DOI:
10.1039/D3RA06727K
(Paper)
RSC Adv., 2023,
13, 32104-32109
High precision measurements of lithium isotopic composition at sub-nanogram by MC-ICP-MS with membrane desolvation
Received
3rd October 2023
, Accepted 27th October 2023
First published on 1st November 2023
Abstract
The geochemistry of Li and Li isotopes is a promising tracer of chemical weathering processes for both modern and ancient times. Therefore, accurate and precise determination of the isotopic composition of Li is required for a large variety of complex geological samples with different Li concentrations and matrix/Li ratios. Especially, geochemical studies of precious geological samples with ultra-low lithium content and high matrix. In this study, the accuracy and the precision corresponding to Li isotopic measurements of low-level samples using multi-collector inductively coupled plasma mass spectrometry (MC-ICP-MS) with membrane desolvation introduction system was evaluated. The method of MC-ICP-MS with membrane desolvation and a high-sensitivity X-skimmer cone, together with a simple one-step column separation enabled the high-precision isotopic analysis of Li quantities as small as 2 ng. The long-term instrumental external reproducibility of δ7Li values for the L-SVEC and SPEX-Li were 0.0 ± 0.1‰ (n = 20) and 12.1 ± 0.4‰ (n = 20), respectively. Based on the measurements on a series of international reference materials over the last two years. The measured δ7Li values for the standards with a variety of matrices, including BHVO-2, AGV-2 and seawater (NASS-6). The δ7Li values of BHVO-2 (4.58 ± 0.35‰), AGV-2 (6.85 ± 0.40‰) and NASS-6 (30.88 ± 0.20‰) are in agreement with the published data within the uncertainty. We also present analytical results for South China Sea surface seawater water, meteorite, limestones and rain water. These results demonstrate the validity of the method for obtaining highly precise and accurate outcomes.
1. Introduction
Lithium (Li) is the third lightest chemical element and its atomic number is 3. Li is the lightest alkali metal and the lightest lithophile element. Li has two naturally occurring isotopes, 6Li (7.52%) and 7Li (92.48%).1 A relatively large mass difference (17%) between the two isotopes and high volatility results in significant mass-dependent fractionation during geochemical processes. The range of variation in lithium-isotope compositions (δ7Li) is more than 60‰ in geological materials.1 Besides, Li is present only in the +1 valence, its isotopic composition is not influenced by redox reactions. Also, Li is not a nutrient and does not participate in biologically mediated reactions and Li isotopes variations are not significantly affected by biological processes. These characteristics make Li isotopes have numerous applications in geochemistry, isotope hydrology, oceanography, environmental sciences, cosmology, and nuclear technology.2,3
Consequently, it is quite essential to conduct high-precision and accurate Li isotopic measurements on geological samples. The isotopic composition of Li can be analyzed by numerous mass spectrometric techniques, e.g., positive ion thermal ionization mass spectrometry (PTIMS), multi-collector inductively coupled plasma mass (MC-ICPMS), inductively coupled plasma source mass spectrometry (ICPMS), and secondary ion mass spectrometry (SIMS).1 Li isotopes were initially performed by PTIMS.4 However, it is only since the advent of the MC-ICP-MS that lithium isotopic analyses have become sufficiently precise for a variety of earth and ocean-science applications.5,6 The MC-ICP-MS technique quickly became a typical approach for determination of Li isotopic compositions in natural samples due to its higher ionization efficiency.7–11
Precise and accurate determination of Li isotope compositions in geological samples is crucial to expanding the application of Li isotopes. Nevertheless, geochemical studies of precious geological samples with ultra-low lithium content and high matrix, such as Lunar soil, meteorite and ocean drilling samples using Li isotopes are limited due to the matrix effects and instrumental sensitivity. To minimize matrix induced mass bias effects, high recovery and complete separation of Li from matrix elements are essential. As is known, the ion intensity can be improved by 3–10-fold with a desolvating nebuliser (i.e., dry plasma). Most Li isotope data in the literature are measured using new generation MC-ICP-MS coupled with the desolvation system to achieve a higher sensitivity too.12 However, systematic research has not yet been conducted to evaluate the influence of membrane desolvation injection system on lithium isotopes. In this study, High precision measurements of lithium isotopic composition by MC-ICP-MS with membrane desolvation introduction system was evaluated. Such as, the sensitivity of different Li concentrations with membrane desolvation introduction system, accuracy and precision of Li isotope measurements with different Li concentrations using membrane desolvation introduction system, etc.
2. Experimental
Chemical purification and isotopic analysis for Li were carried out at Institute of Earth Environment, Chinese Academy of Sciences (IEECAS) in a class-1000 ultraclean laboratory equipped with class-100 laminar flow exhaust hoods to minimize contamination.
2.1. Reagents and materials
Bio-Rad® AG 50W X-12 cationic resin was used in this study for Li purification. Electronic production of HNO3 and HCl (Suzhou Jingrui Chemical Co., Ltd.) were purified by double sub-boiling using a Savillex DST-1000 system at a temperature <60 °C. The ultrapure grade HF (48%) was directly used without further distillation.
Geological certified reference materials samples: a NIST L-SVEC lithium carbonate standard, Seawater reference material for trace metals (NASS-6), basalt powdered reference materials (BHVO-2) and andesite powdered reference materials (AGV-2).
Natural samples: surface seawater water (collected from South China Sea, 80 kilometers from Sanya bay of Hainan, China), meteorite (collected in the desert, 100 kilometers south of Hami city of Xinjiang, China), limestones (collected from the Meishan section in South China, as the Global Strato type Section and Point (GSSP) of the PTB), and rain water (collected from Xi'an of China) were used in this study.
2.2. Sample pretreatment
2.2.1 Sample digestion for rock and meteorite. To reduce the effects of procedural blanks and sample heterogeneity, 1–5 mg of each rock standard and samples (containing 2 to 10 ng of Li) were weighed into a pre-cleaned 15 mL of Teflon microwave digestion vessel. Next, 4 mL of HNO3 and 1 mL of HF were added. Samples were digested using an UltraClave microwave digestion system (Milestone, Italy) following the method of He et al.13 After digestion, the sample solutions were evaporated to dryness on a hot plate at 180 °C. Then, 1.2 mL of aqua regia was added to re-dissolve the samples, which were heated for 4 h at 180 °C. Following this step, the solution was dried again and re-dissolved in 10 mL of 2% v/v HNO3. This solution was dried and finally re-dissolved in 1 mL of 0.5 M HNO3 for ion exchange chromatography.
2.2.2 Sample pretreatment for rain water and seawater. The rain water and seawater containing approximately 2 ng Li, were evaporated to dryness on a hot plate at 180 °C. The final dried sample was dissolved in 2 mL 0.5 M HNO3 for column separation.
2.2.3 Sample pretreatment for limestones. Sample dissolution protocols for Li isotope analysis in limestone following Kalderon-Asael et al.14
2.3. Ion exchange chromatography for Li chemical separation
The Li chemical separation is carried out using a simple one-step ion-exchange chromatography with a procedure following the same method as described in He et al.13,15 Briefly, Li is purified by passing of 8 mL of Bio-Rad® AG 50W X-12 cationic resin in 0.5 mol L−1 HNO3 medium. The precleaning procedure of the resin batch consisted of six-fold washing with concentrated HCl (6 mol L−1) and rinsing with ultrapure water before column packing. The resin was conditioned for the separation with 16 mL of 0.5 M HNO3 before loading the sample. Subsequently, 2 mL of the 0.5 mol L−1 HNO3 sample was loaded on the resin. After the sample was loaded, 0.5 mol L−1 HNO3 was used to elute the sample, with the first 20 mL of eluate discarded. Then, the 21st−22nd mL of eluate was collected in a 5 mL centrifuge tube to monitor Li breakthrough. Then, the following 25 mL (23rd–47th) of eluate was collected as the Li fraction, and the 48th−49th mL was also used to check the recovery. The details of protocol are given in Table 1.
Table 1 Lithium purification procedure
Separation steps |
Eluents |
Volume/mL |
Resin cleaning |
6 M HNO3 |
16 (2 × 8 mL) |
Milli-Q H2O |
8 (1 × 8 mL) |
Conditioning |
0.5 M HNO3 |
16 (2 × 8 mL) |
Sample loading |
0.5 M HNO3 |
2 (1 × 2 mL) |
Elution |
0.5 M HNO3 |
20 (5 × 4 mL) |
Pre-cut collection |
0.5 M HNO3 |
2 (1 × 2 mL) |
Li elution |
0.5 M HNO3 |
25 (5 × 5 mL) |
After-cut collection |
0.5 M HNO3 |
2 (1 × 2 mL) |
Resin cleaning |
6 M HNO3 |
16 (2 × 8 mL) |
|
Milli-Q H2O |
8 (1 × 8 mL) |
2.4. Mass spectrometry of Li isotopes
The isotopic ratios of Li were performed on a Thermo Fisher Neptune Plus MC-ICP-MS also at the IEECAS. This instrument is a double-focusing magnetic sector instrument with variable dispersion (17%) ion optics equipped with nine collectors (Faraday cups). Faraday noise and pre-amplifier gain calibration were performed every analytical day, and ESA deflection (20 V) was used to measure the zero value.
Firstly, Sample solutions were introduced under solution mode (‘wet’ plasma conditions) using a low-flow PFA microcentric (50 μL min−1) nebulizer and a cyclone/double pass spray chamber. The nickel Jet type sample cone and X type skimmer cone were used. The lens voltages, carrier gas, and all parameters were optimized in solution mode. All solutions were in 2% (v/v) HNO3. Then, membrane desolvation introduction system was switched solution mode. Measurements were performed using an Apex Omega (ESI®, USA) sample introduction system with a heated spray chamber set at 140 °C and a Peltier cooling coil at 2 °C. Lithium isotopic ratios were determined using the standard-sample bracketing (SSB) protocol. The operating parameters are provided in Table 2. 6Li and 7Li were measured simultaneously in separate Faraday cups (L4 and H4).
Table 2 Typical operating parameters for Li isotopes measurement using Neptune MC-ICP-MS with membrane desolvation
Parameters |
Values |
RF forward power (W) |
1200 |
Ar cooling gas (L min−1) |
15 |
Ar auxiliary gas (L min−1) |
0.76 |
Ar sample gas (L min−1) |
1.081 |
Extraction voltage (V) |
−2000 |
Acceleration voltage (kV) |
10 |
Sampler cone |
X (nickel) |
Skimmer cone |
Jet (nickel) |
Detection system |
L4, H4 Faraday cups |
Nebulizer |
Low-flow PFA microcentric (50 μL min−1) |
Focus quad (V) |
0.5 |
Dispersion (V) |
26.5 |
Uptake time (s) |
60 |
Integration time (s) |
4.195 |
Measurement time (min) |
4 |
Rinse time (min) |
4 |
Membrane desolvation introduction system |
Apex Omega (ESI®, USA) |
Spray chamber (heater/chiller) |
140 °C/2 °C |
The Li isotopic composition is reported in delta (δ) notation in per mil relative to natural reference material L-SVEC following previous recommendation:2
3. Results and discussion
3.1. Sensitivity of different Li concentrations with membrane desolvation introduction system
Previous studies indicate that different concentrations have different signal sensitivity. The 7Li intensities of SPEX-Li solutions containing 10, 8, 5, 3, 2 and 1 ng Li were determined with desolvation membrane introduction system. The intensity of 7Li ion beam for these different Li concentrations are shown in Fig. 1. The signal intensities of 7Li were 6 V, 4.8 V, 3 V, 1.8 V, 1.2 V and 0.8 for the [Li] as 10, 8, 5, 3, 2 and 1 ng. Generally, to avoid noise disturbance, background intensity should be <1% of sample signal for isotopic analysis. By washing the inlet system via 5% HNO3 and 0.1% HF and then 2% HNO3, the instrumental background intensities of 7Li were lower as 0.008 V.
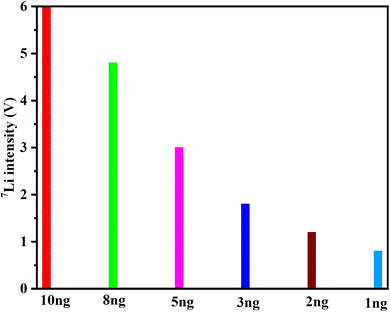 |
| Fig. 1 The integrated average signal intensity of 7Li from Li standard solution (SPEX-Li) with different contents. | |
3.2. Accuracy and precision of Li isotope measurements with different Li concentrations using membrane desolvation introduction system
A series of SPEX-Li containing 100, 50, 20, 10, 8, 5, 2, 1 ng Li was analyzed using “wet plasma” and “Dry plasma (with membrane desolvation)”, respectively. And the results are shown in Fig. 2. Repeated analyses of 100 ng and 50 ng Li had δ7Li values were 12.05 ± 0.06‰ (n = 4) and 12.00 ± 0.16‰ (n = 4). The results from the analyses of 20, 10, 8, 5, 2, 1 ng Li with membrane desolvation were consistent with those of 100 ng and 50 ng Li using “wet plasma”. And δ7Li values were 11.89 ± 0.08‰, 12.01 ± 0.08‰, 12.15 ± 0.06‰, 12.14 ± 0.14‰, 12.12 ± 0.30‰ and 12.40 ± 0.16‰.
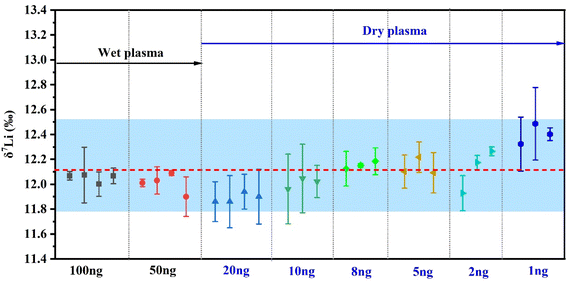 |
| Fig. 2 The δ7Li measured for SPEX-Li with sample sizes of 100 ng and 50 ng [Li] using “Wet plasma” and 20 ng, 10 ng, 8 ng, 5 ng, 3 ng, 2 ng, 1 ng [Li] using “Dry plasma”. | |
3.3. Accuracy and reproducibility of Li isotope measurements for standards
The accuracy and reproducibility of Li isotope measurements was evaluated by repeated analyses of the two standards (L-SVEC and SPEX-Li) with 2 ng [Li] over a one-year period. And the results are shown in Fig. 3. The long-term instrumental external reproducibility of δ7Li for these samples is <0.5‰ (2SD). The measured δ7Li values for the L-SVEC and SPEX-Li were 0.0 ± 0.1‰ (n = 20) and 12.1 ± 0.4‰ (n = 20), respectively. Therefore, high-precision Li isotopes determinations can be obtained using our MC-ICP-MS with membrane desolvation.
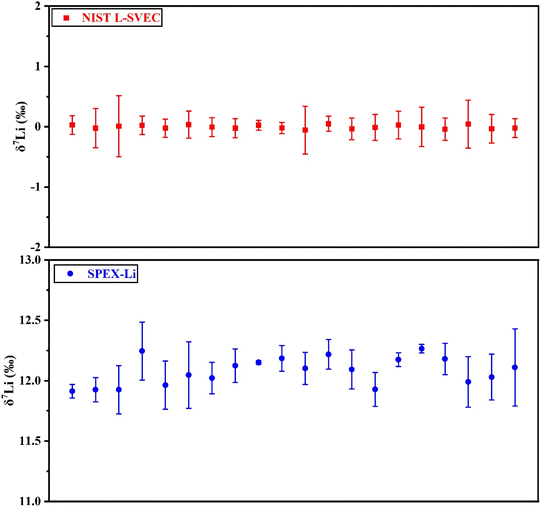 |
| Fig. 3 Evaluation of external precision (2SD) of δ7Li by 20 analyses over one year for NIST L-SVEC and SPEX-Li. Error bars are 2SD uncertainties represents the precision of this study. | |
3.4. Li isotopic compositions of reference materials
Based on more than 1 year repeated measurement of unpurified and purified L-SVEC, and SPEX-Li with 2 ng [Li] (Fig. 3), two geological reference materials BHVO-2, AGV-2 and seawater reference material NASS-6 with 2 ng [Li] were measured to confirmed the accuracy of δ7Li. Data for measurements of reference materials in this study and literature are plotted in Fig. 4. The δ7Li values of BHVO-2 (4.58 ± 0.35‰), AGV-2 (6.85 ± 0.40‰) and seawater (30.88 ± 0.20‰) are in agreement with the published data within the uncertainty (Fig. 4).3,11,15–22
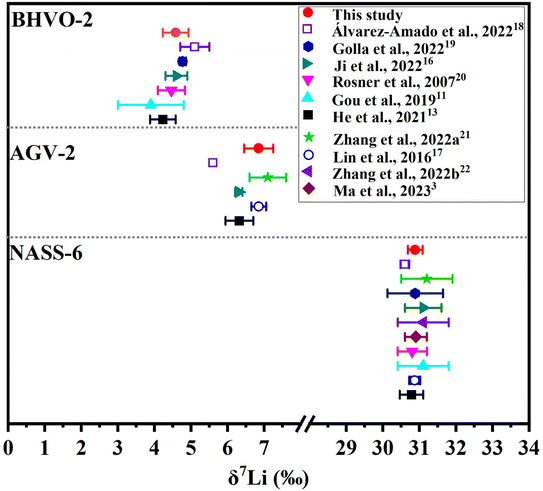 |
| Fig. 4 Comparison of δ7Li values of selected geological reference materials measured in this study and literature. Precision is plotted as 2SD for comparison. | |
3.5. Li isotope measurements of natural samples
3.5.1 Seawater δ7Li. In the open ocean, Li is a conservative element that is well-mixed with a uniform concentration and isotopic composition. In the ocean, due to its long residence time >1.5 My relative to the mixing time of the ocean (1000 years), modern seawater is homogenous with respect to lithium concentration (26 mM) and isotopic composition lithium displays uniform concentration isotopic composition (δ7Li = 31.0 ± 0.5‰).23,24 Additionally, seawater is commonly used as an interlaboratory standard. Therefore, a more precise definition of δ7Lisw is desirable.0.2 mL South China Sea surface water was conducted with AG 50W X-12 cationic resin. Li isotope ratios were investigated by MC-ICP-MS. The average (n = 5) δ7Lisw values was 30.97 ± 0.24‰. The average δ7Lisw value of seawater is generally understood to be 30.8‰, with reported values ranging from 30.60 to 31.20‰ (Fig. 4).
3.5.2 δ7Li of rain water. Rainwater is one of important end members, however, the lithium content is relatively low with less than 1.0 ppb. There rainwater samples from Xi'an in China were collected and analyzed. The δ7Li values of these samples were 8.53 ± 0.14‰ (n = 3), 10.19 ± 0.04‰ (n = 3) and 8.51 ± 0.23‰ (n = 3), respectively.
3.5.3 δ7Li of limestones. Limestones is one of useful archive for reconstruct seawater Li isotope compositions. Two samples from different time were processed with AG 50W X-12 cationic resin. The δ7Li values of these two samples were 9.93 ± 0.21‰ (n = 3) and 14.49 ± 0.12‰ (n = 3).
3.5.4 δ7Li of meteorite. Lithium isotope ratios were investigated by MC-ICP-MS using membrane desolvation on two meteorites. The measured δ7Li values of these two samples were −1.5 ± 0.39‰ (n = 4) and 6.41 ± 0.32‰ (n = 4), showing huge isotope fractionation of Li.
4. Conclusions
High precision (<0.5‰ at the 2 s level) Li isotope determinations in small amounts of Li (2 ng) were achieved using a combination of MC-ICP-MS and a membrane desolvation system. The accuracy and reproducibility of Li isotope measurements were assessed through repeated analyses of the two standards (L-SVEC and SPEX-Li) with 2 ng [Li] over a one-year timeframe. The long-term instrumental external reproducibility of δ7Li for these samples was found to be less than 0.5‰ (2SD). The measured δ7Li values for the L-SVEC and SPEX-Li standards were determined to be 0.0 ± 0.1‰ (n = 20) and 12.1 ± 0.4‰ (n = 20), respectively. Additionally, three reference materials (BHVO-2, AGV-2 and NASS-6) were analyzed and displayed mean δ7Li values of 4.58 ± 0.35‰ (2 s, n = 6), 6.85 ± 0.40‰ (2 s, n = 6) and 30.88 ± 0.20‰ (2 s, n = 6). The same protocol was then applied to samples with very low sample amounts, such as South China Sea surface seawater water, meteorite, limestones and rain water. This method allows for precise (≤0.6‰) and accurate determination of δ7Li in sub-nanogram samples.
Data availability
Data will be made available on request.
Author contributions
All the authors have participated in experiments, writing, discussion and editing the manuscript.
Conflicts of interest
The authors declare that they have no known competing financial interests or personal relationships that could have appeared to influence the work reported in this paper.
Acknowledgements
This work was supported by the National Natural Science Foundation of China (No. 42130206, 42173023), the Strategic Priority Research Program of Chinese Academy of Sciences (XDB40000000), Shaanxi Provincial Natural Science Foundation for Distinguished Young Scholars (2022JC-16), and The State Key Laboratory of Loess and Quaternary Geology (SKLLQGPY2101).
References
- F. Z. Teng, J. Watkins and N. Dauphas, Non-Traditional Stable Isotopes, 2017, vol. 82, pp. 1–26 Search PubMed.
- T. B. Coplen, J. A. Hopple, J. K. Böhlke, H. S. Peiser, S. E. Rieder, H. R. Krouse, K. J. R. Rosman, T. Ding, R. D. Vocke Jr, K. M. Révész, A. Lamberty, P. Taylor and P. De Bièvre, U.S. Geological Survey, Water-Resources Investigations, 2002, Report 01, p. 4222 Search PubMed.
- T. Ma, M. Weynell, S. L. Li, J. Zhong, S. Xu and C. Q. Liu, Sci. Total Environ., 2023, 879, 163214 CrossRef CAS PubMed.
- E. Michiels and P. De Bièvre, Int. J. Mass Spectrom. Ion Phys., 1983, 49, 265–274 CrossRef CAS.
- T. Magna, U. H. Wiechert and A. N. Halliday, Mass Spectrom., 2004, 239, 67–76 CAS.
- P. B. Tomascak, F. Tera, R. T. Helz and R. J. Walker, Geochim. Cosmochim. Acta, 1999, 63, 907–910 CrossRef CAS.
- K. F. Huang, C. F. You, Y. H. Liu, R. M. Wang, P. Y. Lin and C. H. Chung, J. Anal. At. Spectrom., 2010, 25, 1019–1024 RSC.
- Y. Hu and F. Z. Teng, J. Anal. At. Spectrom., 2019, 34, 338–346 RSC.
- X. M. Liu and W. Li, J. Anal. At. Spectrom., 2019, 34, 1708–1717 RSC.
- S. Juzer, N. Tanwar and S. Misra, J. Anal. At. Spectrom., 2022, 37, 1541–1553 RSC.
- L. F. Gou, Z. D. Jin, L. Deng, M. Y. He and C. Y. Liu, Spectrochim. Acta, Part B, 2018, 146, 1–8 CrossRef CAS.
- A. B. Jeffcoate, T. Elliott, A. Thomas and C. Bouman, Geostand. Geoanal. Res., 2004, 28, 161–172 CrossRef CAS.
- M. Y. He, C. G. Luo, H. Lu, Z. D. Jin and L. Deng, J. Anal. At. Spectrom., 2019, 34, 1773–1778 RSC.
- B. Kalderon-Asael, J. A. R. Katchinoff, N. J. Planavsky, A. V. S. Hood, M. Dellinger, E. J. Bellefroid, D. S. Jones, A. Hofmann, F. O. Ossa, F. A. Macdonald, C. Wang, T. T. Isson, J. G. Murphy, J. A. Higgins, A. J. West, M. W. Wallace, D. Asael and P. A. E. Pogge von Strandmann, Nature, 2021, 595, 394–398 CrossRef CAS PubMed.
- M. Y. He, J. B. Dong, Z. D. Jin, C. Y. Liu, J. Xiao, F. Zhang, H. Sun, Z. Q. Zhao, L. F. Gou, W. G. Liu, C. G. Luo, Y. G. Song, L. Ma and L. Deng, Geochim. Cosmochim. Acta, 2021, 299, 151–162 CrossRef CAS.
- T. T. Ji, X. W. Jiang, L. F. Gou, Z. D. Jin, H. Zhang, L. Wan, G. L. Han, H. M. Guo and X. S. Wang, Geochim. Cosmochim. Acta, 2022, 326, 313–327 CrossRef CAS.
- J. Lin, Y. Liu, Z. Hu, L. Yang, K. Chen, H. Chen, K. Zong and S. Gao, J. Anal. At. Spectrom., 2016, 31, 390–397 RSC.
- F. Álvarez-Amado, M. Rosales, L. Godfrey, C. Poblete-González, E. Morgado, M. Espinoza, A. Hidalgo-Gajardo, D. Volosky and J. Cortés-Aranda, J. Geochem. Explor., 2022, 241, 107062 CrossRef.
- J. K. Golla, J. Bouchez, M. L. Kuessner, D. M. Rempe and J. L. Druhan, Earth Planet. Sci. Lett., 2022, 595, 117773 CrossRef CAS.
- M. Rosner, L. Ball, B. Peucker-Ehrenbrink, J. Blusztajn, W. Bach and J. Erzinger, Geostand. Geoanal. Res., 2007, 31, 77–88 CrossRef CAS.
- F. Zhang, M. Dellinger, R. G. Hilton, J. Yu, M. B. Allen, A. L. Densmore, H. Sun and Z. D. Jin, Nat. Commun., 2022, 13, 1–10 CAS.
- J. W. Zhang, Y. N. Yan, Z. Q. Zhao, X. M. Liu, X. D. Li, D. Zhang, H. Ding, J. L. Meng and C. Q. Liu, Earth Planet. Sci. Lett., 2022, 600, 117875 CrossRef CAS.
- L. Bastian, N. Vigier, S. Reynaud, M. E. Kerros, M. Revel and G. Bayon, Geostand. Geoanal. Res., 2018, 42, 403–415 CrossRef CAS.
- J. Roberts, K. Kaczmarek, G. Langer, L. C. Skinner, J. Bijma, H. Bradbury, A. V. Turchyn, F. Lamy and S. Misra, Geochim. Cosmochim. Acta, 2018, 236, 336–350 CrossRef CAS.
|
This journal is © The Royal Society of Chemistry 2023 |