Correlation of luminescence and Judd-Ofelt intensity parameters in red ZrO2: Eu3+, Al3+ phosphor: the influences of Al3+ ions, Mater. Sci. Eng. B: Solid-State Mater. Adv. Technol., 2020, 32, 114794 CrossRef.
- S. Sharma, A. S. Rao and K. Kishore, Energy transfer dynamics in thermally stable Sm3+/Eu3+ co-doped AEAlBS glasses for near UV triggered photonic device applications, J. Non-Cryst. Solids, 2021, 580, 121392 CrossRef.
- X. Zhang, R. Cui, K. Guo, M. Zhang, J. Zhang and C. Deng, Luminescence properties of Ca2GdNbO6: Sm3+, Eu3+ red phosphors with high quantum yield and excellent thermal stability for WLEDs, Ceram. Int., 2023, 49, 15402–15412 CrossRef CAS.
- P. K. Pandey, P. Dixit, V. Chauhan and P. C. Pandey, Luminescence properties and energy transfer studies in thermally stable Bi2O3: Sm3+, Eu3+ phosphor, J. Alloys Compd., 2023, 952, 169911 CrossRef CAS.
- E. Rai, A. Roy, A. Rai, V. J. Fulari and S. B. Rai, Structural and luminescent properties and energy transfer from Tb3+ to Eu3+ in LaVO4:xTb3+/yEu3+ phosphors, J. Alloys Compd., 2023, 937, 168395 CrossRef CAS.
- X. Wu, X. Zhao, Q. Ren, L. Du, M. Pei and O. Hai, Design efficient energy transfer Ca2Al2SiO7: Bi3+, Eu3+ phosphors by cationic substitution for full-spectrum W-LED lighting, Ceram. Interfaces, 2023, 49, 18852–18860 CrossRef CAS.
- J. Wu, C. Liu, H. Jia, Y. Qi, Z. Liu, Y. Hu and F. Feng, Optical properties, energy transfer and thermal stability of spherical nano-phosphor YPO4:Eu3+:Sm3+, J. Lumin., 2022, 245, 118791 CrossRef CAS.
- Y. Li, X. Zhang, Y. Wang, S. Ma, F. Jing, Z. Hu and Y. Wu, Growth and optical properties of a Sm3+, Eu3+ co-doped La2CaB10O19 crystal, J. Cryst. Growth, 2023, 614, 127243 CrossRef CAS.
- P. Yu, R. Cui, C. Xu, G. Zhang, J. Zhang and C. Deng, A novel efficient Sr3In2WO9: Sm3+, Eu3+ phosphor for white LED applications, Opt. Laser Technol., 2022, 163, 109389 CrossRef.
- L. Li, H. K. Yang, B. K. Moon, Z. Fu, C. Guo, J. H. Jeong, S. S. Yi, K. Jang and H. S. Lee, Photoluminescence Properties of CeO2:Eu3+ Nanoparticles Synthesized by a Sol-Gel Method, J. Phys. Chem. C, 2009, 113, 610–617 CrossRef CAS.
- A. K. Pal, S. Som and C. H. Lu, Synthesis and spectroscopic analysis of Sm3+ doped CeO2 ceramic powders for the application of white LEDs, Ceram. Int., 2018, 44, 18256–18263 CrossRef CAS.
- N. V. Hai, D. T. Hien, N. T. K. Linh, B. T. Hoan, N. M. Tu and H. N. Van, Blue-excited red emission of CeO2:Eu3+, Al3+ cubic phosphor: influence of Al3+ ion doping and Judd-Ofelt theory, J. Lumin., 2023, 263, 120047 CrossRef.
- C. R. Michel and A. H. Martínez-Preciado, CO sensor based on thick films of 3D hierarchical CeO2 architectures, Sens. Actuators, B, 2014, 197, 177–184 CrossRef CAS.
- G. Vimal, K. P. Mani, D. Alexander, P. R. Biju, N. V. Unnikrishnan, M. A. Ittyachen and C. Joseph, Facile synthesis of Sm3+/Eu3+ codoped CeO2 ultrafine nanocrystals and oxygen vacancy site dependent photoluminescence, Opt. Mater., 2015, 50, 220–228 CrossRef CAS.
- L. Sun, Y. Tan, D. Li, H. Du and D. Guo, Defects and symmetry influence on visible emission of Bi3+ co-doped CeO2:Eu3+ phosphor, Opt. Mater., 2019, 100, 109654 CrossRef.
- K. Y. Jung, J. C. Lee, D. S. Kim, B. K. Choi and W. J. Kang, Co-doping effect of monovalent alkali metals on optical properties of CeO2:Eu nanophosphor prepared by spray pyrolysis and application for preparing pearlescent pigments with red emission, J. Lumin., 2017, 192, 1313–1321 CrossRef CAS.
- S. Mahamuda, K. Swapna, M. Venkateswarlu, A. S. Rao, S. Shakya and G. V. Prakash, Spectral characterisation of Sm3+ ions doped oxy-fluoroborate glasses for visible orange luminescent applications, J. Lumin., 2014, 154, 410–424 CrossRef CAS.
- D. T. T. Dung, V. T. N. Minh, N. X. Truong, P. V. Huan, P. H. Vuong, N. D. Hung, B. T. Hoan, L. M. Tu and H. N. Van, Dual-mode green emission and temperature sensing properties of rare-earth-element-doped biphasic calcium phosphate composites, J. Alloys Compd., 2021, 871, 159483 CrossRef.
- G. Ouertani, M. Ferhi, K. Horchani-Naifer and M. Ferid, Effect of Sm3+ concentration and excitation wavelength on spectroscopic properties of GdPO4:Sm3+ phosphor, J. Alloys Compd., 2021, 885, 161178 CrossRef CAS.
- U. Rambabu and S. Han, Luminescence optimization with superior asymmetric ratio (red/orange) and color purity of MBO3:Eu3+@SiO2 (M = Y, Gd and Al) nano down-conversion phosphors, RSC Adv., 2013, 3, 1368–1379 RSC.
- H. T. Nam, P. D. Tam, N. V. Hai and H. N. Van, Multifunctional optical thermometry using dual-mode green emission of CaZrO3:Er/Yb/Mo perovskite phosphors, RSC Adv., 2023, 13, 14660–14674 RSC.
- P. Singh, H. Mishra, P. C. Pandey and S. B. Rai, Structure, photoluminescence properties, and energy transfer phenomenon in Sm3+/Eu3+ co-doped CaTiO3 phosphors, New J. Chem., 2022, 47, 1460–1471 RSC.
- H. Duan, R. Cui, M. Zhang and C. Deng, Photoluminescence properties and energy transfer studies of Ba2YAlO5: Sm3+, Eu3+ orange-red phosphors, Optik, 2021, 238, 166774 CrossRef CAS.
- J. Zheng, X. Wu, Q. Ren, W. Bai, Y. Ren, M. Wang and O. Hai, Investigation of luminescence properties and energy transfer in Sm3+ and Eu3+ co-doped Sr3Y(BO3)3 red phosphors, Opt. Laser Technol., 2019, 122, 105857 CrossRef.
- H. Tang, X. Zhang, L. Cheng, L. Jiang, X. Mi, Q. Liu, J. Xie and Y. Wang, Photoluminescence properties and energy transfer mechanisms of Na4CaSi3O9: Sm3+, Eu3+ novel orange-red phosphors, J. Lumin., 2019, 214, 116532 CrossRef CAS.
- B. Su, H. Xie, Y. Tan, Y. Zhao, Q. Yang and S. Zhang, Luminescent properties, energy transfer, and thermal stability of double perovskites La2MgTiO6:Sm3+, Eu3+, J. Lumin., 2018, 204, 457–463 CrossRef CAS.
- D. Geng, M. Shang, D. Yang, Y. Zhang, Z. Cheng and J. Lin, Tunable luminescence and energy transfer properties in KCaGd(PO4)2:Ln3+/Mn2+ (Ln = Tb, Dy, Eu, Tm; Ce, Tb/Dy) phosphors with high quantum efficiencies, J. Mater. Chem., 2012, 22, 23789 RSC.
- M. Shang, G. Li, X. Kang, D. Yang, D. Geng and J. Lin, Tunable Luminescence and Energy Transfer Properties of Sr3AlO4F:RE3+ (RE = Tm/Tb, Eu, Ce) Phosphors, ACS Appl. Mater. Interfaces, 2011, 7, 2738–2746 CrossRef PubMed.
- R. B. Basavaraja, D. Navami, N. H. Deepthi, M. Venkataravanappa, R. Lokesh, K. H. S. Kumar and T. K. Sreelakshmi, Novel orange-red emitting Pr3+ doped CeO2 nanopowders for white light emitting diode applications, Inorg. Chem. Commun., 2020, 120, 108164 CrossRef.
- E. F. Huerta, O. S. Romero, A. N. Meza-Rocha, S. Bordignon, A. Speghini and U. Caldino, Lithium-aluminum-zinc phosphate glasses activated with Sm3+, Sm3+/Eu3+ and Sm3+/Tb3+ for reddish-orange and white light generation, J. Alloys Compd., 2020, 846, 156332 CrossRef CAS.
- Q. Ren, B. Wang, X. Wu, T. Wei and Z. Huo, Luminescence properties and energy transfer in Dy3+ and Eu3+ co-doped Sr3Y(PO4)3 phosphor, J. Alloys Compd., 2016, 684, 677–682 CrossRef CAS.
|
This journal is © The Royal Society of Chemistry 2023 |