DOI:
10.1039/C7TX00260B
(Paper)
Toxicol. Res., 2018,
7, 396-407
The systemic toxicity of heavy metal mixtures in rats
Received
29th September 2017
, Accepted 14th December 2017
First published on 29th January 2018
Abstract
To explore the health effects of multi-heavy metal exposure, Sprague Dawley (SD) rats were orally given one dose of heavy metal mixtures (HMMs). The eight most common detectable heavy metals in Ningbo area are zinc (Zn), copper (Cu), manganese (Mn), chromium (Cr), nickel (Ni), cadmium (Cd), lead (Pb) and mercury (Hg). In this study, mixtures of these eight heavy metals were prepared using the compounds zinc sulfate heptahydrate, cupric sulfate, manganese dichloride, potassium dichromate, nickel dichloride, cadmium dichloride, lead acetate, and methyl mercury chloride with ion mass proportions of 1070.0, 312.6, 173.1, 82.6, 30.0, 13.3, 6.6, and 1.0, respectively. The rats were randomly divided into four groups. Beside the control group, each rat received a corresponding dose of HMMs 215, 464 or 1000 mg per kg body weight (bwt), respectively. The rats were observed for 4 weeks. During the last week of observation, the Morris water maze test was used to investigate spatial learning and memory in the treated rats. The rats were exsanguinated under complete chloral hydrate anesthesia and organ coefficients were measured. Biochemical tests of blood and serum samples were carried out. The results showed abnormalities in the hematological system, decreased renal function, hepatic injury and disturbances in the electrolyte balance of the rats treated with a high dose of HMMs. Death of some rats was also observed. This paper analyzed how a one-time high dose oral administration of HMMs induced systemic toxicity.
1. Introduction
Heavy metals are high density elements occurring naturally in the environment in a minute quantity.1 With the rise of industrialization and anthropogenic activities heavy metal pollution occurred especially in China.2 Mining and manufacturing cause these toxic metals to be released into water or the atmosphere. Some of these metals are also used in fuels and coal, and therefore are released during combustion, ore smelting or through waste disposal machineries in the form of metal fumes of suspended particulates. Some trace amounts of heavy metals such as zinc and copper are useful for the body, but other toxic metals can accumulate in large quantities and cause health problems. Multiple heavy metals may simultaneously enter the human body through air, water or food. Once inside the body, heavy metals are not easily metabolized or excreted, they build up quickly and bioaccumulate leading to an increase in their concentration.3 As a major port city in eastern China, Ningbo is known for the variety of sea food it offers. Fish, crab, shell fish and shrimp are among the most consumed sea food here. Recent studies using fish as bioindicators of trace element pollution revealed high levels of heavy metals in migratory fish.4 Similar research on sea weed produced similar results.1,5 According to the priority chemical list, lead, mercury, organic mercury compounds, cadmium, hexavalent chromium and nickel are all metals with great public health concern.6 Due to their toxicity and high frequency of occurrence, cadmium and its compounds, hexavalent chromium compounds and nickel compounds are classified as human carcinogens level 1, and inorganic lead compounds are classified as level 2A by the International Agency for Research on Cancer (IARC). Lead causes damage to the central nervous system and kidneys and affects heme synthesis.7 Cadmium can induce disturbances in calcium metabolism, renal tubular dysfunction, osteoporosis, and even lung cancer.8 Methyl mercury is mainly absorbed through the digestive tract. The absorption of inorganic mercury is below 15% while that of alkyl mercury is above 90%. Mercury has toxic effects on the digestive and immune systems. It also affects the central and peripheral nervous systems.9 Chromium(VI) compounds or hexavalent chromium is the most poisonous among the chromium compounds. It has high genotoxicity effects such as chromosomal aberrations, sister chromatid exchanges, and respiratory carcinogenicity. It can combine with nucleic acids and nucleoproteins and affect the genetic code of the body leading to the induction of cancer.10 Nickel compound exposure can lead to nephrotoxicity, skin irritation and hypersensitivity or lung and nasal cancers. Inhalation is the major route of exposure for nickel toxicity but it may also be absorbed through food. Nickel ions have been shown to contain a high concentration of potent carcinogens. Exposure to these compounds causes a high incidence of nasal and pharyngeal cancers. Once inside the cells, nickel ions accumulate and bind to various peptides and amino acids lowering the oxidation potential and generating oxygen radicals that cause damage to cells.11 Copper, zinc, and manganese are common nutritional metallic elements beneficial to the body at moderate levels but may induce toxicity at high levels. Copper is a key constituent of the respiratory enzyme complex cytochrome C oxidase (COX) and is important in facilitating iron uptake. Excess copper exposure can lead to gastrointestinal symptoms and hepatocellular toxicity. Zinc functions as the structural ion for many specific enzymes. It is involved in a wide range of biological processes, such as signal transduction, gene expression and apoptosis. Excess zinc exposure can lead to ataxia, lethargy, and the inhibition of copper and iron absorption. Manganese(II) ions are the cofactors of many enzymes, particularly those involved in the detoxification of superoxide free radicals. Manganese poisoning can lead to serious neurological symptoms, and psychiatric and motor disturbances.12 Many heavy metals are naturally occurring elements in the environment and affect nearly all biological systems. Epidemiological and experimental evidence showed that their mixture may have combined effects that are different from their individual effects.13–16 There are countless theories about the toxicity of these metals but few studies on mimicking multi-heavy metal exposure simultaneously in the real world. Whether individually or jointly with other metals, the main general route of exposure is through air, soil, water and food. Exposure through food causes metal accumulation at different proportions and site-specific toxicity. In order to mimic multiple heavy metal exposure simultaneously, the eight most common detectable heavy metals predominantly found in fish eaten in the Ningbo area were used to prepare heavy metal mixtures (HMMs). After one dose of HMM oral administration, rats were observed for 4 weeks to study the effect on them.
2. Materials and methods
2.1 Materials
Lead acetate ((CH3COO)2Pb·3H2O, AR ≥ 99.5), cadmium dichloride (CdCl2·2.5H2O, AR ≥ 99.0), nickel dichloride (NiCl2·6H2O, AR ≥ 98.0), manganese dichloride (MnCl2·4H2O, AR ≥ 99.0), zinc sulfate heptahydrate (ZnSO4·7H2O, AR ≥ 99.5), cupric sulfate (CuSO4·5H2O, AR ≥ 99.0), and potassium dichromate (K2Cr2O7, AR ≥ 99.8), were all purchased from Sinopharm Chemical Reagent Co., Ltd (Shanghai, People's Republic of China). Other reagents used include methyl mercury chloride which was purchased from Dr Ehrenstorfer GmbH (Germany). Biochemical analysis was performed using a Hitachi 7600-110 auto analyzer (Hitachi Ltd, Tokyo, Japan) and hematological analysis using a Sysmex blood analyser (Sysmex XT-1800i; Sysmex Co., Kobe, Japan). Sprague Dawley (SD) rats were obtained from the Zhejiang Provincial Laboratory Animal Science Center (Hangzhou, Zhejiang, People's Republic of China). Water maze testing was performed using a Morris water maze video analysis system (RD1101-MWM-G, Shanghai Mobiledatum Information Technology Co., Ltd, People's Republic of China).
2.2 Methods
2.2.1 Proportion estimation of each heavy metal in the mixtures.
In this study, the proportion of each heavy metal in the HMMs is based on the estimation of the eight most common detectable heavy metals in aquatic products including fish, shrimp, shellfish and crabs consumed in the Ningbo area; fish was used to represent all these aquatic products in this paper.17,18 The mass percentages of zinc (Zn), copper (Cu), manganese (Mn), chromium (Cr), nickel (Ni), cadmium (Cd), lead (Pb) and mercury (Hg) in this HMM were 64.68%, 19.08%, 10.6%, 2.53%, 1.84%, 0.82%, 0.4% and 0.06%, respectively. The HMMs were composed of the compounds zinc sulfate heptahydrate, cupric sulfate, manganese dichloride, potassium dichromate, nickel dichloride, cadmium dichloride, lead acetate, and methyl mercury chloride with ion mass proportions of 1070.0, 312.6, 173.1, 82.6, 30.0, 13.3, 6.6, and 1.0, respectively. Besides the control group, three different dose groups of HMMs were prepared, and the total compound doses were 215, 464, and 1000 mg per kg body weight (bwt), respectively (Table 1).
Table 1 Experiment groups and concentration of each heavy metal in heavy metal mixtures (HMMs)
Compound used to prepare HMMs |
Experiment groups (mg per kg body weight) |
Control |
215 |
464 |
1000 |
Total compound dose of HMMs in each group was the sum of the eight heavy metals. |
(CH3COO)2Pb·3H2O |
— |
0.376 |
0.811 |
1.747 |
CdCl2·2.5H2O |
— |
0.832 |
1.796 |
3.872 |
CH3HgCl |
— |
0.039 |
0.083 |
0.180 |
CuSO4·5H2O |
— |
37.974 |
81.952 |
176.622 |
ZnSO4·7H2O |
— |
145.512 |
314.036 |
676.802 |
MnCl2·4H2O |
— |
19.282 |
41.613 |
89.684 |
K2Cr2O7 |
— |
7.227 |
15.597 |
33.613 |
NiCl2·6H2O |
— |
3.758 |
8.111 |
17.480 |
2.2.2 Heavy metal mixture preparation and animal care.
The heavy metal compounds were individually prepared at certain high concentrations by dissolving and further diluting in de-ionized water according to the working dosage. Due to the precipitation reactions of some of the heavy metals, Pb, Cd, Hg, and Ni solutions were mixed and named mixture A while Cr, Cu, Zn and Mn solutions were mixed and named mixture B. All SD rats were kept under stress-free, clean, and animal-friendly conditions and standard environmental conditions (relative humidity: 60% ± 10%, room temperature: 20 °C ± 2 °C, and 12-hour light/dark cycle). The rats had access to food and water ad libitum. The experimental design, transportation and care of the animals were approved and performed in compliance with the Ningbo University Institutional Animal Care and Use Committee (AEWC-2014-102).
2.2.3 Experimental design.
Twenty female and twenty male healthy adult SD rats 7 week old were randomly and averagely divided into control and three different HMM groups. Before gavage, the feeding trough was removed and all the rats were required to undergo overnight fasting. Every rat in the three treatment groups received oral administration first of mixture A followed by mixture B after 1 hour. The rats were observed for 4 weeks.
2.2.4 Morris water maze test.
The Morris water maze (MWM) is a round pool (180 cm diameter, 60 cm depth) with black tank walls, filled with water mixed with black ink and maintained at a temperature of 22–24 °C. Visual cues were arrayed around four directions of the pool. The water maze test was performed during the last week of observation. During the first three days, a hidden platform test was carried out. A 12 cm diameter plastic platform was placed in the same quadrant at 1 cm below the surface of the water. There were three trials on the first two days and two trials on the third day. Each rat was released from one of the four entry points and allowed 70 seconds to find the platform. At the end of each trial, if the rat failed to find the platform, it would be permitted to remain in the water for an extra 30 seconds. The day after the hidden platform test, the probe test was performed without the platform. This was done only once. Each rat was released from the opposite side quadrant of the original platform, and had 70 seconds to search for the platform. The swim time, distance traveled and swim path around the pool were recorded during all the trials.
2.2.5 Hematological and biochemical analysis, and organ coefficients.
After the four week observation period, the body weights of all the rats were recorded after which they were exsanguinated under complete chloral hydrate anesthesia. Laboratory tests of blood and serum samples were carried out. Hematological tests to measure the status of red blood cells (rbc), white blood cells (wbc) and their components as well as hemoglobin (HGB) and prothrombin levels were performed. To analyze the hepatic function and renal profile, biochemical and hormonal tests such as alanine aminotransferase (ALT), alkaline phosphatase (ALP), blood urea nitrogen and creatinine, albumin, globulin, total protein and total bile acid levels were measured. The brain, heart, lung, liver, spleen, kidneys, and testes were taken out and weighed immediately. The organ coefficients were calculated as the ratio of tissue wet weight (g) to body weight (100 g).
2.2.6 Statistical analysis.
Statistical analysis was performed using SPSS 16.0 statistical software (SPSS Inc., USA). One-way ANOVA was used for organ coefficients’ comparison, hematological, biochemical, and hormonal analyses and probe test. Repeated measures of ANOVA were used to analyze the change of body weight and the MWM learning data. The LSD-t or Dunnett-t post hoc tests were followed when appropriate. Post hoc tests were not performed on those groups with fewer than two cases. The Student's t-test was used to compare the gender differences. The results are presented as means ± standard error (SEM). All data generated or analyzed during this study are included in this published article.
3. Results
3.1 The survival of rats after the exposure
After oral administration of heavy metal mixtures, the time of death and the survival time of the rats were recorded. One male rat in the 1000 mg per kg bwt group and two female rats in the 464 mg per kg bwt group died within 48 hours. In the second week, another male rat in the 1000 mg per kg bwt group and one female rat in the 464 mg per kg bwt group died. One other male rat in the 464 mg per kg bwt group died in the fourth week.
3.2 The body weight changes
The ANOVA analysis results showed that on the day before treatment (0d), no difference in body weight was found among the four groups of animals including both females and males. The repeated measures ANOVA analysis and multiple comparisons showed that the body weights of the 464 mg per kg bwt female group were significantly lower than that of the control group in the second week after the treatment. The increase in body weight among the 464 mg per kg bwt female and male groups was slower than that of the other groups (Fig. 1).
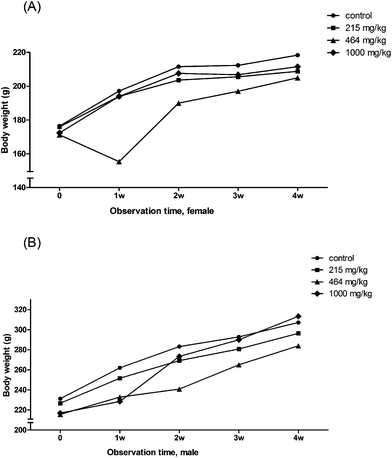 |
| Fig. 1 The change in body weight during the 4 week observation. (A) The mean body weight changes in female rats. (B) The mean body weight changes in male rats. | |
3.3 Organ coefficients
No significant differences were found in the organ coefficients of the brain, heart, lungs, liver or testes in male or female rats among the HMM groups. However, there were differences in the kidney coefficients among the four female groups (F(3,13) = 2.849, P = 0.078). The coefficient of kidneys in the 1000 mg per kg bwt female group (0.7283 ± 0.04040 g per 100 g) significantly increased compared with the control group (0.6658 ± 0.03709 g per 100 g) or 215 mg per kg bwt (0.6848 ± 0.03282 g per 100 g) group. The change of the spleen coefficient in males was different from that of females. The coefficient of spleen in the 1000 mg per kg bwt male group (0.1571 ± 0.01687 g per 100 g) was significantly lower than that of the 464 mg per kg bwt (0.2024 ± 0.03294) and 215 mg per kg bwt (0.1935 ± 0.01406 g per 100 g) groups as well as the control group (0.1914 ± 0.02645 g per 100 g). However, the coefficient of spleen in the 464 mg per kg bwt female group (0.2560 ± 0.03154 g per 100 g) was significantly higher than that of the 215 mg per kg bwt group (0.1952 ± 0.03626 g per 100 g) or the control group (0.2128 ± 0.01010 g per 100 g).
3.4 Hematological analysis
Some hematological parameters significantly decreased or increased among the different HMM dose groups. In the 464 mg per kg bwt female group, there was a significant decrease in the number of neutrophils but an increase in lymphocytes compared to the control group. However, in the 1000 mg per kg bwt male group, the percentage of neutrophils significantly increased while that of lymphocytes decreased compared to the control group. The basophil count for the high dose group was significantly lower than the low dose groups (Fig. 2). There was a significant decrease in the RBC, HGB, RDW-CV and MPV in the 1000 mg per kg bwt male group compared to the control group. A significant decrease was also found in the PDW-CV and PCT between the control group and the 1000 mg per kg bwt female group. The plasma prothrombin time (PT) in the 1000 mg per kg bwt group was 32.27 ± 2.94 seconds, significantly lower than the control group (35.93 ± 5.32 seconds). The prothrombin time international ratio (PT-INR) in the 1000 mg per kg bwt group was 2.62 ± 0.24, also significantly lower than the control group (2.92 ± 0.43) (Fig. 3).
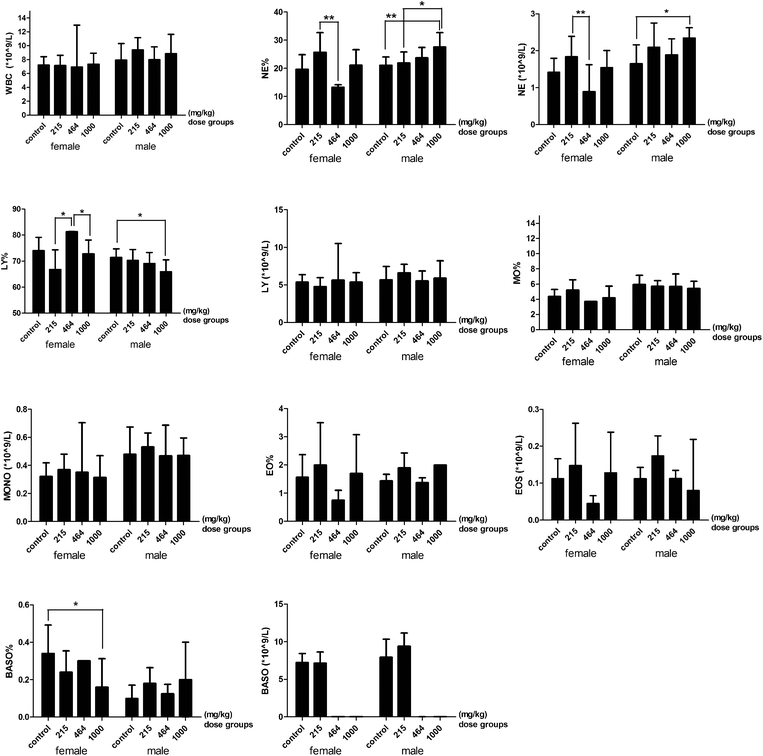 |
| Fig. 2 WBC related parameters of the rats treated with heavy metal mixtures. Note: There is a significant difference between the two groups (*P ≤ 0.1, **P ≤ 0.05). The error bars mean SE. Abbreviations: WBC: white blood cells; NE%: the percent of neutrophils in WBC; NE: neutrophil; LY%, the percent of lymphocytes in WBC; LY: lymphocyte; MO%, the percent of monocytes in WBC; MONO, monocyte; EO%: the percent of eosinophils in WBC; EOS: eosinophil; BASO%: the percent of basophils in WBC; BASO, basophil. | |
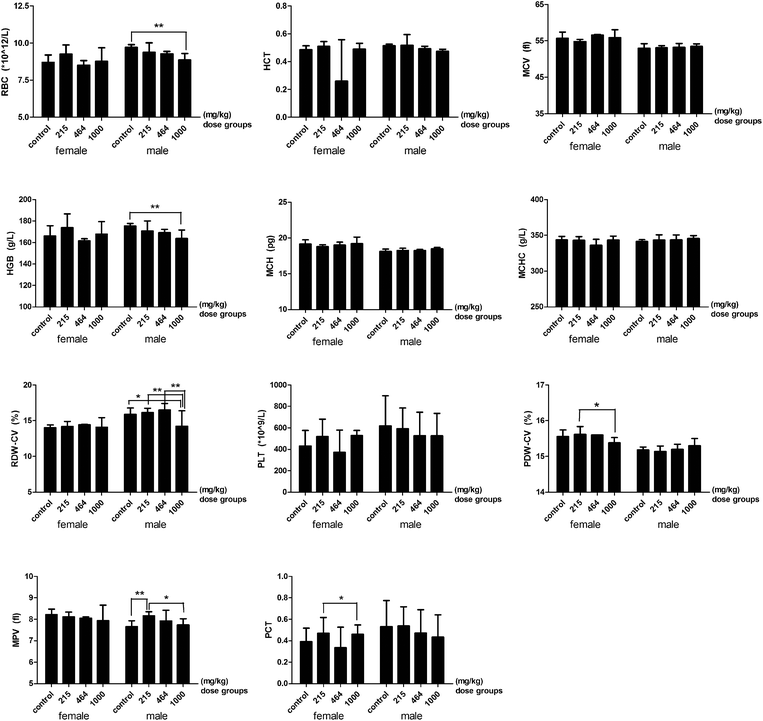 |
| Fig. 3 RBC, HGB and PLT related parameters of rats treated with heavy metal mixtures. Note: There is a significant difference between the two groups (*P ≤ 0.1, **P ≤ 0.05). Abbreviations: RBC, red blood cells; HCT, hematocrit; MCV, mean corpuscular volume; HGB, hemoglobin; MCH, mean corpuscular hemoglobin; MCHC, mean corpuscular hemoglobin concentration; RDW-CV, red blood cell distribution width; PLT, platelet; PDW-CV, platelet distribution width; MPV, mean platelet volume; PCT, platelet crit. | |
3.5 Biochemical and hormonal analysis
Biochemical tests to analyze hepatic function, renal function, serum electrolytes and lipids were carried out. The serum alanine aminotransferase levels (ALT) in the 464 mg per kg bwt female group were about 10 times higher than the other groups. The serum levels of AST/ALT in the 464 mg per kg bwt male group significantly increased compared to the control group. The high dose (1000 mg per kg bwt) of the HMMs induced a significant increase in serum indirect bilirubin levels in females. The serum levels of total protein, albumin, and globulin and the ratio of albumin and globulin significantly decreased in the 464 mg per kg bwt and the 1000 mg per kg bwt groups compared to the control group. The serum levels of alkaline phosphatase (ALP), gamma-glutamyl transpeptidase (GGT), total bile acid (TBA) and glycylproline dipeptidyl aminopeptidase (GPDA) significantly increased in the 464 mg per kg bwt or the 1000 mg per kg bwt group compared to the control group, except that the ALP in the 1000 mg per kg bwt male group significantly decreased (Fig. 4).
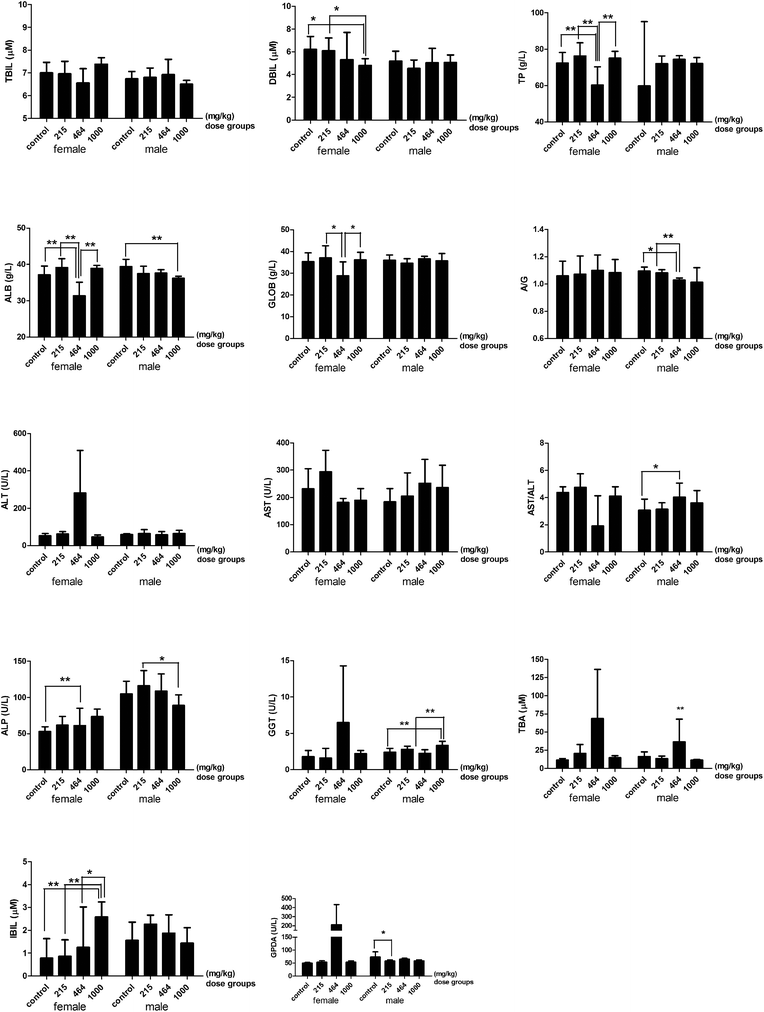 |
| Fig. 4 The hepatic function index of rats treated with heavy metal mixtures. Note: There is a significant difference between the two groups (*P ≤ 0.1, **P ≤ 0.05). Abbreviations: TBIL, total bilirubin; DBIL, direct bilirubin; IBIL, indirect bilirubin; TP, total protein; ALB, albumin; GLOB, globulin; A/G, albumin/globulin; ALT, alanine aminotransferase; AST, aspartate aminotransferase; ALP, alkaline phosphatase; GGT, gamma-glutamyl transpeptidase; TBA, total bile acid; GPDA, glycylproline dipeptidyl aminopeptidase. | |
The renal profile parameters, urea nitrogen and the ratio of urea nitrogen and creatinine significantly decreased in the 1000 mg per kg bwt group compared to the lower dose groups (Fig. 5). No significant difference in the serum levels of creatinine and uric acid was detected between any dose groups. The levels of serum electrolytes changed according to the dose of heavy metal mixtures given (Fig. 6). The serum levels of sodium (Na), chlorine (Cl), calcium (Ca), magnesium (Mg), and iron (Fe) were significantly lower in the 464 mg per kg bwt or the 1000 mg per kg bwt group compared to the control group. The phosphorus level in the 464 mg per kg bwt group was significantly higher than the control group. In the lipid profile, there was a significant decrease in the levels of total cholesterol (TG), high-density lipoprotein (HDLC), low density lipoprotein (LDLC) and glucose (GLU) in the higher dose (464 mg per kg bwt or 1000 mg per kg bwt) HMM groups. However, the serum triglyceride level was much higher in the 464 mg per kg bwt group compared to the control group. The urinary biochemistry and electrolyte analysis revealed that urinary ALP in the 464 mg per kg bwt group was 107.2 ± 52.39 U L−1, higher than other groups. The concentration of urinary calcium and glucose significantly decreased in the 215 mg per kg bwt, 464 mg per kg bwt, and 1000 mg per kg bwt groups, compared to the control group.
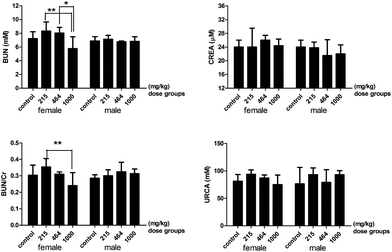 |
| Fig. 5 The renal profile parameters of rats treated with heavy metal mixtures. Note: There is a significant difference between the two groups (*P ≤ 0.1, **P ≤ 0.05). Abbreviations: BUN, blood urea nitrogen; CREA, creatinine; BUN/Cr, blood urea nitrogen/creatinine; URCA, uric acid. | |
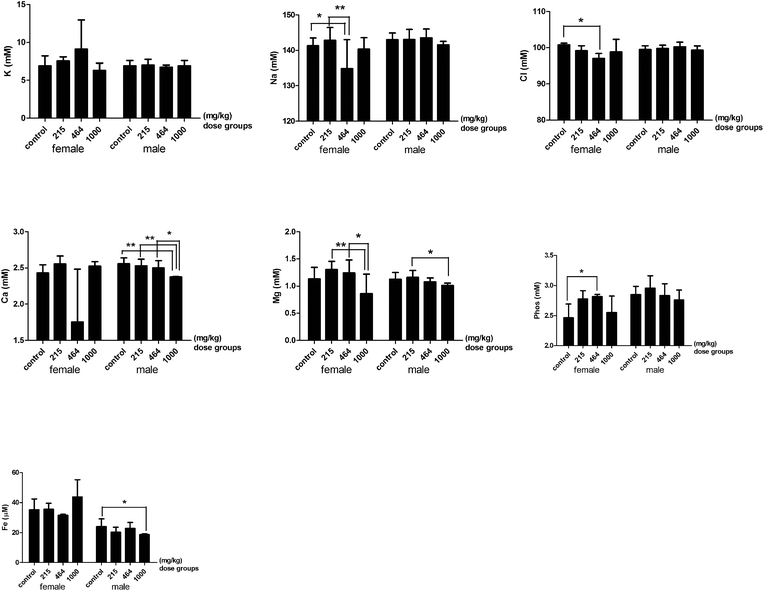 |
| Fig. 6 Serum electrolytes of rats treated with heavy metal mixtures. Note: There is a significant difference between the two groups (*P ≤ 0.1, **P ≤ 0.05). Abbreviations: PHOS, phosphorus. | |
The heavy metal mixture exposure also induced some changes in serum hormones in rats (Table 2). The serum thyroxin (T4) significantly increased in the 1000 mg per kg bwt male group compared to the control group, but there was a dose dependent decrease in female groups. The serum prolactin in the 464 mg per kg bwt female group and the serum triiodothyronine in the 464 mg per kg bwt male group increased, but not significantly. There was a dose dependent decrease in the concentration of total testosterone in male groups, but the differences were not very significant (P > 0.1).
Table 2 The levels of hormones in rats after treatment with different doses of heavy metal mixtures (HMMs) and 4 week observation
Female/male |
Control |
215 mg per kg bwt |
464 mg per kg bwt |
1000 mg per kg bwt |
There is a significant difference compared with other groups (*P ≤ 0.1, **P ≤ 0.05). Abbreviations: PRL, prolactin; Prog, progesterone; E2, estradiol; TT, total testosterone; T3, triiodothyronine; T4, thyroxine. |
Female
|
PRL (μg L−1) |
0.053 ± 0.033 |
0.083 ± 0.040 |
0.280 ± 0.283 |
0.017 ± 0.006 |
Prog (μg L−1) |
20.990 ± 3.926 |
18.853 ± 5.447 |
31.035 ± 12.678 |
21.243 ± 7.214 |
E2 (ng L−1) |
51.667 ± 11.150 |
35.333 ± 37.820 |
50.500 ± 21.920 |
34.667 ± 23.029 |
TT (μg L−1) |
0.893 ± 0.065 |
0.820 ± 0.192 |
0.800 ± 0.071 |
0.810 ± 0.131 |
T4 (μg L−1) |
64.748 ± 12.832 |
63.360 ± 6.426 |
54.165 ± 4.052 |
53.210 ± 9.212 |
Male
|
PRL (μg L−1) |
0.023 ± 0.023 |
0.008 ± 0.010 |
0.013 ± 0.006 |
0.005 ± 0.007 |
Prog (μg L−1) |
9.323 ± 0.813* |
4.245 ± 2.608* |
8.303 ± 4.604* |
9.080 ± 2.220* |
E2 (ng L−1) |
7.667 ± 4.509 |
8.250 ± 2.630 |
15.667 ± 12.662 |
8.000 ± 1.414 |
TT (μg L−1) |
8.227 ± 7.306 |
3.335 ± 2.432 |
2.963 ± 1.176 |
2.380 ± 2.107 |
T3 (μg L−1) |
0.460 ± 0.040 |
0.508 ± 0.086 |
0.557 ± 0.067 |
0.545 ± 0.064 |
T4 (μg L−1) |
50.447 ± 6.316** |
53.783 ± 7.428** |
58.457 ± 3.541 |
66.065 ± 2.029** |
3.6 The results of the Morris water maze test
There was a sharp decrease in latency and distance traveled over the trial days in all groups (ANOVA for repeated measures: days effect: latency: F(2,118) = 4.833, P = 0.01; traveled distance: F(2,96) = 9.892, P < 0.001). The last trials of the hidden platform tests on days 1, 2 and 3 were the 3rd, 6th and 8th trials respectively (Fig. 7). In trial 6, the total distance traveled by the control group, the 215 mg per kg bwt, and 1000 mg per kg bwt groups significantly decreased compared to the 464 mg per kg bwt group. In the 8th trial, a shorter time was taken in finding the platform by the control group (29.62 ± 8.69 seconds) and the total distance traveled was 763.61 ± 188.75 cm, much lower than other groups. The search strategy analysis revealed that on test day 3, more spatial search was performed by the control group compared to the 464 mg per kg bwt group. The probe trial showed significant differences in the times across the platform among the female groups (F(3,13) = 2.685, P < 0.1), with the swimming time in the correct quadrant (F(3,13) = 4.081, P < 0.05), and the percent of distance traveled (F(3,13) = 4.081, P < 0.05). Further multiple comparisons revealed that in the control group female rats travelled across the platform significantly quicker, spent more time swimming and traveled longer distances in the correct quadrant and the platform perimeter zone, compared to that of the other groups (Fig. 8). The 215 mg per kg bwt male rats also traveled significantly longer distances in the platform perimeter zone, compared to the 464 mg per kg bwt or 1000 mg per kg bwt group.
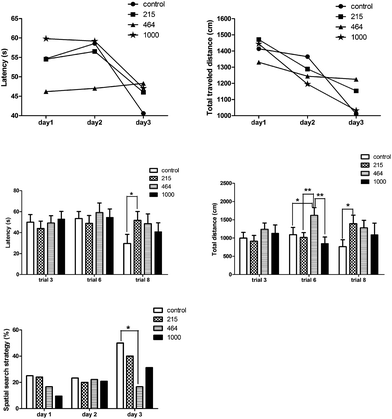 |
| Fig. 7 Effect of heavy metal mixtures on the spatial memory of rats by hidden platform tests. Note: There is a significant difference between the two groups (*P ≤ 0.1, **P ≤ 0.05). | |
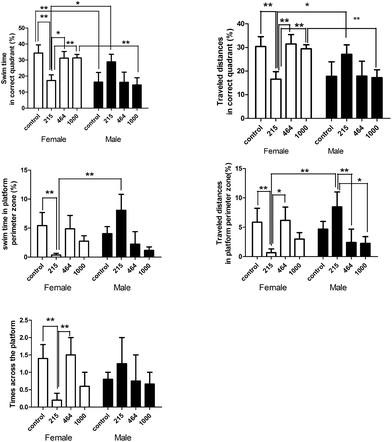 |
| Fig. 8 Effect of heavy metal mixtures on the spatial memory of rats by the probe trial. Note: There is a significant difference between the two groups (*P ≤ 0.1, **P ≤ 0.05). | |
There is, however, a gender difference in the effect of HMM exposure in the rats. In the control group, the female rats spent significantly more time swimming in the correct quadrant than the male rats. In the 215 mg per kg bwt group, the male rats spent a lot of time swimming and also traveled longer distances in the right quadrant and within the perimeter of the platform than the female rats. In the 1000 mg per kg bwt group, the female rats spent much more time swimming and traveled longer distances in the correct quadrant than their male counterparts. The HMM exposure induced more toxic effects on the 215 mg per kg bwt and 464 mg per kg bwt female groups than on the 1000 mg per kg bwt group.
4. Discussion
There is a growing concern about the health hazards posed by multi-heavy metal pollution through sea food consumption. Generally humans are not exposed to a single toxic metal in the environment, but rather are exposed to heterogeneous metal mixtures with multiple health hazards.19 Therefore assessment of the toxic effect posed by combinations of different heavy metals has more practical significance than evaluating the effect of exposure to a single heavy metal. The proportion and concentration of heavy metals vary based on the environmental and geographical location, thus different locations have different amounts of heavy metal exposure. The research by You et al. in 2011 to assess heavy metals in drinking water in parts of China and the study by Sun et al. in 2013 to measure PM2.5 pollution in China all concluded that the essential elements, zinc, copper and manganese, make up the majority in all the pathways.20,21 Based on the data obtained from the estimation of the proportion of HMMs in fish, the three most predominant metals found in fish in Ningbo were zinc, copper and manganese. This is in line with previous studies, however it is not clear what effect high dose exposure to heterogeneous essential heavy metals will cause on the body as well as their combined effect with other non-essential heavy metals. From the results of this study, there was a significant dose-dependent response in the various groups. No death was found in the control and the low dose HMM exposure groups during the 4 week observation period but only a few rats survived in the high dose group. There was no significant weight gain among the rats exposed to high doses of the HMMs rather a weight loss and other abnormalities of the hematological system, injury of hepatic and renal function and the decline of neurobehavioral function. The electrolyte balance, lipid metabolism, and endocrine system were also altered. The fatality rate of HMMs in 4 weeks was 40% in the 464 mg per kg bwt group and 20% in the 1000 mg per kg bwt group. This brought about lots of further questions on the probing and evaluation of the experimental procedure and all the steps. After careful evaluation and ruling out experimental errors, we concluded that the fatality may be due to the precipitation of metals in the high dose group or the protective effect of the greater proportion of copper, zinc and manganese in the 1000 mg per kg bwt group compared to the proportion in the 464 mg per kg bwt group which may have a relatively less protective effect. Further studies and research are needed to confirm the antagonistic, synergistic and addictive effects of heterogeneous heavy metals on the body and at what ratio they provide a protective effect or become toxic.
4.1 Effect of HMMs on the hematological system
A study conducted at The National Toxicology Program (USA) with exposure of 4 mg per kg body weight per day sodium dichromate for five days, equivalent to the total exposure of 14 mg per kg bwt chromium ions, concluded that the male rat mean cell volume and mean cell hemoglobin decreased.22 In the 464 and 1000 mg per kg bwt groups, a significant decrease in RBC count, hemoglobin, MPV, RDW-CV, PDW-CV and PCT was found compared to the control group. These results can lead to anemia, coagulation disorders and other hemorrhagic disturbances. High doses of Cu and Zn have a detrimental effect on the hematopoietic system. This phenomenon was observed when intraperitoneal administration of 8 mg per kg bwt zinc acetate produced toxic effects on the hematopoietic, cytogenetic and endocrine systems.23 In the 464 mg per kg bwt female group, the number of neutrophils decreased but the lymphocytes increased compared to the control group, but complete opposite is seen in the 1000 mg per kg bwt group, where the percentage of neutrophils significantly increased while that of lymphocytes decreased. This needs further investigation on the role of HMMs in cancers, as a high lymphocyte count with a low neutrophil count might be caused by cancers and a low level of lymphocytes is associated with an increased rate of infection.
4.2 Effect of HMMs on the neurological system
Oloveira's study concluded that 1% w/v zinc acetate in drinking water for 60 days caused learning impairment in mice.24 The result of the MWM test confirms the inability of the rats in the 464 and 1000 mg per kg bwt groups to swim in the correct quadrant, this may be due to the decrease in cognitive function and memory loss. Exposure to high Mn levels is known to cause adverse neurological effects in humans, therefore their combination with other heavy metals (including essential and non-essential) has the potential to induce neurological damage. In trial 6, the total distance traveled by the control group significantly decreased compared to the 464 and 1000 mg per kg bwt groups just like the 8th trial where the rats in the control group located the platform quickly while the 464 and 1000 mg per kg bwt groups had difficulty in locating the platform. Female rats in the control group spent significantly more time swimming in the correct quadrant than the male rats but the male rats in the high dose group spent more time swimming than their female counterparts. This could be an indication that HMMs could have different effects on different genders. The overall MWM test result showed that HMMs induced more neurological effects on the 215 and 464 mg per kg bwt groups than the 1000 mg per kg bwt group. Could this be due to the metal–metal reaction or the protective effect of some of the essential metals? More research is required to find out in detail about the metal–metal interaction and the combined effect of HMMs on memory.
4.3 Effect of HMMs on electrolytes, liver and renal function
Exposure to individual as well as combined non-essential heavy metals Pb, Cd and Hg has a negative effect on the concentration and distribution of essential metals Cu and Zn, however it is not completely clear what effect high levels of these essential heavy metals will have on electrolytes in the body. Several research articles confirmed that increased Zn and Cu might be the major toxic factors at high doses of HMMs with regard to the electrolyte levels but may also play certain protective roles at lower doses. In this study, the serum levels of Na, Cl, Ca, Mg, and Fe as well as TG, HDLC, LDLC and GLU were significantly lower in the 464 mg per kg bwt or the 1000 mg per kg bwt group compared to the control group. There was a significant decrease in the levels of total protein, albumin, globulin, the ratio of albumin and globulin, PT and PT-INR in the 464 and the 1000 mg per kg bwt groups compared to the control group. These results demonstrate the effect of HMMs on the kidney and liver and the difficulty in clotting the blood. Excessive exposure of Cr and Mn has an effect on thyroid functions and can cause goiter. From the results, there was a significant increase in the serum T4 levels, affecting the secretion and metabolism of thyroid hormones. The protective effects of zinc against many metals, such as cadmium, chromium and nickel, were widely and deeply studied. The nickel exposure in the 1000 mg per kg bwt group was 4.3 mg per kg bwt which is relatively low. According to a study by Whanger et al., exposure to 100 mg per kg bwt nickel acetate diet which is equivalent to 5 mg kg−1 d−1 nickel had no effect on the rats25 and Afonne et al. demonstrated that zinc prevents chromium-induced testicular injury in mice.26 Other research studies showed that slight renal tubular epithelial damage was observed in male rats with the lowest-observed-adverse-effect level (LOAEL) of 10 mg per kg bwt copper per day for 2 weeks, and higher levels of cupric sulfate led to kidney and liver damage and induced microcytic anemia.27 The reduction in blood urea nitrogen and the ratio of urea nitrogen and creatinine levels in the high dose group is an indication of the HMM effect on the renal function.
4.4 Relevance of the doses to humans
Analyzing the body surface area of the rats and extrapolating the exposure rate of the doses on humans will be equivalent to a ratio of 6.3
:
1 (mg kg−1) respectively.18 Thus the exposure due to fish consumption in Ningbo in relation to the 215, 464, and 1000 mg per kg body weight in rats will be equivalent to a total of 20 day, 6 week and 13 week HMM exposure, respectively. Gad et al. reported that the LD50 values for potassium dichromate varied in female and male rats, and the females were more sensitive.28 The results are consistent with our experiment, the female and male rats responded differently, the female rats in the 464 mg per kg bwt group suffered more deaths than the male rats.
5. Conclusion
Our results demonstrate that high dose exposure to HMMs induced toxic effects on various organs and systems in the form of loss of body weight, disturbance in the hematological system, hepatic injury and renal insufficiency as well as abnormal neurological behaviors and animal death. Destabilization of the electrolyte balance, lipid and endocrine metabolism were also observed. We recommend that beside the quantity, the proportion of different heavy metals consumed in food should be taken into consideration while assessing risks associated with multi-heavy metal exposure. Further studies are necessary to elucidate the combined effects of HMMs after long-term low-level exposure and to figure out the unique roles, characteristics and proportion of each metal in different pathways.
Conflicts of interest
There are no conflicts of interest to declare.
Acknowledgements
The authors would like to thank Ms Linda Bowman for her assistance in the preparation of this article.
This work was partly supported by the National Nature Science Foundation of China (81273111), the Scientific Projects of Zhejiang Province (2015C33148 and 2015C37117), the Foundations of Innovative Research Team of Educational Commission of Zhejiang Province (T200907), the Ningbo Scientific Innovation Team for Environmental Hazardous Factor Control and Prevention (2016C51001), the Zhejiang Key Laboratory of Pathophysiology (201703), and KC Wong Magna Fund in Ningbo University.
References
- J. Lu, A. Li and P. Huang, Distribution, sources and contamination assessment of heavy metals in surface sediments of the South Yellow Sea and northern part of the East China Sea, Mar. Pollut. Bull., 2017, 124(1), 470–479 CrossRef CAS PubMed.
- X. S. Luo, S. Yu, Y. G. Zhu and X. D. Li, Trace metal contamination in urban soils of China, Sci. Total Environ., 2012, 421, 17–30 CrossRef PubMed.
- Z. Cooper, R. Bringolf, R. Cooper, K. Loftis and A. L. Bryan,
et al., Heavy metal bioaccumulation in two passerines with differing migration strategies, Sci. Total Environ., 2017, 592, 25–32 CrossRef CAS PubMed.
- C. Plessl, E. O. Otachi, W. Korner, A. Avenant-Oldewage and F. Jirsa, Fish as bioindicators for trace element pollution from two contrasting lakes in the Eastern Rift Valley, Kenya: spatial and temporal aspects, Environ. Sci. Pollut. Res. Int., 2017, 421, 17–30 Search PubMed.
- C. Rubio, G. Napoleone, G. Luis-Gonzalez, A. J. Gutierrez and D. Gonzalez-Weller,
et al., Metals in edible seaweed, Chemosphere, 2017, 173, 572–579 CrossRef CAS PubMed.
-
ATSDR, The Priority List of Hazardous Substances That Will Be the Subject of Toxicological Profiles, Agency for Toxic Substances and Disease Registry, GA, 2013 Search PubMed.
-
H. Abadin, A. Ashizawa, Y.-W. Stevens, F. Llados and G. Diamond, et al., Toxicological profile for lead, US Department of Health and Human Services, Public Health Service Agency for Toxic Substances and Disease Registry, Atlanta, Georgia, 2007 Search PubMed.
-
O. Faroon, A. Ashizawa, S. Wright, P. Tucker and K. Jenkins, et al., Toxicological Profile for Cadmium, Agency for Toxic Substances and Disease Registry, Atlanta, Georgia, 2012 Search PubMed.
-
J. Risher and R. DeWoskin, Toxicological profile for mercury, Agency for Toxic Substances and Disease Registry, Atlanta, Georgia, 1999 Search PubMed.
- K. P. Nickens, S. R. Patierno and S. Ceryak, Chromium genotoxicity: a double-edged sword, Chem.-Biol. Interact., 2010, 188, 276–288 CrossRef CAS PubMed.
-
M. Fay, Toxicological profile for nickel, Agency for Toxic Substances and Disease Registry, Atlanta, Georgia, 2005 Search PubMed.
-
M. Williams, G. D. Todd, N. Roney, J. Crawford and C. Coles, et al., Toxicological Profile for Manganese, Agency for Toxic Substances and Disease Registry, Atlanta, Georgia, 2012 Search PubMed.
- W. P. Norwood, U. Borgmann, D. G. Dixon and A. Wallace, Effects of metal mixtures on aquatic biota: a review of observations and methods, Hum. Ecol. Risk Assess., 2003, 9, 795–811 CrossRef CAS.
- M. Heneweer, M. Muusse, M. V. D. Berg and J. T. Sanderson, Additive estrogenic effects of mixtures of frequently used UV filters on pS2-gene transcription in MCF-7 cells, Toxicol. Appl. Pharmacol., 2005, 208, 170–177 CrossRef CAS PubMed.
- A. Kortenkamp, Ten years of mixing cocktails: a review of combination effects of endocrine-disrupting chemicals, Environ. Health Perspect., 2007, 115, 98–105 CrossRef PubMed.
- J. L. Mauderly and J. M. Samet, Is there evidence for synergy among air pollutants in causing health effects, Environ. Health Perspect., 2009, 117, 1–6 CrossRef CAS PubMed.
- X. Lin, Y. Gu and Q. Zhou, Study: joint toxicity of heavy metal mixtures related to proportions of fish consumption, J. Ningbo Univ., 2016, 29, 22–27 Search PubMed.
-
X. Lin, Study on the Joint Toxicity of Common Heavy Metal Pollutants in Aquatic Products, Ningbo University, 2015, p. 77 Search PubMed.
- A. P. Neal and T. R. Guilarte, Mechanisms of lead and manganese neurotoxicity, Toxicol. Res., 2013, 2, 99–114 RSC.
- H. You, Z. Pang, Y. Liang and Y. Ling, Health risk assessment of heavy metals in drinking water in a certain district of Foshan City, South China J. Prev. Med., 2011, 37, 32–36 Search PubMed.
- G. J. Sun, L. Yao, L. Jiao, Y. Shi and Q. Y. Zhang,
et al., Characterizing PM2.5 Pollution of a Subtropical Metropolitan Area in China, Atmos. Clim. Sci., 2013, 3, 100–110 CAS.
- J. R. Bucher, NTP toxicity studies of sodium dichromate dihydrate (CAS No. 7789-12-0) administered in drinking water to male and female F344/N rats and B6C3F1 mice and male BALB/c and am3-C57BL/6 mice, Toxic Rep. Ser., 2007, 72, 1-g4 Search PubMed.
- F. Piao, K. Yokoyama, N. Ma and T. Yamauchi, Subacute toxic effects of zinc on various tissues and organs of rats, Toxicol. Lett., 2003, 145, 28–35 CrossRef CAS PubMed.
- F. De Oliveira, M. Viana, A. Antoniolli and M. Marchioro, Differential effects of lead and zinc on inhibitory avoidance learning in mice, Braz. J. Med. Biol. Res., 2001, 34, 117–120 CrossRef CAS PubMed.
- P. Whanger, Effects of dietary nickel on enzyme activities and mineral contents in rats, Toxicol. Appl. Pharmacol., 1973, 25, 323–331 CrossRef CAS PubMed.
- O. J. Afonne, O. E. Orisakwe, I.-O. A. Ekanem and D. D. Akumka, Zinc protects chromium-induced testicular injury in mice, Indian J. Pharmacol., 2002, 34, 26–31 CAS.
- C. Hebert, NTP technical report on the toxicity studies of Cupric Sulfate (CAS No. 7758-99-8) Administered in Drinking Water and Feed to F344/N Rats and B6C3F1 Mice, Toxic Rep. Ser., 1993, 29, 1-d3 Search PubMed.
-
S. Gad, W. Powers, B. Dunn, G. Hoffman and K. Siino, et al., Acute toxicity of four chromate salts, 1986, pp. 43–58 Search PubMed.
Footnote |
† These authors contributed equally to this work. |
|
This journal is © The Royal Society of Chemistry 2018 |