DOI:
10.1039/C7QO00766C
(Research Article)
Org. Chem. Front., 2018,
5, 24-28
Synthesis of N-sulfenyl-sulfoximines and -sulfenamides through a metal-free N–H/S–H dehydrocoupling reaction†
Received
28th August 2017
, Accepted 17th September 2017
First published on 19th September 2017
Abstract
A metal-free, iodine-catalyzed N–H/S–H dehydrocoupling reaction of sulfoximines and anilines with various thiols to construct sulfur–nitrogen bonds is herein presented. A variety of structurally diverse N-sulfenylsulfoximines and sulfenamides were prepared with up to 97% yield. The reaction was carried out in the presence of hydrogen peroxide in PEG400 under mild conditions.
Sulfur-containing molecules are ubiquitous in nature, possessing great versatility and wide applications in biologically relevant processes.1 Among them, compounds with N–S bonds are some of the most important bioactive skeletons.2 Nevertheless, due to their vulnerability to oxidation and their propensity to form inactive metal-thiolate complexes of sulfur-containing substrates, methods for the effective construction of N–S bonds are scarce. These methods suffer from the usage of toxic metal catalysts, the requirement of a stoichiometric amount of base and harsh reaction conditions.3–6 Therefore, it is highly desirable to develop a facile and green methodology for N–S bond formation.
Sulfoximines are still a rather unrecognized kind of compound, although decades have passed since their discovery.7 People’s interests have mainly focused on their synthetic applications8 until recently, as sulfoximines have emerged as exciting building blocks for new drug discovery.9 Consequently, the development of new and environmentally friendly synthetic approaches for sulfoximines is of great importance. Particularly, due to their usefulness in agrochemicals and drug development, N-substituted sulfoximine derivatives, for instance N-arylsulfoximines10 and 1,2-benzothiazines,11 have attracted continuous attention.3k,12 However, N-sulfenylation has rarely been reported. Conventional synthetic routes (Scheme 1, path a) are limited in application due to the usage of hazardous chloride and redundant synthetic steps.13 In 2016, the copper-catalyzed N-sulfenylation of sulfoximines was developed by Cheng and Yu using disulfides as the sulfur source (Scheme 1, path b).3k The transition metal catalyst, sodium acetate base and environmentally unfriendly solvent make this protocol less environmentally benign. It is worth noting that the generation of the sulfoximine anion is crucial in these two processes. Bolm et al. reported the synthesis of N-trifluoromethylthio sulfoximines via N-Br derivatives (Scheme 1, path c),12e which is a much less practical procedure.
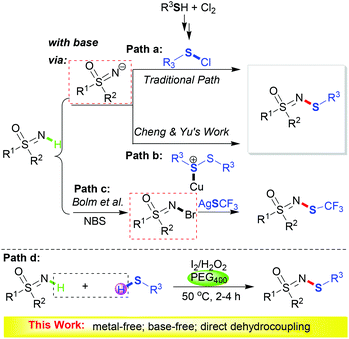 |
| Scheme 1 Comparison of previous work and this work. | |
Iodide/oxidant-assisted oxidative coupling transformations have continuously attracted attention due to their high efficiency and environmentally benign features.14 In addition, the use of thiols as thiolating reagents is a straightforward and high atom economy method for sulfenylation. It is well known that polyethylene glycol (PEG), as a nonhazardous alternative to the currently used organic solvents, has a number of benign characteristics.15
Inspired by these aspects and our continuous interest in metal-free processes16 and organosulfur chemistry,17 we wish to develop an iodide-catalyzed pathway to realize the N-sulfenylation of sulfoximine (Scheme 1, path d). As far as we know, this is the first example of a direct intermolecular dehydrocoupling reaction to construct N-sulfenylsulfoximines.
After systematic optimization of the reaction conditions, the standard conditions were set as 1a (1 equiv.), 2a (2 equiv.), I2 (10 mol%) and H2O2 (2.5 equiv.) in PEG400 at 50 °C (see the ESI, Table 1†).
With these optimized reaction conditions in hand, the scope of various sulfoximines was investigated, as shown in Scheme 2. In general, the N–H/S–H dehydrocoupling reactions of sulfoximines and benzenethiol went well, providing N-sulfenylated sulfoximines in excellent yields (83–97%). No obvious electronic effect was observed in view of the yields of S,S-diarylsulfoximines (3aa–3ca) and S-aryl-S-alkyl sulfoximines (3da–3ga). Moreover, the stereoelectronic effects of the S-alkyl substituent of S-alkyl-S-phenyl sulfoximines show little relevance (3da and 3ha–3ja). Notably, S-cyclopropyl-S-phenyl sulfoximine was also efficiently N-sulfenylated to produce 3ja with 90% yield, whose skeleton exists in bioactive compounds such as BAY 1000394.9a,b
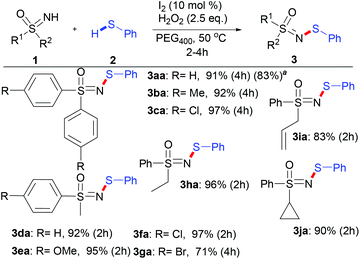 |
| Scheme 2 The N–H/S–H dehydrocoupling reaction of thiophenol and various sulfoximines. Reaction conditions: 1 (0.1 mmol), 2 (0.2 mmol, 2 equiv.), I2 (10 mol%) and H2O2 (30% in water, 2.5 equiv.) in PEG400 (1.5 mL) at 50 °C (oil bath) for 2–4 h in air. Isolated yields are shown. a The scaled-up reaction was performed using 5 mmol sulfoximine (1.086 g), 0.5 mol% I2 and 2 equiv. H2O2 in 15 mL PEG400 at 50 °C for 12 h to afford 1.351 g of 3aa (yield: 83%). | |
Various thiols were suitable for the N–H/S–H dehydrocoupling reaction, and a wide range of N-sulfenylsulfoximines were accessible in moderate to excellent yields (Scheme 3).
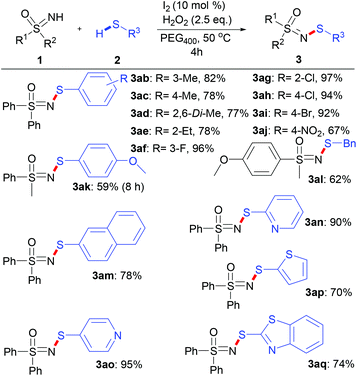 |
| Scheme 3 The N–H/S–H dehydrocoupling reaction of various thiols. Reaction conditions: 1 (0.1 mmol), 2 (0.2 mmol, 2 eq.), I2 (10 mol%) and H2O2 (30% in water, 2.5 equiv.) in PEG400 (1.5 mL) at 50 °C (oil bath) for 4 h in air. Isolated yields are shown. | |
The reactions of methyl- and ethyl-substituted thiols with S,S-diphenylsulfoximines led to products 3ab–3ae in yields ranging from 77% to 82%. The thiol with a para-methoxy substituent reacted with S-phenyl-S-methyl sulfoximine to give 3ak in 59% yield. Substrates bearing an electron-withdrawing substituent on the phenyl group, such as a halogen (F, Cl, Br) (3af–3ai), were relatively more reactive. However, an excessively strong electron-withdrawing group, such as the 4-nitro group, results in a lower yield (3aj, 67%). Notably, the reactions with benzyl mercaptan (3al) and 2-naphthalenthiol (3am) also proceeded smoothly under these reaction conditions to give the corresponding products with yields of 62% and 78%, respectively. Pleasingly, reactions with heterocyclic (pyridyl, thienyl and benzothiazolyl) thiols proceeded fairly well under the optimized conditions (3an–3aq), and the yields of the corresponding products ranged from 70% to 95%, highlighting the efficiency of this synthetic methodology.
To verify the practicality of our newly developed procedure, a reaction was conducted on the gram scale (5 mmol) using 1a as the substrate (Scheme 1). Gratifyingly, a similar yield of 83% compared to that of the 0.1 mmol scale (91%) was obtained, even under the conditions of lower catalyst loading (0.5 mol%) and a lower H2O2 amount (2 equiv.).
Next, in order to demonstrate the generality of this methodology, anilines were introduced to react with thiols, as shown in Scheme 4. To our satisfaction, anilines with methyl, bromo, ester, nitro, trifluoromethyl and cyano groups all reacted well with thiophenol, producing the desired sulfenamides in moderate yields (5aa–5ag). In addition, N-methyl substituted aniline also gave the target product in a yield of 62% (5ah).
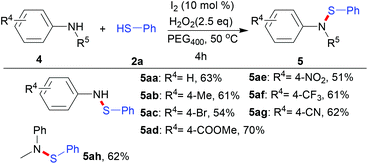 |
| Scheme 4 The N–H/S–H dehydrocoupling reaction of thiophenol and various anilines. Reaction conditions: 4 (0.1 mmol), 2a (0.2 mmol, 2 equiv.), I2 (10 mol%) and H2O2 (30% in water, 2.5 equiv.) in PEG400 (1.5 mL) at 50 °C (oil bath) for 4 h in air. Isolated yields are shown. | |
To gain more information on the reaction principles of this catalytic process, mechanistic studies were performed (Scheme 5). As the thiol is readily converted into the disulfide under our experimental conditions,18 diphenyl disulfide was subjected to the standard conditions, which led to 3aa in a yield of 89% (Scheme 5, path a). This indicates that the disulfide might be the intermediate which leads to the target product.
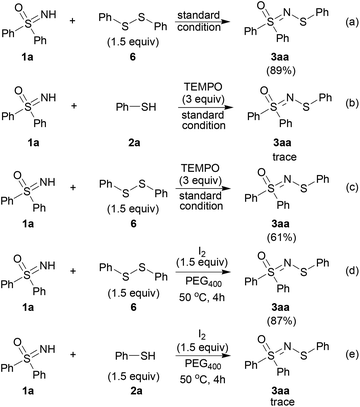 |
| Scheme 5 Mechanistic studies. | |
When a radical scavenger (2,2,6,6-tertramethylpiperidine N-oxide, TEMPO) was added to the reaction mixture, the reaction process was suppressed remarkably (Scheme 5, path b). However, when diphenyl disulfide was used as the substrate, the desired product was obtained with moderate yield (Scheme 5, path c). This suggests that the radical scavenger only inhibited the formation of the disulfide intermediate, and the pathway from the disulfide to the product was not affected. Moreover, diphenyl disulfide in the presence of a stoichiometric amount of iodine reacted with 1a to give the target compound (Scheme 5, path d). On the contrary, the result from using thiophenol instead of diphenyl disulfide was far from satisfactory (Scheme 5, path c).
Based on the literature reports19 and our preliminary experimental results, we proposed a plausible mechanism (Scheme 6). Initially, the thiol reacts to give the disulfide intermediate. Simultaneously, the disulfide reacts with I2 to form the active electrophilic species, R–S–I. 1 acts as a nucleophile to attack R–S–I which produces 7, which then undergoes deprotonation to generate the desired product, 3. Hydriodic acid is oxidized by H2O2 to restore the I2 catalyst, and water is the sole by-product.
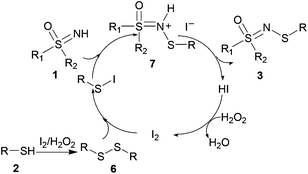 |
| Scheme 6 Proposed mechanism. | |
Conclusions
In summary, an unprecedented iodine-catalyzed intermolecular S–H/N–H dehydrocoupling reaction of sulfoximines or anilines with thiols has been accomplished. This N-sulfenylation process provides a simple and effective strategy to synthesize N-sulfenylsulfoximines and sulfenamides. This methodology is characterized by its utilization of a non-toxic reaction medium, high atom economy and wide functional group tolerance.
Conflicts of interest
There are no conflicts to declare.
Acknowledgements
This study was funded by the National Natural Science Foundation of China (Grant No. 21372034), the Department of Science and Technology of Sichuan Province (Grant No. 2016HH0074), Chengdu Science and Technology Bureau (Grant No. 2015-HM01-00362-SF) and the State Key Laboratory of Geohazard Prevention and Geoenvironment Protection Independent Research Project (Grant No. SKLGP2016Z004).
Notes and references
-
(a) Y. G. Wang, S. Chackalamannil, W. Chang, W. Greenlee, V. Ruperto, R. A. Duffy and R. McQuade, Bioorg. Med. Chem. Lett., 2001, 11, 891 CrossRef CAS PubMed;
(b) A. Gangjee, Y. B. Zeng, T. Talreja, J. J. McGuire, R. L. Kisliuk and S. F. Queener, J. Med. Chem., 2007, 50, 3046 CrossRef CAS PubMed;
(c) M. Bozdag, M. Pinard, F. Carta, E. Masini, A. Scozzafava, R. McKenna and C. T. Supuran, J. Med. Chem., 2014, 57, 9673 CrossRef CAS PubMed;
(d) R. F. Shang, X. Y. Pu, X. M. Xu, Z. J. Xin, C. Zhang, W. Z. Guo, Y. Liu and J. P. Liang, J. Med. Chem., 2014, 57, 5664 CrossRef CAS PubMed;
(e) E. A. Ilardi, E. Vitaku and J. T. Njardarson, J. Med. Chem., 2013, 57, 2832 CrossRef PubMed.
-
(a) A. Marble, Drugs, 1971, 1, 109 CrossRef CAS PubMed;
(b) J. D. Fischer, M. H. Song, A. B. Suttle, W. D. Heizer, C. B. Burns, D. L. Vargo and K. L. R. Brouwer, Pharm. Res., 2000, 17, 154 CrossRef CAS PubMed;
(c) H. Sugimoto, M. Shichijo, T. Iino, Y. Manabe, A. Watanabe, M. Shimazaki, F. Gantner and K. B. Bacon, J. Pharmacol. Exp. Ther., 2003, 305, 347 CrossRef CAS PubMed.
- Selected examples for the Cu-assisted N–S bond construction:
(a) V. Nair, S. M. Nair, S. Devipriya and D. Sethumadhavan, Tetrahedron Lett., 2006, 47, 1109 CrossRef CAS;
(b) N. Taniguchi, Synlett, 2007, 1917 CrossRef CAS;
(c) N. Taniguchi, Eur. J. Org. Chem., 2010, 2670 CrossRef CAS;
(d) B. S. Bhakuni, S. J. Balkrishna, A. Kumar and S. Kumar, Tetrahedron Lett., 2012, 53, 1354 CrossRef CAS;
(e) F. Wang, C. Chen, G. Deng and C. J. Xi, J. Org. Chem., 2012, 77, 4148 CrossRef CAS PubMed;
(f) Z. Wang, Y. Kuninobu and M. Kanai, J. Org. Chem., 2013, 78, 7337 CrossRef CAS PubMed;
(g) X. Huang, J. C. Wang, Z. Q. Ni, S. C. Wang and Y. J. Pan, Chem. Commun., 2014, 50, 4582 RSC;
(h) C. Lee, Y. N. Lim and H. Y. Jang, Eur. J. Org. Chem., 2015, 5934 CrossRef CAS;
(i) C. Lee, X. Wang and H. Y. Jang, Org. Lett., 2015, 17, 1130 CrossRef CAS PubMed;
(j) N. Taniguchi, Eur. J. Org. Chem., 2016, 2157 CrossRef CAS;
(k) H. Zhu, J. T. Yu and J. Cheng, Chem. Commun., 2016, 52, 11908 RSC.
- AgNO3-promoted N–S bond construction: P. Gogoi, S. R. Gogoi, M. Kalita and P. Barman, Synlett, 2013, 873 CAS.
- (Diacetoxyiodo) benzene-mediated N–S bond construction: E. Rattanangkool, W. Krailat, T. Vilaivan, P. Phuwapraisirisan, M. Sukwattanasinitt and S. Wacharasindhu, Eur. J. Org. Chem., 2014, 4795 CrossRef CAS.
- Co-catalyzed N–S bond formation: Y. Dou, X. Huang, H. Wang, L. Yang, H. Li, B. Yuan and G. Yang, Green Chem., 2017, 19, 2491 RSC.
- H. R. Bentley, E. E. Mcdermott, J. Pace, J. K. Whitehead and T. Moran, Nature, 1950, 165, 150 CrossRef CAS PubMed.
-
(a) C. R. Johnson, M. R. Barbachyn, N. A. Meanwell, C. J. Stark and J. R. Zeller, Phosphorus Sulfur Relat. Elem., 1985, 24, 531–543 CAS;
(b) M. Reggelin and C. Zur, Synthesis, 2000, 1 CrossRef CAS;
(c) H. Okamura and C. Bolm, Chem. Lett., 2004, 33, 482 CrossRef CAS;
(d) H. J. Gais, Heteroat. Chem., 2007, 18, 472 CrossRef CAS;
(e) V. Bizet, C. M. M. Hendriks and C. Bolm, Chem. Soc. Rev., 2015, 44, 3378 RSC.
-
(a) G. Siemeister, U. Lucking, A. M. Wengner, P. Lienau, W. Steinke, C. Schatz, D. Mumberg and K. Ziegelbauer, Mol. Cancer Ther., 2012, 11, 2265 CrossRef CAS PubMed;
(b) U. Lucking, R. Jautelat, M. Kruger, T. Brumby, P. Lienau, M. Schafer, H. Briem, J. Schulze, A. Hillisch, A. Reichel, A. M. Wengner and G. Siemeister, ChemMedChem, 2013, 8, 1067 CrossRef PubMed;
(c) B. Gutmann, P. Elsner, A. O'Kearney-McMullan, W. Goundry, D. M. Roberge and C. O. Kappe, Org. Process Res. Dev., 2015, 19, 1062 CrossRef CAS;
(d) F. P. Vendetti, A. Lau, S. Schamus, T. P. Conrads, M. J. O'Connor and C. J. Bakkenist, Oncotarget, 2015, 6, 44289 Search PubMed;
(e) N. Nishimura, M. H. Norman, L. B. Liu, K. C. Yang, K. S. Ashton, M. D. Bartberger, S. Chmait, J. Chen, R. Cupples, C. Fotsch, J. Helmering, S. R. Jordan, R. K. Kunz, L. D. Pennington, S. F. Poon, A. Siegmund, G. Sivits, D. J. Lloyd, C. Hale and D. J. St Jean, J. Med. Chem., 2014, 57, 3094 CrossRef CAS PubMed;
(f) U. Lücking, Angew. Chem., Int. Ed., 2013, 52, 9399 CrossRef PubMed;
(g) M. Frings, C. Bolm, A. Blum and C. Gnamm, Eur. J. Med. Chem., 2017, 126, 225 CrossRef CAS PubMed.
- M. Miyasaka, K. Hirano, T. Satoh, R. Kowalczyk, C. Bolm and M. Miura, Org. Lett., 2011, 13, 359 CrossRef CAS PubMed.
-
(a) M. Harmata, K. O. Rayanil, M. G. Gomes, P. Zheng, N. L. Calkins, S. Y. Kim, Y. Fan, V. Bumbu, D. R. Lee, S. Wacharasindhu and X. Hong, Org. Lett., 2005, 7, 143 CrossRef CAS PubMed;
(b) A. Garimallaprabhakaran, X. Hong and M. Harmata, ARKIVOC, 2012, 119 CAS;
(c) W. Dong, L. Wang, K. Parthasarathy, F. F. Pan and C. Bolm, Angew. Chem., Int. Ed., 2013, 52, 11573 CrossRef CAS PubMed;
(d) Y. Cheng and C. Bolm, Angew. Chem., Int. Ed., 2015, 42, 12526 CrossRef;
(e) W. H. Jeon, J. Y. Son, J. E. Kim and P. H. Lee, Org. Lett., 2016, 18, 3498 CrossRef CAS PubMed;
(f) J. Wen, D. P. Tiwari and C. Bolm, Org. Lett., 2017, 19, 1706 CrossRef CAS PubMed.
-
(a) R. Kowalczyk, A.
J. F. Edmunds, R. G. Hall and C. Bolm, Org. Lett., 2011, 13, 768 CrossRef CAS PubMed;
(b) L. Wang, H. Huang, D. L. Priebbenow, F. F. Pan and C. Bolm, Angew. Chem., Int. Ed., 2013, 52, 3478 CrossRef CAS PubMed;
(c) C. M. M. Hendriks, R. A. Bohmann, M. Bohlem and C. Bolm, Adv. Synth. Catal., 2014, 356, 1847 CrossRef CAS;
(d) L. Wang, D. L. Priebbenow, W. R. Dong and C. Bolm, Org. Lett., 2014, 16, 2661 CrossRef CAS PubMed;
(e) C. Bohnen and C. Bolm, Org. Lett., 2015, 17, 3011 CrossRef CAS PubMed;
(f) R. K. Chinnagolla, A. Vijeta and M. Jeganmohan, Chem. Commun., 2015, 51, 12992 RSC;
(g) H. B. Peng, J. T. Yu, W. J. Bao, J. W. Xu and J. Cheng, Org. Biomol. Chem., 2015, 13, 10600 RSC;
(h) F. Teng, J. Cheng and J. T. Yu, Org. Biomol. Chem., 2015, 13, 9934 RSC;
(i) F. Teng, S. Sun, Y. Jiang, J. T. Yu and J. Cheng, Chem. Commun., 2015, 51, 5902 RSC;
(j) Y. Zou, J. Xiao, Z. H. Peng, W. R. Dong and D. L. An, Chem. Commun., 2015, 51, 14889 RSC;
(k) H. C. Cheng and C. Bolm, Synlett, 2016, 769 CAS;
(l) N. Sharma and G. Sekar, RSC Adv., 2016, 6, 37226 RSC;
(m) H. Zhu, F. Teng, C. D. Pan, J. Cheng and J. T. Yu, Tetrahedron Lett., 2016, 57, 2372 CrossRef CAS.
-
(a) H. C. Buchholt, Org. Prep. Proced., 1970, 2, 177 CAS;
(b) K. Akutagawa, N. Furukawa and S. Oae, Bull. Chem. Soc. Jpn., 1984, 57, 518 CrossRef CAS.
- For recent examples, see:
(a) U. Kloeckner, P. Finkbeiner and B. J. Nachtsheim, J. Org. Chem., 2013, 78, 2751 CrossRef CAS PubMed;
(b) J. Zhang, J. W. Jiang, Y. L. Li and X. B. Wan, J. Org. Chem., 2013, 78, 11366 CrossRef CAS PubMed;
(c) H. Yu and J. Shen, Org. Lett., 2014, 16, 3204 CrossRef CAS PubMed;
(d) H. Yu, F. L. Zhang and W. H. Huang, Synlett, 2014, 843 CrossRef CAS;
(e) Y. C. Yuan, W. J. Hou, D. Zhang-Negrerie, K. Zhao and Y. F. Du, Org. Lett., 2014, 16, 5410 CrossRef CAS PubMed;
(f) M. A. Hiebel and S. Berteina-Raboin, Green Chem., 2015, 17, 937 RSC;
(g) B. Mondal, S. C. Sahoo and S. C. Pan, Eur. J. Org. Chem., 2015, 3135 CrossRef CAS;
(h) G. C. Senadi, W. P. Hu, T. Y. Lu, A. M. Garkhedkar, J. K. Vandavasi and J. J. Wang, Org. Lett., 2015, 17, 1521 CrossRef CAS PubMed;
(i) H. Yu and J. Shen, RSC Adv., 2015, 5, 9815 RSC;
(j) Y. Siddaraju and K. R. Prabhu, J. Org. Chem., 2016, 81, 7838 CrossRef CAS PubMed;
(k) S. L. Yi, M. C. Li, W. M. Mo, X. Q. Hu, B. X. Hu, N. Sun, L. Q. Jin and Z. L. Shen, Tetrahedron Lett., 2016, 57, 1912 CrossRef CAS.
-
(a) J. Chen, S. K. Spear, J. G. Huddleston and R. D. Rogers, Green Chem., 2005, 7, 64 RSC;
(b) M. J. D. Pires, S. I. Purificação, A. S. Santos and M. M. B. Marques, Synthesis, 2017, 2337 CAS;
(c) M.-A. Hiebel and S. Berteina-Raboin, Green Chem., 2015, 17, 937 RSC;
(d) B. Vaddula, J. Leazer and R. S. Varma, Adv. Synth. Catal., 2012, 354, 986 CrossRef CAS.
-
(a) L. Yang and Q. Zeng, Synthesis, 2017, 3149 CAS;
(b) Y. Zhou, X. Z. Tu, Z. J. Li, L. H. Zhou and Q. L. Zeng, Org. Prep. Proced. Int., 2016, 48, 413 CrossRef CAS;
(c) H. Wei, L. Zhou, Y. Zhou and Q. Zeng, Toxicol. Environ. Chem., 2015, 97, 2 CrossRef CAS.
-
(a) C. Dai, X. F. Sun, X. Z. Tu, L. Wu, D. Zhan and Q. L. Zeng, Chem. Commun., 2012, 48, 5367 RSC;
(b) Q. Wen, L. Zhang, J. Xiong and Q. Zeng, Eur. J. Org. Chem., 2016, 5360 CrossRef CAS;
(c) Q. Zeng, H. Wang, T. Wang, Y. Cai, W. Weng and Y. Zhao, Adv. Synth. Catal., 2005, 347, 1933 CrossRef CAS.
-
(a) K. A. Mazzio, K. Okamoto, Z. Li, S. Gutmann, E. Strein, D. S. Ginger, R. Schlaf and C. K. Luscombe, Chem. Commun., 2013, 49, 1321 RSC;
(b) T. Nabeshima, D. Nishida and T. Saiki, Tetrahedron, 2003, 59, 639 CrossRef CAS;
(c) A. Spurg, G. Schnakenburg and S. R. Waldvogel, Chem. – Eur. J., 2009, 15, 13313 CrossRef CAS PubMed;
(d) F. S. Teplyakov, T. G. Vasileva, M. L. Petrov and D. A. Androsov, Org. Lett., 2013, 15, 4038 CrossRef CAS PubMed.
-
(a) Y. Z. Yan, Y. H. Zhang, C. T. Feng, Z. G. Zha and Z. Y. Wang, Angew. Chem., Int. Ed., 2012, 51, 8077 CrossRef CAS PubMed;
(b) T. Nobuta, N. Tada, A. Fujiya, A. Kariya, T. Miura and A. Itoh, Org. Lett., 2013, 15, 574 CrossRef CAS PubMed;
(c) D. S. Yang, K. L. Yan, W. Wei, J. Zhao, M. Q. Zhang, X. G. Sheng, G. Q. Li, S. L. Lu and H. Wang, J. Org. Chem., 2015, 80, 6083 CrossRef CAS PubMed;
(d) S. K. R. Parumala and R. K. Peddinti, Tetrahedron Lett., 2016, 57, 1232 CrossRef CAS;
(e) Saima, D. Equbal, A. G. Lavekar and A. K. Sinha, Org. Biomol. Chem., 2016, 14, 6111–6118 RSC;
(f) A. Gupta, M. S. Deshmukh and N. Jain, J. Org. Chem., 2017, 82, 4784 CrossRef CAS PubMed.
Footnote |
† Electronic supplementary information (ESI) available. See DOI: 10.1039/C7QO00766C |
|
This journal is © the Partner Organisations 2018 |