DOI:
10.1039/D0QO01097A
(Review Article)
Org. Chem. Front., 2020,
7, 3941-3955
Ni-Catalyzed stereoselective difunctionalization of alkynes
Received
9th September 2020
, Accepted 13th October 2020
First published on 13th October 2020
Abstract
Tri- and tetrasubstituted olefins are widely present in biologically active molecules and functional materials. However, the methods for the stereoselective construction of such moieties are still very limited and extremely challenging. The transition-metal-catalyzed difunctionalization of alkynes is one of the most straightforward and effective choices. In this review, we summarize the progress of the nickel-catalyzed alkyne difunctionalization reaction, with an emphasis on the strategy and control of stereochemistry.
1. Introduction
Tri- and tetra-substituted alkenes are widely present in many biologically and pharmacologically active molecules, including acutiphycin, epothilone A, tamoxifen, isovirescenol A, brasilenol and guadalupol (Scheme 1).1 However, the methods for the stereoselective construction of these unique motifs are still very limited and extremely challenging.2
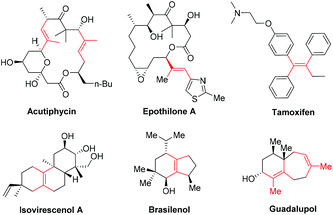 |
| Scheme 1 Representative biologically active compounds containing a tri- or tetrasubstituted alkene moiety. | |
Alkynes are readily available and inexpensive raw materials. Transition-metal-catalyzed difunctionalization of alkynes which is the syn- or anti-selective introduction of two functional groups across the triple bond represents one of the most straightforward and powerful approaches for assembling stereodefined tri- and tetrasubstituted alkenes. Traditionally used rare noble metal catalysts based on Rh, Ir and Pd have been widely used in alkyne difunctionalization reactions.3 However, they are very expensive and their reserves are declining, thus limiting their applications in large-scale industrial processes. To develop more sustainable catalytic methods to produce chemicals, much attention has been directed to seek first row transition metals that are Earth-abundant and sustainable to replace these highly expensive and rare metals in alkyne difunctionalization reactions. In the past few decades, although tremendous progress has been made in the nickel-catalyzed difunctionalization of alkynes, there is still a lack of comprehensive discussion on stereoselectivity control.4 In this review, we summarize the progress of the nickel-catalyzed alkyne difunctionalization reaction for the construction of multisubstituted alkenes, with an emphasis on the strategy and control of stereochemistry. Since the hydrofunctionalization of alkynes has been well reviewed, we will not discuss it in this paper.5
2. Ni-Catalyzed syn-difunctionalization of alkynes
The Ni-catalyzed syn-selective difunctionalization of alkynes has been well exploited, in which two functional groups are installed on the same side of the double bond in the products. As shown in Scheme 2, four effective strategies have been successfully developed. The most common strategy is to utilize the nature of syn-migratory insertion of an alkyne into organonickel species to form alkenyl-nickel intermediate 1, which can undergo reductive elimination or further functionalization to provide a reliable route to multi-substituted alkenes (Scheme 2a). The migratory insertion of alkynes into a nickelacycle intermediate, followed by reductive elimination, is also a common method for the syn-selective difunctionalization of alkynes (Scheme 2b). Another powerful strategy is the coupling reaction of alkynes with π-unsaturated compounds, such as aldehydes, enones, and imines, which involves the formation of a five-membered nickelacycle intermediate 2 through oxidative cyclization (Scheme 2c). In addition, the merging of photoredox and nickel catalysis is emerging as an alternative strategy to access multi-substituted alkenes in a syn-selective manner (Scheme 2d).
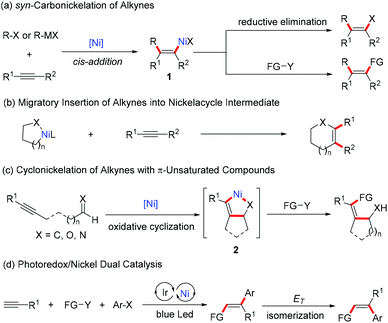 |
| Scheme 2 Strategies for syn-selective difunctionalization of alkynes. | |
2.1
syn-Carbonickelation of alkynes
The arylcyanation reaction of alkynes involves the direct cleavage and addition of strong aryl–CN bonds across the carbon–carbon triple bond, and represents a high atom-economic and efficient route for the preparation of alkenyl nitriles. In 2004, Hiyama and co-workers demonstrated the first example of the Ni-catalyzed arylcyanation of alkynes for the synthesis of β-aryl-substituted alkenyl nitriles (Scheme 3).6 The electron-deficient benzonitriles can react efficiently to give alkenyl nitriles in excellent yields. However, the arylcyanation reaction of alkynes using electron-rich benzonitriles is generally sluggish.
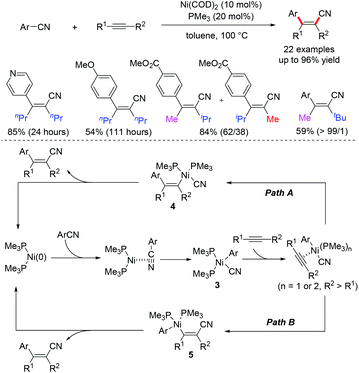 |
| Scheme 3 Ni-Catalyzed arylcyanation of alkynes. | |
Two possible mechanisms were considered for this transformation. The oxidative addition of Ar–CN to Ni(0) followed by the migratory insertion of alkynes into the resulting Ar–Ni(II)–CN species 3 at the Ar–Ni bond affords an alkenylnickel intermediate 4, which undergoes reductive elimination to give the arylcyanation products and regenerate the active Ni(0) catalyst (path A). Alternatively, the possibility of migratory insertion of alkyne into the Ar–Ni(II)–CN species 3 at the CN–Ni bond to form alkenylnickel intermediate 5 cannot be ruled out (path B). Interestingly, when asymmetric alkynes were examined, the reaction preferably produced the arylcyanation product in which the aryl group is remote from the bulky iso-propyl (62/38) or tert-butyl group (>99/1). These results indicate that the migratory insertion of alkynes into the Ni–Ar bond is feasible, and the nickel center prefers to be far away from the bulky groups due to steric hindrance.
Further theoretical studies by DFT calculation also validate that path A is more favourable because it is much more difficult to achieve migratory insertion of alkyne into the Ni–CN bond than the Ni–Ar bond.7
The same group further developed the carbocyanation of alkynes with the aid of nickel–Lewis acid dual catalysis, in which the Lewis acid co-catalyst was thought to activate the electrophilic CN center. With this strategy, a wide array of nitriles not only electron-rich benzonitriles, but also alkenyl, alkynyl, benzyl, allyl, alkyl, as well as pentafluorobenzyl nitriles were demonstrated to be compatible with this alkyne carbocyanation reaction (Scheme 4).8
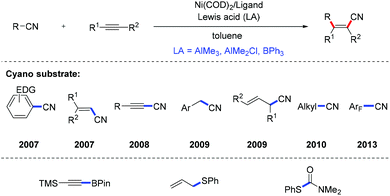 |
| Scheme 4 Ni-Catalyzed carbocyanation, alkynylboration, thioallylation and thiocarbamoylation of alkynes. | |
Ni-Catalyzed intermolecular alkynylboration,9 thioallylation,10 and thiocarbamoylation11 of alkynes were also developed for the efficient synthesis of alkenylborons and alkenysulfides (Scheme 4), which are versatile synthetic intermediates in organic synthesis.
The three-component redox neutral difunctionalization of alkynes is also a very effective method for constructing multi-substituted olefins by simultaneously introducing nucleophiles and electrophiles across the triple bonds. In 2015, Hayashi and co-workers reported a Ni-catalyzed cross coupling of alkynes with aryl Grignard reagents and aryl halides, providing an operationally simple method for synthesizing tetrasubstituted alkenes with high stereo- and regioselectivity (Scheme 5).12
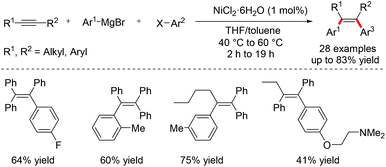 |
| Scheme 5 Ni-Catalyzed three-component coupling of aryl Grignard reagents, alkynes and aryl halides. | |
Xi13 and Cheng14 independently reported the nickel-catalyzed regioselective arylcarboxylation of alkynes with arylmagnesium reagents and carbon dioxide (CO2, 1 atm) for the synthesis of trisubstituted β-arylacrylic acids (Scheme 6). One possible mechanism is proposed in Scheme 6. The oxidative cycloaddition reaction of Ni(0) with alkyne affords the Ni(II) complex 6, which undergoes transmetalation with the Grignard reagent to generate alkenyl-Ni(II) complex 7. The reductive elimination of 7 followed by the addition of CO2 and hydrolysis will deliver the desired β-arylacrylic acids.
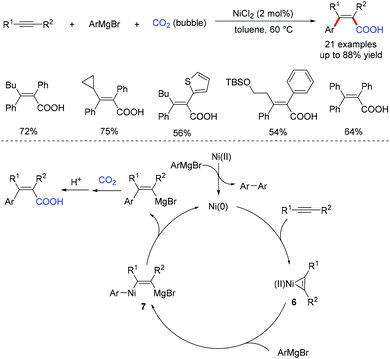 |
| Scheme 6 Ni-Catalyzed arylcarboxylation of alkynes. | |
Following Hayashi15 and Murakami's pioneering studies16 on the Rh-catalyzed cyclization of o-formyl aryl boronic acids with alkynes, Lam reported the Ni-catalyzed cyclization reaction of 1-phenyl-1-butyne with 2-formylphenylboronic acid using (S,Sp)-tBu-phosferrox (L1) as the chiral ligand, giving the indenol product in 81% yield with 87% ee (Scheme 7).17 The indene skeleton was constructed via syn-arylnickelation of the alkyne followed by nucleophilic addition of the resulting alkenylnickel species onto the aldehyde.
 |
| Scheme 7 Ni-Catalyzed cyclization of 2-formylphenylboronic acid with alkyne. | |
Our group reported a nickel-catalyzed cascade cyclization of enynones 8 for the modular synthesis of bridged tricyclo[5.2.1.01,5]decanes 9 with three quaternary stereocenters in good yields and excellent enantioselectivities (92–99% ee).18 A possible catalytic cycle is proposed in Scheme 8. The syn-selective addition of arylnickel species to the triple bond formed the alkenylnickel intermediate 10. Intramolecular migratory insertion of alkenylnickel 10 to the double bond followed by nucleophilic cyclization onto one of the ketone groups affords the tricyclo[5.2.1.01,5]decane product 9 upon hydrolysis (Scheme 8).
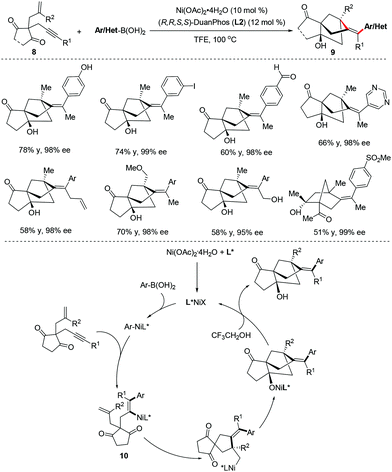 |
| Scheme 8 Ni-Catalyzed enantioselective cyclization of enynones. | |
The reductive difunctionalization of alkynes has also been developed, which involves the simultaneous incorporation of two electrophiles to both sides of the triple bond without the use of preformed organometallic reagents. In 2002, Cheng et al. disclosed a Ni-catalyzed intermolecular reductive carbonickelation of o-halophenyl ketones with alkynes using Zn powder as a reducing agent, providing an efficient route to functionalized indenol derivatives (Scheme 9).19 The oxidative addition of aryl halides to nickel(0) species, followed by the migratory insertion of alkynes into the Ni–Ar bond affords alkenylnickel intermediate 11. Intramolecular nucleophilic addition of alkenylnickel 11 to the carbonyl, followed by transmetalation with zinc halide, delivers the indenol product upon hydrolysis. In addition, the same strategy has also been applied to the preparation of substituted quinolines20 and (iso)quinolines.21
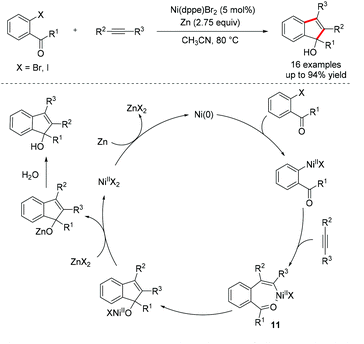 |
| Scheme 9 Ni-Catalyzed reductive carbocyclization of alkynes with o-halophenyl ketones. | |
Maddaluno and co-workers described a Ni-catalyzed reductive arylfunctionalization of alkynes (Scheme 10).22 Remarkably, the nucleophilic alkenylnickel species 12 resulting from the intramolecular arylnickelation of the triple bond could be trapped by a variety of electrophiles, such as benzaldehyde, nonanal, benzyl chloride, allyl acetate, and α-chloroesters, providing a convenient method for the synthesis of substituted benzofurans and chromanes.
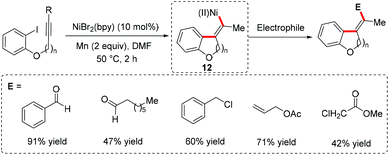 |
| Scheme 10 Ni-Catalyzed reductive arylfunctionalization of alkynes. | |
Recently, our group had also reported a Ni-catalyzed highly regio- and enantioselective reductive cyclization reaction of acrylamides with asymmetric internal alkynes.23 This transformation takes place under mild conditions with high efficiency, providing rapid access to structurally diverse cyclopentannulated indolines in good yields with high regioselectivity (>20/1) and enantioselectivities (27 examples, 82–96% ee) (Scheme 11). A mechanistic study showed that the cyclopentannulated indolines are afforded through a highly regioselective migratory insertion of asymmetric internal alkynes into the σ-alkyl-Ni(II) species 13, followed by nucleophilic addition of the resulting alkenyl nickel to unactivated amides.
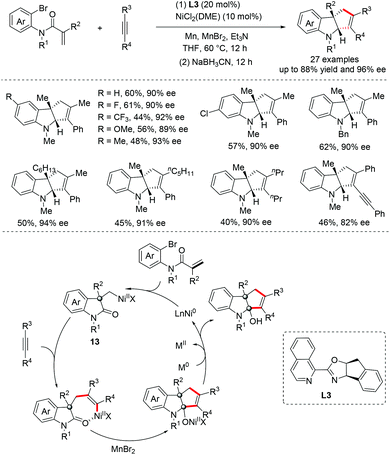 |
| Scheme 11 Ni-Catalyzed enantioselective reductive cyclization of alkynes with arylbromide-tethered acrylamides. | |
2.2 Migratory insertion of alkynes into the nickelacycle intermediate
Another method for the syn-difunctionalization of alkynes is to replace a part of the cyclic compounds with low-valent nickel while eliminating small molecules such as CO or CO2 to form the nickelacycle intermediate 14 followed by migratory insertion of alkynes. Matsubara and co-workers initiated the study of Ni-catalyzed decarbonylative cycloaddition of anhydrides to alkynes for the synthesis of isocoumarins (Scheme 12).24 As shown in Scheme 12, a series of heterocycles could be effectively constructed by this strategy.25
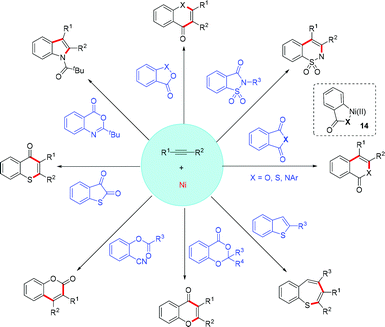 |
| Scheme 12 Ni-Catalyzed cycloaddition reaction of unsaturated compounds with alkynes. | |
Matsubara and co-workers reported a Ni-catalyzed cycloaddition between α,β-unsaturated carbonyl compounds with alkynes.26 Mechanistic studies have shown that the reaction proceeded through the oxidative cyclization of Ni(0) and enone to form an oxa-nickelacycle intermediate 15, followed by migratory insertion of alkyne (Scheme 13).
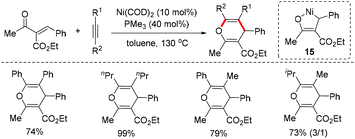 |
| Scheme 13 Nickel-catalyzed cycloaddition of enone with alkynes. | |
2.3 Cyclonickelation of alkynes with π-unsaturated compounds
Ni-Catalyzed reductive coupling of alkynes and aldehydes, pioneered by the group of Sato,27 Montgomery,28 and Jamison,29 is an attractive and general approach for the synthesis of stereodefined allylic alcohols bearing tri- and tetrasubstituted alkenes.2d,30
The Montgomery group demonstrated the first example of Ni-catalyzed cyclization/alkylation of alkynals with organozinc reagents to form cyclic allylic alcohols and the three-component coupling of aldehydes, organozincs and alkynes to synthesize acyclic allylic alcohols with complete control of alkene stereochemistry (Scheme 14).31 The oxidative cyclization of Ni(0) with aldehydes and alkynes affords a common oxa-nickelacycle intermediate 18. Transmetalation of species 18 with an organozinc reagent gives the alkenylnickel species 19, followed by reductive elimination to deliver the alkylated product 17. Alternatively, in the presence of a catalytic PPh3 ligand, β-hydride elimination occurs prior to reductive elimination, therefore resulting in the reductive product 16.
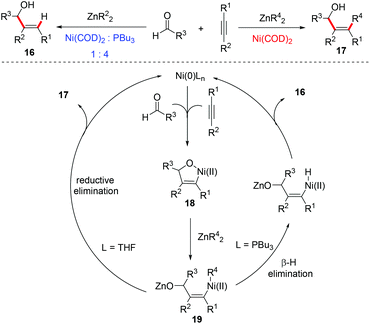 |
| Scheme 14 Ni-Catalyzed reductive coupling of alkynes and aldehydes. | |
The reductive coupling reaction of alkynes with other unsaturated carbonyl compounds, such as enones,28a,32 carbon dioxide,33 imines,29c and epoxides,34 have also been successfully developed for the synthesis of various functionalized tetrasubstituted alkenes (Scheme 15). This Ni-catalyzed multi-component coupling of alkynes has been applied to the total synthesis of many biologically active natural products, such as isodomoic acid G,35 isogeissoschizoid,36 and erythrocarine37 (Scheme 15).
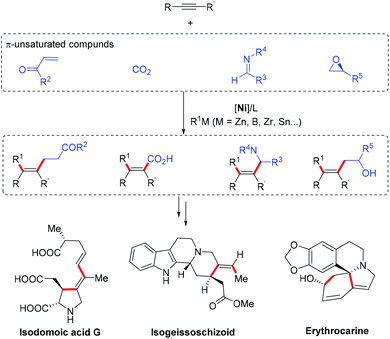 |
| Scheme 15 Ni-Catalyzed reductive coupling of alkynes and various π-unsaturated compounds and synthetic applications. | |
Asymmetric versions of the multi-component coupling of alkynes have been exploited. The Jamison group realized the asymmetric three-component reductive coupling of alkynes, Et3B and imines using a P-chiral ferrocenyl phosphane ligand L4, providing an efficient way to enantiomerically enriched tetrasubstituted allylic amines (Scheme 16a).38 Zhou and co-workers developed an Ni-catalyzed asymmetric alkylative coupling reaction of alkynes, aldehydes and ZnMe2 using a spiro phosphoramidite ligand L5 (Scheme 16b).39 In addition, Tang's group used their own independently developed P-chiral phosphorus ligand L6 to further explore this reaction (Scheme 16c).40 The asymmetric transformation provides an efficient way to prepare chiral allylic alcohols bearing tetrasubstituted olefin functionality.
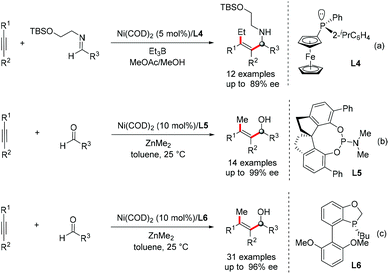 |
| Scheme 16 Ni-Catalyzed asymmetric coupling of alkynes with imines or aldehydes. | |
Recently, Montgomery et al. combined the Ni-catalyzed oxidative cyclization of alkynals and reductive cross-electrophile coupling together as a new approach for the synthesis of tetrasubstituted alkenes (Scheme 17).41 The reaction employed alkyl halide as an alkylating reagent and obviated the requirement for preformed organometallic reagents, greatly improving the functional group compatibility compared to the classic coupling method. The oxidative cyclization of nickel(0) with alkynals affords the nickelacycle intermediate 20. The nickel–oxygen bond is cleaved by Et3SiCl to form the silyl protected alkenyl nickel(II) intermediate 21, which is reduced by Mn(0) to generate the alkenyl Ni(I) species 22. The oxidative addition of alkyl halide followed by reductive elimination delivers the desired products along with Ni(I) species, which undergoes further Mn-mediated reduction to regenerate the active Ni(0) catalyst (Scheme 17).
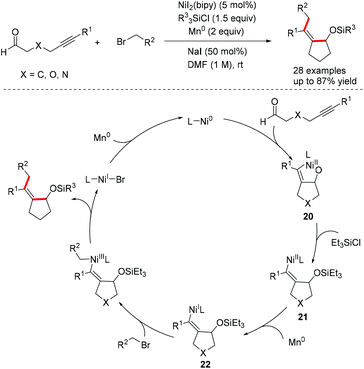 |
| Scheme 17 Ni-Catalyzed reductive coupling of alkynals with alkyl halides. | |
The same group further developed a Ni-catalyzed [3 + 2] reductive cycloaddition of alkynes with enals using Ni(COD)2/PBu3 as the catalyst and Et3B as the reductant. This method provides a novel strategy for the assembly of five-membered carbocyclic rings. Both inter- and intramolecular variants of the process could proceed smoothly to provide an array of cyclopentenol derivatives (Scheme 18a).42
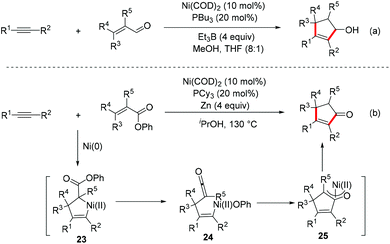 |
| Scheme 18 Ni-Catalyzed reductive [3 + 2] cycloaddition of alkynes with α,β-unsaturated carbonyl compounds. | |
In addition, a Ni-catalyzed [3 + 2] cycloaddition reaction of α,β-unsaturated phenyl esters with alkyne in iPrOH using Zn powder as the reductant was also demonstrated by Ohashi and co-workers (Scheme 18b).43 A possible mechanism involving C–O bond activation was proposed. The nickelacyclopentene intermediate 23 was formed through the oxidative cyclization of α,β-unsaturated phenyl ester with alkyne and nickel(0). β-Phenoxy elimination followed by insertion of the resulting ketene intermediate 24 into the C–Ni bond provides η3-oxaallylnickel species 25, which undergoes alcoholysis to deliver the cyclopentenone product.
2.4 Ni/photoredox dual catalysis
Photoredox catalysis has been studied and can be used to promote the contra-thermodynamic E/Z isomerization of olefins through energy-transfer. Chu et al. reported an intermolecular syn-selective alkylarylation of terminal alkynes with tertiary alkyl oxalates and aryl bromides via photoredox-nickel dual catalysis.44Scheme 19 explains the mechanism of syn-selectivity. Single-electron transfer between photoexcited Ir(III) and tertiary alkyl oxalate is expected to generate the tertiary alkyl radical and Ir(II). Addition of the resulting alkyl radical to terminal alkyne gives alkenyl radical 27. An anti-addition of alkenyl radical 27 to Ni(0) leads to the (E)-alkenyl-Ni(I) species 28. The oxidative addition with aryl bromide, followed by reductive elimination gives E-substituted alkenes 29. Finally, a photochemical E/Z isomerization of the resulting E-alkenes through the energy transfer process will deliver the desired Z-alkene 30.
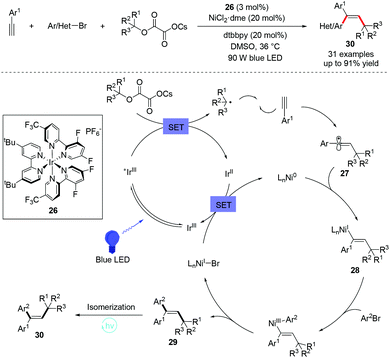 |
| Scheme 19 Ni/photoredox dual catalyzed syn-selective alkylarylation of terminal alkynes. | |
Very recently, Rueping et al. further developed a three-component cross-coupling reaction of alkynes, aryl halides and sodium sulfinates via a photoredox/nickel dual catalysis, enabling one-pot access to alkenyl sulfoxides under mild reaction conditions (Scheme 20, top).45 In addition, a similar arylalkylation of alkynes was also reported.46 The protocols possess a broad substrate scope and good functional-group tolerance, albeit with moderate stereoselectivity (syn-addition manner) (Scheme 20, bottom). Both these transformations proceed via a mechanism involving a single-electron transfer with a subsequent energy-transfer activation pathway.
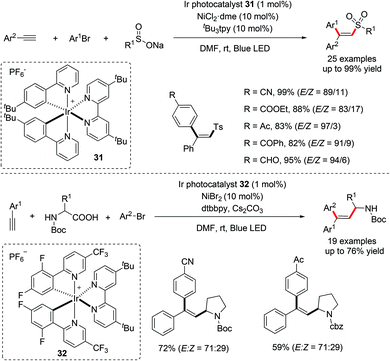 |
| Scheme 20 Ni/photoredox dual-catalyzed arylsulfonylation and arylalkylation of alkynes. | |
3. Ni-Catalyzed anti-difunctionalization of alkynes
Compared with the well-developed syn-selective difunctionalization of alkynes, the Ni-catalyzed anti-selective alkyne difunctionalization reaction is still uncommon. One effective strategy to obtain formal anti-difunctionalized products is electrophile triggered cyclization, which employs alkyne substrates bearing a heteroatom-containing substituent at the adjacent position. After activation of the triple bond by nickel coordination, an intramolecular nucleophilic addition to the alkyne forms the trans-alkenylnickel species 33 (Scheme 21a). Alternatively, Ni-catalyzed radical addition/coupling reaction of terminal alkynes is another important method to provide anti-difunctionalized products (Scheme 21b). In addition, although the carbonickelation of alkynes usually undergoes in a syn-selective manner, in some cases, due to the steric hindrance of the substrates or driven by the formation of more stable and chelated alkenyl-nickel species, E/Z isomerization of the resulting alkenyl-nickel species may take place (Scheme 21c).
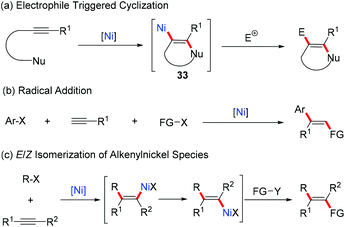 |
| Scheme 21 Strategies for anti-selective difunctionalization of alkynes. | |
3.1 Electrophile triggered cyclization
Although palladium-catalyzed electrophile triggered cyclization of alkynes bearing a hetero nucleophile has been well developed for the synthesis of a wide variety of heterocycles,47 the nickel catalyst was less employed as a catalytic precursor within this reactivity pattern. Recently, Dake et al. reported a nickel-catalyzed anti-arylamination of 2-alkynyl-N-sulfonylanilides to form 2,3-difunctionalized N-arylsulfonylindoles.48 A possible mechanism involving oxidative addition, alkyne amination and reductive elimination was proposed (Scheme 22).
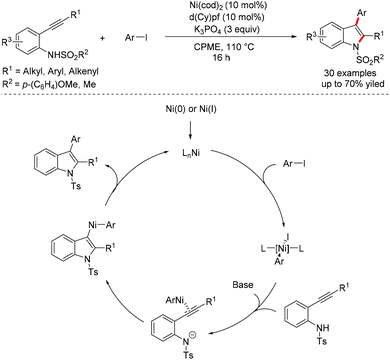 |
| Scheme 22 Ni-Catalyzed anti-selective arylamination of 2-alkynyl-N-sulfonylanilides. | |
3.2 Radical addition/coupling with terminal alkynes
In 2016, Nevado and co-workers disclosed a Ni-catalyzed three-component coupling reaction of terminal alkynes, alkyl halides and boronic acids for the stereoselective synthesis of trisubstituted alkenes (Scheme 23).49 The protocol, involving the simultaneous incorporation of both aryl and alkyl groups across the triple bond in a radical-mediated process, provided access to trisubstituted alkenes in a highly regio- and stereocontrolled manner. Significantly, both activated and unactivated alkyl halides were well tolerated under the optimized reaction conditions. The proposed mechanism starts with the generation of active Ni(I) species in situ and reacts with organoboron reagents through transmetalation. The resulting Ar–Ni(I) species is responsible for the activation of alkyl halides to produce an alkyl radical along with the Ar–Ni(II) intermediate 34. The alkyl radical then undergoes highly selective addition to the terminal alkyne to deliver vinyl radical intermediate 35. The subsequent recombination of the vinyl radical intermediate 35 with Ar–Ni(II) 34 will afford the key Ni(III) intermediate 36, which undergoes reductive elimination to furnish the desired products while regenerating the active Ni(I) catalyst (Scheme 23).
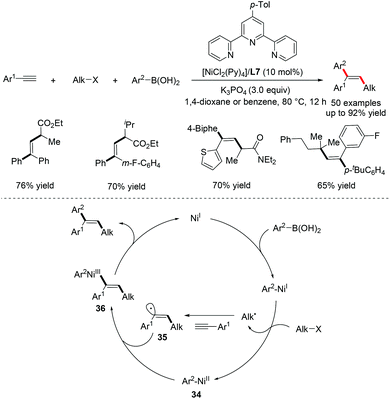 |
| Scheme 23 Ni-Catalyzed anti-selective arylalkylation of terminal alkynes. | |
The same group further reported a similar stereoselective carbosulfonylation of terminal alkynes by utilizing sulfonyl chlorides as radical precursors, enabling the rapid synthesis of β,β-disubstituted vinyl sulfones with broad functional group compatibility (Scheme 24).50
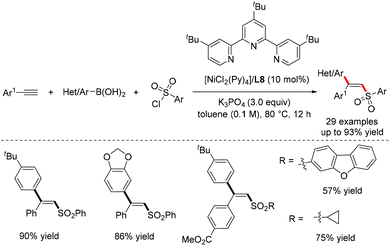 |
| Scheme 24 Ni-Catalyzed anti-selective carbosulfonylation of terminal alkynes. | |
3.3
E/Z isomerization of alkenylnickel species
3.3.1
E/Z isomerization driven by steric hindrance of substrates.
In 2005, Suginome's group reported a nickel-catalyzed trans-carboboration of chloroboryl homopropargylic ethers with organotin regents, which provided a new way for the stereoselective synthesis of highly functionalized organoboron compounds (Scheme 25).51 A possible mechanism was put forward to elucidate the observed trans-addition mode. The oxidative addition of the B–Cl bond to nickel, followed by intramolecular cis-migratory insertion of alkyne into the B–Ni bond resulted in cis-alkenylnickel intermediate 37. It was speculated that the considerable steric repulsion caused by the diisopropylamino group and the chlorobis-(triphenylphosphine)nickel moiety forced the isomerization of cis-alkenylnickel to trans-alkenylnickel 38. Delivery of the alkynyl group from the organotin reagent via the transmetalation step and the subsequent reductive elimination would afford trans-alkynylboration products (Scheme 25).
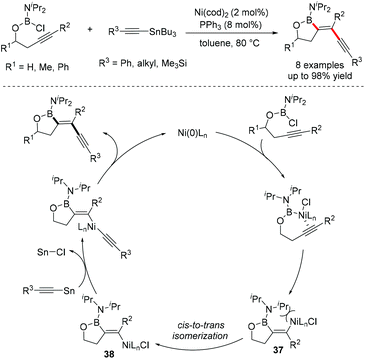 |
| Scheme 25 Ni-Catalyzed anti-carboboration of alkynes. | |
Inspired by the dramatic impact of steric factors on promoting the isomerization of alkenyl nickel species, an unprecedented anti-carbocyanation of 1,6-enynes under nickel catalysis was realized by the Arai group (Scheme 26).52 The catalytic protocol was triggered by hydronickelation of alkenes followed by carbonickelation of alkynes. Mechanism studies have shown that bulky substituents such as TIPS or TMS are essential for causing steric repulsion after enyne cyclization, and this effect would control the geometry of the C–C double bond through a key nickel–carbene intermediate.
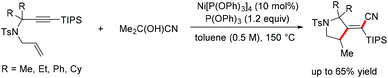 |
| Scheme 26 Ni-Catalyzed anti-carbocyanation of 1,6-enynes. | |
Martin and co-workers reported a Ni-catalyzed reductive cyclization/carbonylation of unactivated alkyl halides with carbon dioxide (Scheme 27).53 This robust protocol fused the CO2 fixation with a cascade reductive process, resulting in carbonylated carbocyclic products with a divergent syn/anti selectivity pattern modulated by substrates. Specifically, the cis-selective cyclization/carboxylation products were exclusively produced as preliminary alkyl halides were used, however, the trans-selectivity was favoured with bulk substituents at the α-position of alkyl bromides.
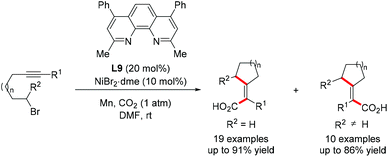 |
| Scheme 27 Ni-Catalyzed divergent cyclization/carboxylation of unactivated alkyl bromides with CO2. | |
Similar effects have been demonstrated in Montgomery's work on three-component reaction of aldehydes, alkynes, and alkyl halides, in which the Z isomer of alkylative cyclization products predominated when secondary alkyl bromides were employed (Scheme 28).41 The result of this Z/E isomerization may be driven by the crowded steric environment of the vinylnickel intermediate. Significantly, the reaction concentration also has an extraordinary effect on the E/Z selectivity, where the proportion of the Z isomer of the product increases as the reaction concentration decreases.
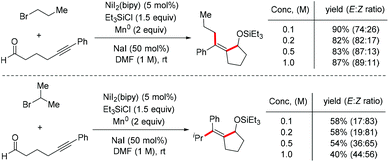 |
| Scheme 28 Ni-Catalyzed oxidative cyclization and reductive cross electrophile coupling of aldehydes, alkynes, and alkyl halides. | |
3.3.2
E/Z isomerization driven by the formation of chelated alkenyl-nickel species.
In 2016, Liu et al. disclosed a nickel-catalyzed addition/cyclization of alkyne-nitriles with organoboronic acids, enabling the construction of highly functionalized 1-naphthylamines (Scheme 29).54 Remarkably, aryl boronic acids bearing a wide range of diverse substitutes underwent the cyclization smoothly to afford the desired 1-naphthylamines in moderate to high yields. On the basis of mechanistic studies, the author proposed a catalytic cycle triggered by Ni(I) species, which was generated by the disproportionation reaction of Ni(0) and Ni(II). Transmetalation of Ni(I) species with arylboronic acid leading to an arylnickel(I) complex, followed by migratory insertion of the carbon–carbon triple bond into Ar–Ni(I) species affords an alkenylnickel(I) intermediate 39. The tethered cyano group may play a role in facilitating the cis–trans isomerization by stabilizing the alkenylnickel(I) species. An intramolecular nucleophilic addition of the alkenylnickel(I) 39 to the cyano group, followed by protonation and tautomerization, will furnish 1-naphthylamines and regenerate the active Ni(I) catalyst (Scheme 29).
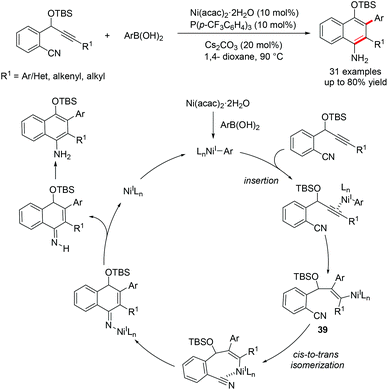 |
| Scheme 29 Ni-Catalyzed anti-addition/cyclization of alkyne-nitriles with organoboronic acids. | |
Lam and co-workers independently reported a Ni-catalyzed enantioselective anti-arylative cyclization of alkynones and organoboronic acids (Scheme 30).17 By using the (R)-Ph-Phox as a ligand, a wide variety of alkynones or cyclohexa-1,3-dienones are compatible with this transformation and exhibit excellent enantioselectivities.
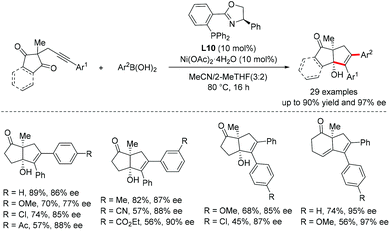 |
| Scheme 30 Ni-Catalyzed enantioselective anti-arylative cyclization of alkynyl electrophiles with organoboronic acids. | |
Our group recently developed a new catalyst system for the anti-arylative cyclization of alkynones and aryl halides through a reductive cross-coupling strategy.55 The transformation proceeds smoothly in the absence of organometallic reagents and features high functional group tolerance, providing an effective platform to access a wide variety of synthetically useful endo-cyclic tetrasubstituted allylic alcohols in a stereoselective manner (Scheme 31). A possible reaction mechanism for the reductive arylative cyclization of alkynone was proposed. The oxidative addition of ArX into Ni(0) followed by migratory insertion of alkyne into the resulting arylnickel(II) species affords an alkenyl-Ni(II) intermediate 40. A reversible E/Z isomerization process takes place to produce a new alkenyl-Ni(II) intermediate 41, which could be reduced by Mn0 to give more nucleophilic alkenyl-Ni(I) species 42. Nucleophilic addition of alkenyl-Ni(I) 42 to the ketone followed by protonolysis produced the endo-cyclic tetrasubstituted allylic alcohols. The catalytically active Ni(0) species was then regenerated upon Mn0 reduction (path B, Scheme 31). The direct cyclization of the alkenyl-Ni(II) intermediate 41 to the ketone carbonyl cannot be excluded (path A, Scheme 31).
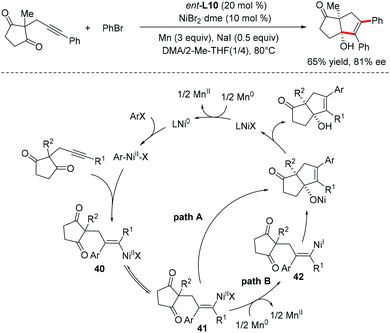 |
| Scheme 31 Ni-Catalyzed enantioselective anti-arylative cyclization of alkynones with aryl halides. | |
Our group further demonstrated the Ni-catalyzed reductive coupling of unsymmetrical internal alkynes, which is still a significant challenge in organic synthesis (Scheme 32).56 Both self-coupling and cross-coupling versions of reductive coupling of two unsymmetrical internal alkynes were achieved using a hemilabile directing group strategy, enabling rapidly access to a series of synthetically challenging pentasubstituted 1,3-dienes with diverse functional groups in good yields with high regio- and enantioselectivity (mostly >20/1 rr, >90% ee). Compared to traditional cross-coupling reactions, the reaction features high atom- and step-economy, without requiring the use of prepared stereodefined coupling partners such as vinyl halides or vinyl organometallics. A rationalized mechanism for this transformation was proposed based on the mechanistic studies. Initially, a catalytically active Ni(0) species was formed upon the reduction of the Ni(II) precatalyst by Zn dust. Oxidative cyclization of alkynone 43 with another unsymmetrical internal alkyne 44 gave a nickelacycle 46, in which the tethered carbonyl or hydroxyl group serves as a hemilabile directing group to control the regioselectivity. Then, selective protonation of 46 by alcohol would afford the conjugated dienylnickel species 47, which could undergo a reversible cis–trans isomerization to produce a new dienylnickel intermediate 48. Finally, the target pentasubstituted 1,3-diene 45 was formed through intramolecular nucleophilic attack of the dienylnickel 48 to carbonyls and the subsequent protonation.
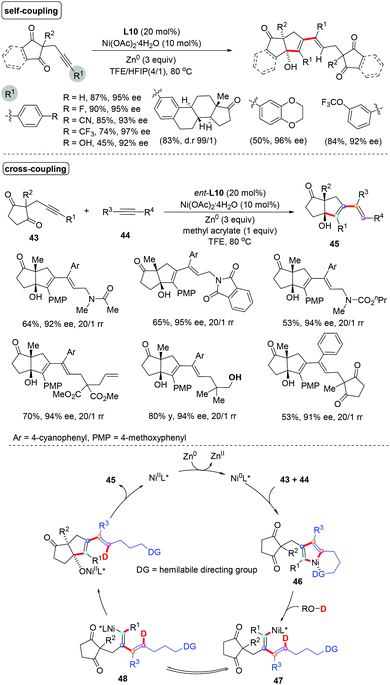 |
| Scheme 32 Ni-Catalyzed reductive coupling of unsymmetrical internal alkynes. | |
Several other types of electrophiles were demonstrated to be suitable for this nickel-catalyzed anti-carbonickelation cyclization reaction. In 2017, the Lam group developed highly enantioselective allylic alkenylations of Z-allylic phosphate tethered alkynes which provide a range of chiral 1,4-diene-containing carbo- and heterocycles (Scheme 33a).57 In 2018, the same group further developed a nickel-catalyzed desymmetrization of malonate esters for the enantioselective synthesis of highly functionalized cyclopent-2-enones, and the cyclization is enabled by the reversible E/Z isomerization of alkenylnickel species (Scheme 33b).58 Later, the less electrophilic N-Ts-amides were proved to effectively capture the alkenylnickel species to obtain multisubstituted pyrroles (Scheme 33c).59 Trapping the alkenylnickel intermediate by an azide group, a non-carbon center electrophile has also been developed for the efficient synthesis of 2,3-diarylquinolines (Scheme 33d).60
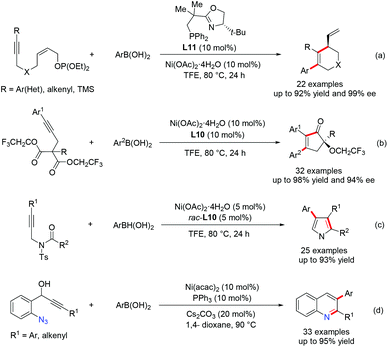 |
| Scheme 33 Ni-Catalyzed cyclization of alkynyl electrophiles with organoboronic acids involving anti-carbonickelation of alkynes. | |
Ni-Catalyzed desymmetrization of alkyne-tethered malononitriles with aryl boronic acids was developed by Liu group (Scheme 34).61 This protocol involves the cis-addition of aryl boronic acids to alkynes, followed by E/Z isomerization of alkenylnickel species and selective nitrile insertion, providing unprecedented access to 5–7-membered skeletons bearing a nitrile-containing quaternary stereocenter in good yields with excellent enantioselectivities.
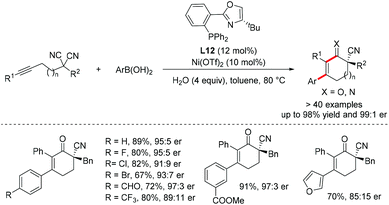 |
| Scheme 34 Ni-Catalyzed enantioselective anti-arylative cyclization of alkyne-tethered malononitriles. | |
4. Conclusions
In this review, we summarize the recent advances in the nickel-catalyzed stereoselective alkyne difunctionalization reaction. Different strategies for controlling stereoselectivity towards the synthesis of stereodefined tri- and tetrasubstituted alkenes have been highlighted. Although considerable progress has been made in this rapidly evolving field in the past few decades, many intriguing challenges still lie ahead to extend the use of these methodologies.
Firstly, most of these methods require the use of stoichiometric organometallic regents, which impose limitations in their synthetic applicability. The stereoselective difunctionalization of alkynes via the reductive cross-coupling strategy would be more advantageous, but there are very minimal successful examples in this area.
Secondly, the stereoselective difunctionalization of alkynes is still tailored for a specific substrate class. In particular, the anti-difunctionalization of alkynes by the radical-mediated process is restricted to terminal alkynes.
Thirdly, the detailed reaction mechanism for controlling stereoselectivity is still indistinct in many transformations. Thus more effort needs to be focused on the detailed mechanistic studies, as it will be conducive for designing and developing innovative catalytic systems.
Conflicts of interest
There are no conflicts to declare.
Acknowledgements
We acknowledged financial support from the “1000-Youth Talents Plan”, Wuhan University and National Natural Science Foundation of China (No. 21702149).
Notes and references
-
(a) F. C. Engelhardt, Y. J. Shi, C. J. Cowden, D. A. Conlon, B. Pipik, G. Zhou, J. M. McNamara and U. H. Dolling, Synthesis of a NO-releasing prodrug of rofecoxib, J. Org. Chem., 2006, 71, 480–491 CrossRef CAS;
(b) N. F. McKinley and D. F. O'Shea, Carbolithiation of diphenylacetylene as a stereoselective route to (Z)-tamoxifen and related tetrasubstituted olefins, J. Org. Chem., 2006, 71, 9552–9555 CrossRef CAS;
(c) J. Wang, Z. Dong, C. Yang and G. Dong, Modular and regioselective synthesis of all-carbon tetrasubstituted olefins enabled by an alkenyl Catellani reaction, Nat. Chem., 2019, 11, 1106–1112 CrossRef CAS;
(d) M. Li, T. Y. Yao, S. Z. Sun, T. X. Yan, L. R. Wen and L. B. Zhang, The ruthenium(II)-catalyzed C-H olefination of indoles with alkynes: the facile construction of tetrasubstituted alkenes under aqueous conditions, Org. Biomol. Chem., 2020, 18, 3158–3163 RSC.
-
(a) A. B. Flynn and W. W. Ogilvie, Stereocontrolled synthesis of tetrasubstituted olefins, Chem. Rev., 2007, 107, 4698–4745 CrossRef CAS;
(b) R. Chinchilla and C. Najera, Chemicals from alkynes with palladium catalysts, Chem. Rev., 2014, 114, 1783–1826 CrossRef CAS;
(c) H. Yoshida, Borylation of alkynes under base/coinage metal catalysis: some recent developments, ACS Catal., 2016, 6, 1799–1811 CrossRef CAS;
(d) R. M. Moslin, K. Miller-Moslin and T. F. Jamison, Regioselectivity and enantioselectivity in nickel-catalysed reductive coupling reactions of alkynes, Chem. Commun., 2007, 2007, 4441–4449 RSC.
- For selected reviews, see:
(a) G. Zeni and R. C. Larock, Synthesis of heterocycles via palladium π-olefin and π-alkyne chemistry, Chem. Rev., 2004, 104, 2285–2309 CrossRef CAS;
(b) E. Negishi, Z. Huang, G. Wang, S. Mohan, C. Wang and H. Hattori, Recent advances in efficient and selective synthesis of di-, tri-, and tetrasubstituted alkenes via Pd-catalyzed alkenylation–carbonyl olefination synergy, Acc. Chem. Res., 2008, 41, 1474–1485 CrossRef CAS;
(c) R. Dorel and A. M. Echavarren, Gold(I)-catalyzed activation of alkynes for the construction of molecular complexity, Chem. Rev., 2015, 115, 9028–9072 CrossRef CAS.
- When we were preparing the manuscript, a review article about Ni-catalyzed anti-selective alkyne functionalization reactions was published: S. E. Bottcher, L. E. Hutchinson and D. J. Wilger, Nickel-catalyzed anti-selective alkyne functionalization reactions, Synthesis, 2020, 52, 2807–2820 CrossRef CAS.
-
(a) F. Alonso, I. P. Beletskaya and M. Yus, Transition-metal-catalyzed addition of heteroatom-hydrogen bonds to alkynes, Chem. Rev., 2004, 104, 3079–3159 CrossRef CAS;
(b) R. Severin and S. Doye, The catalytic hydroamination of alkynes, Chem. Soc. Rev., 2007, 36, 1407–1420 RSC;
(c) V. P. Ananikov, A. V. Makarov and I. P. Beletskaya, Catalytic hydrofunctionalization of alkynes through P-H bond addition: the unique role of orientation and properties of the phosphorus group in the insertion step, Chem. – Eur. J., 2011, 17, 12623–12630 CrossRef CAS;
(d) X. Zeng, Recent advances in catalytic sequential reactions involving hydroelement addition to carbon-carbon multiple bonds, Chem. Rev., 2013, 113, 6864–6900 CrossRef CAS;
(e) G. Lalic and A. Suess, Copper-catalyzed hydrofunctionalization of alkynes, Synlett, 2016, 27, 1165–1174 CrossRef;
(f) Y. Zheng and W. Zi, Transition-metal catalyzed enantioselective hydrofunctionalization of alkynes, Tetrahedron Lett., 2018, 59, 2205–2213 CrossRef CAS;
(g) H. Wen, G. Liu and Z. Huang, Recent advances in tridentate iron and cobalt complexes for alkene and alkyne hydrofunctionalizations, Coord. Chem. Rev., 2019, 386, 138–153 CrossRef CAS.
- Y. Nakao, S. Oda and T. Hiyama, Nickel-catalyzed arylcyanation of alkynes, J. Am. Chem. Soc., 2004, 126, 13904–13905 CrossRef CAS.
- Y. Ohnishi, Y. Nakao, H. Sato, Y. Nakao, T. Hiyama and S. Sakaki, A theoretical study of nickel(0)-catalyzed phenylcyanation of alkynes. Reaction mechanism and regioselectivity, Organometallics, 2009, 28, 2583–2594 CrossRef CAS.
-
(a) Y. Nakao, T. Yukawa, Y. Hirata, S. Oda, J. Satoh and T. Hiyama, Allylcyanation of alkynes: regio- and stereoselective access to functionalized di- or trisubstituted acrylonitriles, J. Am. Chem. Soc., 2006, 128, 7116–7117 CrossRef CAS;
(b) Y. Nakao, A. Yada, S. Ebata and T. Hiyama, A dramatic effect of Lewis-acid catalysts on nickel-catalyzed carbocyanation of alkynes, J. Am. Chem. Soc., 2007, 129, 2428–2429 CrossRef CAS;
(c) Y. Nakao, Y. Hirata, M. Tanaka and T. Hiyama, Nickel/BPh3-catalyzed alkynylcyanation of alkynes and 1,2-dienes: an efficient route to highly functionalized conjugated enynes, Angew. Chem., Int. Ed., 2008, 47, 385–387 CrossRef CAS;
(d) Y. Nakao and T. Hiyama, Nickel-catalyzed carbocyanation of alkynes, Pure Appl. Chem., 2008, 80, 1097–1107 CAS;
(e) Y. Hirata, T. Yukawa, N. Kashihara, Y. Nakao and T. Hiyama, Nickel-catalyzed carbocyanation of alkynes with allyl cyanides, J. Am. Chem. Soc., 2009, 131, 10964–10973 CrossRef CAS;
(f) A. Yada, T. Yukawa, Y. Nakao and T. Hiyama, Nickel/AlMe2Cl-catalysed carbocyanation of alkynes using arylacetonitriles, Chem. Commun., 2009, 3931–3933 RSC;
(g) Y. Nakao, A. Yada and T. Hiyama, Heteroatom-directed alkylcyanation of alkynes, J. Am. Chem. Soc., 2010, 132, 10024–10026 CrossRef CAS;
(h) Y. Minami, H. Yoshiyasu, Y. Nakao and T. Hiyama, Highly chemoselective carbon-carbon σ-bond activation: nickel/Lewis acid catalysed polyfluoroarylcyanation of alkynes, Angew. Chem., Int. Ed., 2013, 52, 883–887 CrossRef CAS.
- M. Suginome, M. Shirakura and A. Yamamoto, Nickel-catalyzed addition of alkynylboranes to alkynes, J. Am. Chem. Soc., 2006, 128, 14438–14439 CrossRef CAS.
- R. Hua, H. Takeda, S. Y. Onozawa, Y. Abe and M. Tanaka, Nickel-catalyzed thioallylation of alkynes with allyl phenyl sulphides, Org. Lett., 2007, 9, 263–266 CrossRef CAS.
- T. Inami, T. Kurahashi and S. Matsubara, Nickel-catalysed synthesis of tetrasubstituted vinyl sulfides from thiocarbamates and internal alkynes, Chem. Commun., 2015, 51, 1285–1288 RSC.
- F. Xue, J. Zhao, T. S. Hor and T. Hayashi, Nickel-catalyzed three-component domino reactions of aryl Grignard reagents, alkynes, and aryl halides producing tetrasubstituted alkenes, J. Am. Chem. Soc., 2015, 137, 3189–3192 CrossRef CAS.
- S. Wang and C. Xi, Nickel-catalyzed arylative carboxylation of alkynes with arylmagnesium reagents and carbon dioxide leading to trisubstituted acrylic acids, Org. Lett., 2018, 20, 4131–4134 CrossRef CAS.
- C.-H. Hung, R. Santhoshkumar, Y.-C. Chang and C.-H. Cheng, Synthesis of trisubstituted acrylic acids through nickel-catalyzed carbomagnesiation of alkynes and carbon dioxide fixation, Eur. J. Org. Chem., 2018, 6924–6928 CrossRef CAS.
- R. Shintani, K. Okamoto and T. Hayashi, Rhodium-catalyzed synthesis of indenols by regioselective coupling of alkynes with ortho-carbonylated arylboronic acids, Chem. Lett., 2005, 34, 1294–1295 CrossRef CAS.
- T. Matsuda, M. Makino and M. Murakami, Synthesis of 1H-inden-1-ol derivatives via rhodium-catalyzed annulation of o-acylphenylboronic acids with alkynes, Chem. Lett., 2005, 34, 1416–1417 CrossRef CAS.
- C. Clarke, C. A. Incerti-Pradillos and H. W. Lam, Enantioselective nickel-catalyzed anti-carbometallative cyclizations of alkynyl electrophiles enabled by reversible alkenylnickel E/Z isomerization, J. Am. Chem. Soc., 2016, 138, 8068–8071 CrossRef CAS.
- J. Chen, Y. Wang, Z. Ding and W. Kong, Synthesis of bridged tricyclo[5.2.1.01,5]decanes via nickel-catalyzed asymmetric domino cyclization of enynones, Nat. Commun., 2020, 11, 1882 CrossRef CAS.
-
(a) D. K. Rayabarapu and C. H. Cheng, Nickel-catalyzed regioselective carbocyclization of ortho-halophenyl ketones with propiolates: an efficient route to disubstituted indenols, Chem. Commun., 2002, 942–943 RSC;
(b) D. K. Rayabarapu, C. H. Yang and C. H. Cheng, Regioselective synthesis of indenols via nickel-catalyzed carbocyclization reaction, J. Org. Chem., 2003, 68, 6726–6731 CrossRef CAS.
- R. P. Korivi and C. H. Cheng, Nickel-catalyzed cyclization of 2-iodoanilines with aroylalkynes: an efficient route for quinoline derivatives, J. Org. Chem., 2006, 71, 7079–7082 CrossRef CAS.
- R. P. Korivi and C. H. Cheng, Highly efficient synthesis of isoquinolines via nickel-catalyzed annulation of 2-iodobenzaldimines with alkynes: evidence for dual pathways of alkyne insertion, Org. Lett., 2005, 7, 5179–5182 CrossRef CAS.
-
(a) M. Durandetti, L. Hardou, M. Clement and J. Maddaluno, Heterocyclization by catalytic carbonickelation of alkynes: a domino sequence involving vinylnickels, Chem. Commun., 2009, 4753–4755 RSC;
(b) M. Durandetti, L. Hardou, R. Lhermet, M. Rouen and J. Maddaluno, Synthetic applications of the nickel-catalyzed cyclization of alkynes combined with addition reactions in a domino process, Chem. – Eur. J., 2011, 17, 12773–12783 CrossRef CAS.
- Y. Ping, K. Wang, Q. Pan, Z. Ding, Z. Zhou, Y. Guo and W. Kong, Ni-catalyzed regio- and enantioselective domino reductive cyclization: one-pot synthesis of 2,3-fused cyclopentannulated indolines, ACS Catal., 2019, 9, 7335–7342 CrossRef CAS.
- Y. Kajita, T. Kurahashi and S. Matsubara, Nickel-catalyzed decarbonylative addition of anhydrides to alkynes, J. Am. Chem. Soc., 2008, 130, 17226–17227 CrossRef CAS.
- For selected reviews, see:
(a) T. Kurahashi and S. Matsubara, Nickel-catalyzed reactions directed toward the formation of heterocycles, Acc. Chem. Res., 2015, 48, 1703–1716 CrossRef CAS. For selected examples, see:
(b) Y. Kajita, S. Matsubara and T. Kurahashi, Nickel-catalyzed decarbonylative addition of phthalimides to alkynes, J. Am. Chem. Soc., 2008, 130, 6058–6059 CrossRef CAS;
(c) A. Ooguri, K. Nakai, T. Kurahashi and S. Matsubara, Nickel-catalyzed cycloaddition of salicylic acid ketals to alkynes via elimination of ketones, J. Am. Chem. Soc., 2009, 131, 13194–13195 CrossRef CAS;
(d) N. Maizuru, T. Inami, T. Kurahashi and S. Matsubara, Nickel-catalyzed cycloaddition of anthranilic acid derivatives to alkynes, Org. Lett., 2011, 13, 1206–1209 CrossRef CAS;
(e) K. Nakai, T. Kurahashi and S. Matsubara, Nickel-catalyzed cycloaddition of o-arylcarboxybenzonitriles and alkynes via cleavage of two carbon-carbon σ bonds, J. Am. Chem. Soc., 2011, 133, 11066–11068 CrossRef CAS;
(f) T. Shiba, T. Kurahashi and S. Matsubara, Nickel-catalyzed decarbonylative alkylidenation of phthalimides with trimethylsilyl-substituted alkynes, J. Am. Chem. Soc., 2013, 135, 13636–13639 CrossRef CAS;
(g) W. Guan, S. Sakaki, T. Kurahashi and S. Matsubara, Reasons two nonstrained C–C σ-bonds can be easily cleaved in decyanative [4+2] cycloaddition catalyzed by nickel(0)/Lewis acid systems. theoretical insight, ACS Catal., 2014, 5, 1–10 Search PubMed;
(h) T. Inami, T. Kurahashi and S. Matsubara, Nickel-catalyzed reaction of thioisatins and alkynes: a facile synthesis of thiochromones, Org. Lett., 2014, 16, 5660–5662 CrossRef CAS;
(i) P. Mi, P. Liao, T. Tu and X. Bi, decarbonylative C-C bond-forming reactions of saccharins by nickel catalysis: homocoupling and cycloaddition, Chem. – Eur. J., 2015, 21, 5332–5336 CrossRef CAS;
(j) T. Inami, T. Takahashi, T. Kurahashi and S. Matsubara, Nickel-catalyzed [5+2] cycloaddition of 10π-electron aromatic benzothiophenes with alkynes to form thermally metastable 12π-electron nonaromatic benzothiepines, J. Am. Chem. Soc., 2019, 141, 12541–12544 CrossRef CAS;
(k) P. Mi, L. He, T. Shen, J. Z. Sun and H. Zhao, A novel fluorescent skeleton from disubstituted thiochromenones via nickel-catalyzed cycloaddition of sulfobenzoic anhydrides with alkynes, Org. Lett., 2019, 21, 6280–6284 CrossRef CAS.
- I. Koyama, T. Kurahashi and S. Matsubara, Nickel-catalyzed [4+2] cycloaddition of enones with alkynes, J. Am. Chem. Soc., 2009, 131, 1350–1351 CrossRef CAS.
-
(a) N. Saito, T. Katayama and Y. Sato, Nickel-catalyzed highly regioselective multicomponent coupling of ynamides, aldehydes, and silane: a new access to functionalized enamides, Org. Lett., 2008, 10, 3829–3832 CrossRef CAS;
(b) N. Saito, Y. Sugimura and Y. Sato, A facile construction of bi- or tricyclic skeletons by nickel-catalyzed stereoselective cyclization of alkynylcycloalkanone, Org. Lett., 2010, 12, 3494–3497 CrossRef CAS.
-
(a) J. Montgomery and A. V. Savchenko, Nickel-catalyzed cyclizations of alkynyl enones with concomitant stereoselective tri- or tetrasubstituted alkene introduction, J. Am. Chem. Soc., 1996, 118, 2099–2100 CrossRef CAS;
(b) X.-Q. Tang and J. Montgomery, Nickel catalysis in the stereoselective preparation of quinolizidine, pyrrolizidine, and indolizidine alkaloids: total synthesis of (+)-Allopumiliotoxin 267A, J. Am. Chem. Soc., 1999, 121, 6098–6099 CrossRef CAS;
(c) X. Qi and J. Montgomery, New three-component synthesis of 1,3-dienes employing nickel catalysis, J. Org. Chem., 1999, 64, 9310–9313 CrossRef CAS;
(d) M. Lozanov and J. Montgomery, A new two-step four-component synthesis of highly functionalized cyclohexenols by sequential nickel-catalyzed couplings, J. Am. Chem. Soc., 2002, 124, 2106–2107 CrossRef CAS;
(e) Y. Ni, K. K. Amarasinghe and J. Montgomery, Nickel-catalyzed cyclizations and couplings with vinylzirconium reagents, Org. Lett., 2002, 4, 1743–1745 CrossRef CAS.
-
(a) W.-S. Huang, J. Chan and T. F. Jamison, Highly selective catalytic intermolecular reductive coupling of alkynes and aldehydes, Org. Lett., 2000, 2, 4221–4223 CrossRef CAS;
(b) J. Chan and T. F. Jamison, Synthesis of (-)-terpestacin via catalytic, stereoselective fragment coupling: siccanol is terpestacin, not 11-epi-terpestacin, J. Am. Chem. Soc., 2003, 125, 11514–11515 CrossRef CAS;
(c) S. J. Patel and T. F. Jamison, Catalytic three-component coupling of alkynes, imines, and organoboron reagents, Angew. Chem., Int. Ed., 2003, 42, 1364–1367 CrossRef CAS;
(d) K. M. Miller, W. Huang and T. F. Jamison, Catalytic asymmetric reductive coupling of alkynes and aldehydes: enantioselective synthesis of allylic alcohols and α-hydroxy ketones, J. Am. Chem. Soc., 2003, 125, 3442–3443 CrossRef CAS;
(e) E. A. Colby, K. C. O'Brie and T. F. Jamison, Total syntheses of amphidinolides T1 and T4 via catalytic, stereoselective, reductive macrocyclizations, J. Am. Chem. Soc., 2005, 127, 4297–4307 CrossRef CAS.
- For selected reviews, see:
(a) S. Saito and Y. Yamamoto, Recent advances in the transition-metal-catalyzed regioselective approaches to polysubstituted benzene derivatives, Chem. Rev., 2000, 100, 2901–2916 CrossRef CAS;
(b) J. Montgomery, Nickel-catalyzed cyclizations, couplings, and cycloadditions involving three reactive components, Acc. Chem. Res., 2000, 33, 467–473 CrossRef CAS;
(c) J. Montgomery, Nickel-catalyzed reductive cyclizations and couplings, Angew. Chem., Int. Ed., 2004, 43, 3890–3908 CrossRef CAS;
(d) M. Jeganmohan and C.-H. Cheng, Cobalt- and nickel-Catalyzed Regio- and stereoselective reductive coupling of alkynes, allenes, and alkenes with alkenes, Chem. – Eur. J., 2008, 14, 10876–10886 CrossRef CAS;
(e)
H. A. Malik, R. D. Baxter and J. Montgomery, Nickel-Catalyzed Reductive Couplings and Cyclizations, in Catalysis without Precious Metals, ed. R. M. Bullock, Wiley-VCH, Weinheim, 1st edn, 2010, pp. 181–210 Search PubMed;
(f) E. P. Jackson, H. A. Malik, G. J. Sormunen, R. D. Baxter, P. Liu, H. Wang, A.-R. Shareef and J. Montgomery, Mechanistic basis for regioselection and regiodivergence in Nickel-catalyzed reductive couplings, Acc. Chem. Res., 2015, 48, 1736–1745 CrossRef CAS;
(g) E. A. Standley, S. Z. Tasker, K. L. Jensen and T. F. Jamison, Nickel catalysis: synergy between method development and total synthesis, Acc. Chem. Res., 2015, 48, 1503–1514 CrossRef CAS;
(h) M. Holmes and L. A. Schwartz, Intermolecular metal-catalyzed reductive coupling of dienes, allenes, and enynes with carbonyl compounds and imines, Chem. Rev., 2018, 118, 6026–6052 CrossRef CAS.
- E. Oblinger and J. Montgomery, A New Stereoselective method for the preparation of allylic alcohols, J. Am. Chem. Soc., 1997, 119, 9065–9066 CrossRef CAS.
-
(a) S.-i. Ikeda and Y. Sato, Synthesis of stereodefined enynes by the nickel-catalyzed coupling reaction of alkynyltins, alkynes, and enones, J. Am. Chem. Soc., 1994, 116, 5975–5976 CrossRef CAS;
(b) S.-i. Ikeda, K. Kondo and Y. Sato, Nickel-catalyzed tandem coupling of α,β-enones, alkynes, and alkynyltins for the eegio- and stereoselective synthesis of conjugated enynes, J. Org. Chem., 1996, 61, 8248–8255 CrossRef CAS;
(c) J. Montgomery, M. V. Chevliakov and H. L. Brielmann, Nickel-catalyzed heterocycle construction with stereoselective exocyclic alkene introduction, Tetrahedron Lett., 1997, 53, 16449–16462 CrossRef CAS;
(d) S.-i. Ikeda, K. Kondo and Y. Sato, Nickel-catalyzed tandem coupling of enones, alkynes, alkynylzincs, and chlorotrimethylsilane, Chem. Lett., 1999, 28, 1227–1228 CrossRef;
(e) S. Mannathan, M. Jeganmohan and C. H. Cheng, Nickel-catalyzed borylative coupling of alkynes, enones, and bis(pinacolato)diboron as a route to substituted alkenyl boronates, Angew. Chem., Int. Ed., 2009, 48, 2192–2195 CrossRef CAS;
(f) C. M. Yang, M. Jeganmohan, K. Parthasarathy and C. H. Cheng, Highly selective nickel-catalyzed three-component coupling of alkynes with enones and alkenyl boronic acids: a novel route to substituted 1,3-dienes, Org. Lett., 2010, 12, 3610–3613 CrossRef CAS.
- M. Takimoto, K. Shimizu and M. Mori, Nickel-promoted alkylative or arylative carboxylation of alkynes, Org. Lett., 2001, 3, 3345–3347 CrossRef CAS.
- K. M. Miller, C. Molinaro and T. F. Jamison, Catalytic reductive carbon-carbon bond-forming reactions of alkynes, Tetrahedron: Asymmetry, 2003, 14, 3619–3625 CrossRef CAS.
- Y. Ni, K. K. D. Amarasinghe, B. Ksebati and J. Montgomery, First total synthesis and stereochemical definition of isodomoic acid G, Org. Lett., 2003, 5, 3771–3773 CrossRef CAS.
- R. S. Fornicola, K. Subburaj and J. Montgomery, A new entry to the isogeissoschizoid skeleton, Org. Lett., 2002, 4, 615–617 CrossRef CAS.
- K. Shimizu, M. Takimoto and M. Mori, Novel synthesis of heterocycles having a functionalized carbon center via nickel-mediated carboxylation: total synthesis of erythrocarine, Org. Lett., 2003, 5, 2323–2325 CrossRef CAS.
- S. J. Patel and T. F. Jamison, Asymmetric catalytic coupling of organoboranes, alkynes, and imines with a removable (trialkylsilyloxy)ethyl group-girect access to enantiomerically pure primary allylic amines, Angew. Chem., Int. Ed., 2004, 43, 3941–3944 CrossRef CAS.
- Y. Yang, S. F. Zhu, C. Y. Zhou and Q. L. Zhou, Nickel-catalyzed enantioselective alkylative coupling of alkynes and aldehydes: synthesis of chiral allylic alcohols with tetrasubstituted olefins, J. Am. Chem. Soc., 2008, 130, 14052–14053 CrossRef CAS.
- M. Nie, W. Fu, Z. Cao and W. Tang, Enantioselective nickel-catalyzed alkylative alkyne–aldehyde cross-couplings, Org. Chem. Front., 2015, 2, 1322–1325 RSC.
- K. W. Shimkin and J. Montgomery, Synthesis of tetrasubstituted alkenes by tandem metallacycle formation/cross-electrophile coupling, J. Am. Chem. Soc., 2018, 140, 7074–7078 CrossRef CAS.
- A. Herath and J. Montgomery, Catalytic intermolecular enal–alkyne [3+2] reductive cycloadditions, J. Am. Chem. Soc., 2006, 128, 14030–14031 CrossRef CAS.
- M. Ohashi, T. Taniguchi and S. Ogoshi, Nickel-catalyzed formation of cyclopentenone derivatives via the unique cycloaddition of α,β-unsaturated phenyl esters with alkynes, J. Am. Chem. Soc., 2011, 133, 14900–14903 CrossRef CAS.
- L. Guo, F. Song, S. Zhu, H. Li and L. Chu,
syn-Selective alkylarylation of terminal alkynes via the combination of photoredox and nickel catalysis, Nat. Commun., 2018, 9, 4543 CrossRef.
- C. Zhu, H. Yue, B. Maity, I. Atodiresei, L. Cavallo and M. Rueping, A multicomponent synthesis of stereodefined olefins via nickel catalysis and single electron/triplet energy transfer, Nat. Catal., 2019, 2, 678–687 CrossRef CAS.
- H. Yue, C. Zhu, R. Kancherla, F. Liu and M. Rueping, Regioselective hydroalkylation and arylalkylation of alkynes by photoredox/nickel dual catalysis: application and mechanism, Angew. Chem., Int. Ed., 2020, 59, 5738–5746 CrossRef CAS.
- For selected reviews, see:
(a) G. Zeni and R. C. Larock, Synthesis of heterocycles via palladium-catalyzed oxidative addition, Chem. Rev., 2006, 106, 4644–4680 CrossRef CAS;
(b) K. Krüger, A. Tillack and M. Beller, Catalytic synthesis of indoles from alkynes, Adv. Synth. Catal., 2008, 350, 2153–2167 CrossRef;
(c) L. N. Guo, X. H. Duan and Y. M. Liang, Palladium-catalyzed cyclization of propargylic compounds, Acc. Chem. Res., 2011, 44, 111–122 CrossRef CAS;
(d) K. Majumdar, S. Samanta and B. Sinha, Recent developments in palladium-catalyzed formation of five- and six-membered fused heterocycles, Synthesis, 2012, 44, 817–847 CrossRef CAS;
(e) M. Platon, R. Amardeil, L. Djakovitch and J. C. Hierso, Progress in palladium-based catalytic systems for the sustainable synthesis of annulated heterocycles: a focus on indole backbones, Chem. Soc. Rev., 2012, 41, 3929–3968 RSC;
(f) R. Vicente, Recent advances in indole syntheses: New routes for a classic target, Org. Biomol. Chem., 2011, 9, 6469–6480 RSC.
- C. N. Voth and G. R. Dake, Nickel-catalyzed arylative additions on 2-alkynyl-N-arylsulfonylanilides to construct functionalized indoles, Eur. J. Org. Chem., 2020, 2020, 744–748 CrossRef CAS.
- Z. Li, A. Garcia-Dominguez and C. Nevado, Nickel-catalyzed stereoselective dicarbofunctionalization of alkynes, Angew. Chem., Int. Ed., 2016, 55, 6938–6941 CAS.
- A. Garcia-Dominguez, S. Muller and C. Nevado, Nickel-catalyzed intermolecular carbosulfonylation of alkynes via sulfonyl radicals, Angew. Chem., Int. Ed., 2017, 56, 9949–9952 CrossRef CAS.
- A. Yamamoto and M. Suginome, Nickel-catalyzed trans-alkynylboration of alkynes via activation of a boron–chlorine bond, J. Am. Chem. Soc., 2005, 127, 15706–15707 CrossRef CAS.
- T. Igarashi, S. Arai and A. Nishida,
Anti carbocyanative cyclization of enynes under nickel catalysis, J. Org. Chem., 2013, 78, 4366–4372 CrossRef CAS.
- X. Wang, Y. Liu and R. Martin, Ni-catalyzed divergent cyclization/carboxylation of unactivated primary and secondary alkyl halides with CO2, J. Am. Chem. Soc., 2015, 137, 6476–6479 CrossRef CAS.
- X. Zhang, X. Xie and Y. Liu, Nickel-catalyzed cyclization of alkyne-nitriles with organoboronic acids involving anti-carbometalation of alkynes, Chem. Sci., 2016, 7, 5815–5820 RSC.
- Z. Zhou, W. Liu and W. Kong, Ni-catalyzed reductive antiarylative cyclization of alkynones, Org. Lett., 2020, 22, 6982–6987 CrossRef CAS.
- Z. Zhou, J. Chen, H. Chen and W. Kong, Stereoselective synthesis of pentasubstituted 1,3-dienes via Ni-catalyzed reductive coupling of unsymmetrical internal alkynes, Chem. Sci., 2020, 11, 10204–10211 RSC.
- C. Yap, G. M. J. Lenagh-Snow, S. N. Karad, W. Lewis, L. J. Diorazio and H. W. Lam, Enantioselective Nickel-catalyzed intramolecular allylic alkenylations enabled by reversible alkenylnickel E/Z isomerization, Angew. Chem., Int. Ed., 2017, 56, 8216–8220 CrossRef CAS.
- S. N. Karad, H. Panchal, C. Clarke, W. Lewis and H. W. Lam, Enantioselective synthesis of chiral cyclopent-2-enones by nickel-catalyzed desymmetrization of malonate esters, Angew. Chem., Int. Ed., 2018, 57, 9122–9125 CrossRef CAS.
- S. M. Gillbard, C. H. Chung, S. N. Karad, H. Panchal, W. Lewis and H. W. Lam, Synthesis of multisubstituted pyrroles by nickel-catalyzed arylative cyclizations of N-tosyl alkynamides, Chem. Commun., 2018, 54, 11769–11772 RSC.
- G. R. Kumar, R. Kumar, M. Rajesh and M. S. Reddy, A nickel-catalyzed anti-carbometallative cyclization of alkyne-azides with organoboronic acids: synthesis of 2,3-diarylquinolines, Chem. Commun., 2018, 54, 759–762 RSC.
- Z. Lu, X. D. Hu, H. Zhang, X. W. Zhang, J. Cai, M. Usman, H. Cong and W. B. Liu, Enantioselective assembly of cycloenones with a nitrile-containing all-carbon quaternary center from malononitriles enabled by Ni catalysis, J. Am. Chem. Soc., 2020, 142, 7328–7333 CrossRef CAS.
|
This journal is © the Partner Organisations 2020 |