DOI:
10.1039/C7IB00178A
(Technical Innovation)
Integr. Biol., 2018,
10, 67-73
3D-Printed seed planter and well array for high-throughput seed germination screening†
Received
13th October 2017
, Accepted 30th November 2017
First published on 1st December 2017
Abstract
Seed germination is an important means of evaluating seed quality. In the present study, a well array for a seed germination experiment was designed and fabricated by 3D printing for the first time. Each hollow cone-shaped well can hold one seed, which not only prevented the seed from falling out of the well but also ensured that part of the seed was fully exposed to the sublayer of wet filter paper, allowing it to receive water for germination. Coupled with a 3D-printed seed planter, single seeds can be quickly placed into arrayed wells. Moreover, the number of sprouts could be automatically obtained from image analysis, which greatly improved the efficiency of the entire experiment. In summary, the high-throughput, easy-to-use seed germination well array that we have developed has been shown to have potential applications in botanical seed research and agricultural production.
Insight, innovation, integration
Seeds are the key to agricultural production, and high-quality seeds are the basis of all agricultural production. Seed germination is an important means of evaluating seed quality. A germination array and seed planter were designed and fabricated by 3D printing. Simply rotating the crank of the seed planter can easily deliver individual seeds into the arrayed wells. The hollow cone-shaped wells can hold one seed, which not only prevented the seed from falling out of the well but also ensured that part of the seed was fully exposed to the sublayer of wet filter paper, allowing it to receive water for germination. The 3D printed apparatus is well-suited for in-field studies of seed quality and screening of conditions for seedlings.
|
1. Introduction
Seeds are the key to agricultural production, and high-quality seeds are the basis of all agricultural production. Understanding the factors that affect seed vigor and its mechanism of change as well as the accurate and timely determination of seed vigor is of great value in guiding the safe storage of seeds and their use in agricultural production.1 In general, seed vigor can be tested by determining the germination percentage of seeds, which refers to the percentage of normal seedlings that grow within a specified condition and time relative to the number of total seeds to be inspected.2 The germination test is an important part of scientific research in the areas of seed biology, seed quality management, seed improvement, and seed quality standardization.3 For preservation of germplasm resources, germination percentage is often used in the identification of certain germplasms related to nutrient elements and harmful elements in monitoring seed viability.4,5 Seed germination is also often used for phenotypic evaluation. Finally, the accurate measurement of germination percentage provides a basis for determining the amount of seeds to sow, allowing for higher yields.
At present, germination paper (towel paper, filter paper, and blotters), sand and solid agarose medium are the basic supplies required for germination tests. The germination test normally requires a container such as a plastic bag or Petri dish and a germination bed consisting of a paper bed and a sand bed. In most laboratories, a paper toweling method (roll-towel method) is most commonly used for medium-sized and bold seeds. In principle, seeds are placed in rows on a moistened paper towel, which is spread on a flat surface. After the required germination period, the towels are removed from the container and carefully unwrapped so that the fragile sprouts are not destroyed.6 The difficult part of this method is that the paper should be changed during the germination period. The handling of the seeds, especially placing them at the same positon on the substrate, is tedious and time-consuming. Modern botany requires rapid and convenient methods to determine and quantify seed germination. In rural areas, especially on farms, a seed germination screening method that is easy to operate and has a high-throughput is needed for large-scale germination determination or trials.
An important strategy for high-throughput analysis is the construction of a microarray reaction platform; that is, producing high-density reaction units on a limited area. A microwell array is an innovative method for single cell culture and screening.7 The key point of using a microarray for high-throughput single cell analysis is to fabricate a high density well or chamber that can seed only one cell.8,9 Normally, soft lithography is applied to fabricated high-density microwell arrays. The rate of seeding for an individual single cell can be improved by optimizing the size and shape of the microwell or chamber. Inspired by microarrays used in single cell analysis, we have envisioned an array-based experimental platform for seed germination. The microarray wells used in cell research are mostly made from polydimethylsiloxane (PDMS) due to its high precision in microfabrication, and the dimensions of the well or chamber range from 20–80 μm. Very recently, Park et al. reported an array chip for plant study.10 In their work, a PDMS well array was replicated from a polycarbonate mold which was produced by micro-milling techniques. Then two structured PDMS layers were assembled by a PDMS precursor. Individual seeds were plated into the array chip, which was filled with solid agarose medium. The seed germination can be clearly observed under a stereoscope. The plant chip enables the tracking of an individual seed in a spatially partitioned array. However, the chip is designed for the tracking of an individual seed in solid medium blocks which can establish various chemicals and other environmental conditions for seed growth, while paper-bed based germination assays that are routinely conducted in laboratories have not been investigated. Although both solid agarose medium-based germination assays and paper-bed based germination focus on observing a cotyledon emerging and sprout growth, their experimental conditions are different. First, sterilization of both the agarose medium, container and seeds is required for agarose-based assays. Next, the nutrition, pH and salt conditions would change because the germination assay normally needs 3–5 days, sometimes even over 10 days. Replacing the paper-bed to ensure a stable environment is much easier than changing the solid agarose medium bed. Because of their simplicity, paper-bed germination assays are suitable for fast screening of germination under different environments.
Therefore, for the first time, an array chip was constructed for paper-bed germination assays. Three dimensional (3D) printing is a processing method that can be used to produce new micro devices. It is high speed and low cost, consumes little energy, and is easy to operate.11–13 Thus, with the aim of having a simple structure, a convenient mode of operation, a wide range of applications, and high accuracy, germination apparatus was designed and 3D printed. Moreover, because these cell arrays are arranged in order, they can be combined with image processing to improve the screening rate of seed germination percentage. Therefore, the seed germination 3D cell array developed here could be applied to study environmental stress effects on seed germination. It could be used for rapid and early prediction of environmental threats with high throughput.
2. Experimental
2.1 Reagents
Photosensitive 3D printing resin (SP-DR250) and a 3D printer (MoonRay UV DLP) were purchased from SprintRay Inc. USA. Three types of seeds, namely Sainfoin, Seasonal cabbage, and Liuye amaranth, were obtained from Ding Xi Seed Institute, China. All other chemicals were purchased from Aladdin Chemical Reagent Co, Ltd., China. Chemicals were used as received and prepared in ultrapure water (18 MΩ cm−1, PURELAB flex system, ELGA Corporation) without further purification.
2.2 Design and 3D printing of the germination well array and seed planter
The seed germination well array (3D germination array) consists of three parts: a square box, a piece of filter paper, and a square tray (Fig. 1A). The square box, which is the upper part, comprises 100 independent chambers in 10 × 10 rows. In each chamber, there is an inverted hollow cone-shape germination well. The square box fits into the bottom tray in which filter paper can be placed. The seed planter consists of two parts: a storage box and a seeding system (Fig. 1B). The storage box has a lid, which is mainly used for storing the seeds that need to be placed into the well-array. There are holes on the bottom of the storage box. The diameter of the entrance hole is slightly larger than that of the target seed, and thus only one seed can enter the seeding system (Table 1). The seeding system includes a rotating crank, which contains 10 seed delivering units. Each unit has three blades circling around the crank axis. The rotation of the crank can change the position of the blade, thus controlling the entrance and exit of the seed from the delivery unit. By simply rotating the crank, 10 seeds can be easily placed into the arrayed wells. The coupling of the germination array and the seed planter can facilitate the placement of single seeds in a high-throughput manner (Fig. 1C).
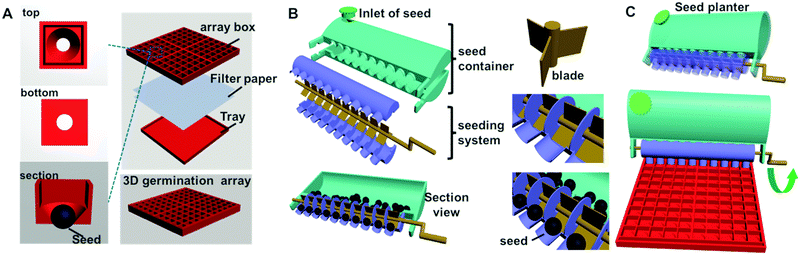 |
| Fig. 1 3D printing to fabricate the germination array and seed planter. (A) Structure of the germination array: individual hollow cone-shape well (left); parts of the device: array box and tray; (B) parts of the seed planter: 10 three-blade units were arranged on a single crank; the spacer between the three-blade unit guides the single seed inoculation into each array well; (C) rotating the crank can deliver 10 seeds into 10 wells at once. | |
Table 1 Dimensions of the array chip for seeds with different sizes
Diameter |
Array chip for |
Sainfoin seed (mm) |
Seasonal cabbage seed (mm) |
Liuye amaranth seed (mm) |
Seed |
5.1 |
3.3 |
2.25 |
Top of the well |
6.0 |
4.5 |
3.0 |
Bottom of the well |
3.0 |
2.25 |
1.5 |
Entrance hole of the seed planter |
5.5 |
3.7 |
2.65 |
The germination array and seed planter were printed using a MoonRay 3D printer (SprintRay Inc. USA). According to the information provided by the manufacturer, the printing resolution of the MoonRay 3D printer is 20 μm in the vertical direction, and 100 μm in the horizontal direction. The finest printing model (20 μm vertical layer) was applied. First, autodesk 3Ds MAX software (Autodesk Media and Entertainment, USA) was used for designing and drawing. Then, the image was converted into STL file format, sliced, and preserved into sequence layers of two-dimensional (2D) horizontal cross-sections before printing. As the image of each layer was transmitted to the printer, the liquid photosensitive resin in the printer storage tank solidified into a thin layer under irradiation at a wavelength of 405 nm. As the printer moved to the next layer in the vertical direction, another solidified layer was printed out and covered the previous cured layer. At the end of printing, the printed object was exposed to irradiation at 405 nm to sufficiently cure the photosensitive resin. Finally, the array box and tray were thoroughly washed with ethanol.
2.3 Germination test of the 3D-array box
The seed germination test was conducted in the 3D arrays. First, moistened filter paper was placed flat in the tray. Then, the array box was placed on the paper prior to placement of the seeds. If the filter paper needed to be replaced during the experiment, the only action needed was to lift the upper array box and change the paper. Model seeds with different sizes, namely Sainfoin, Seasonal cabbage, and Liuye amaranth, were studied as model seeds for the germination experiment (Table 1). After being soaked in warm water at 40 °C for four hours, selected plump seeds were disinfected with 10% sodium hypochlorite for 10 minutes and then washed three times with distilled water. Then, the seeds were fed into the 3D-printed seed planter. By rolling the crank of the planter, seeds can be delivered into each well row-by-row. The seed germination experiment was carried out on a Petri dish as a comparison group. In brief, the moistened filter paper was placed in a 100 mm diameter glass Petri dish. Then, a total of 100 seeds were evenly placed on the filter paper. All seed germination was conducted in an artificial climate incubator (LAC-250HPY-2, Shanghai Long Yue Instrument Inc. China). The filter paper was replaced every two days, and the number of seeds that germinated was counted. If replacement filter paper was required during the experiment for the 3D-array box, the only action that was needed was to lift the upper boxes and change the paper in the square tray, whereas the seeds need to be transferred to a new filter paper and resituated for Petri dish-based paper-beds. All experiments were carried out in triplicate.
2.4 Image analysis
Image analysis software, Image Pro plus (version IPP6.0, MediaCybernetics, USA), was applied to count the seed germination. Specifically, a particle counting and measurement function was utilized to analyze the image. According to the software protocol, the germination picture taken by Nikon D50 (Nikon, Japan) was converted from a Red-Green-Blue (RGB) model to a Hue-Saturation-Intensity (HIS) model. The sprout can be segmented from the whole seed by adjusting the threshold of HIS factors. Moreover, the area of the sprout was defined as 50 to eliminate irrelevant background. The number of sprouts can then be automatically extracted by the software. At the same time, the seed germination picture was manually counted by a researcher who was blinded to the experiment setting.
2.5 Application of the 3D-seed array for studying the influence of salt stress on seed germination
To achieve a high-throughput investigation of salt stress on seed germination, four 3D-germination arrays that consisted of 10 × 10 rows of boxes were combined to form a large platform of 400 total wells. The Seasonal cabbage seeds with diameters of 3.30 mm were used as the model seeds. The effects of salt stress at NaCl concentrations of 0.1, 0.2, 0.3, and 0.4 mol L−1 were studied. In brief, seeds were soaked in a series of treatment solutions for 4 h, and then delivered into the 3D-germination array by the planter. Then, the 3D-germination arrays containing the seeds were cultured in an artificial climate incubator with a temperature of 26 °C, a relative humidity of 75%, and an 8 h light/16 h dark cycle per day. The moistened filter paper was changed every day to maintain sufficient amounts of water for the seeds. The seeds were examined every 12 h for signs of germination, and the number of germinated seeds was photographed and counted. The germination percentage (GP) and relative GP were calculated as follows: | GP = (number of strongly germinating seeds/total number of seeds tested) × 100% | (1) |
| Relative GP = (GP of salt treated-seed/GP of control seed) × 100% | (2) |
2.6 Statistic analysis
All experiments were carried out in triplicate. The results were presented as mean ± the standard deviation. Each of the experimental values was compared to its corresponding control. The data were analyzed by Student's t-test using Origin Statistic software (OriginLab Corporation, USA). A p-value <0.05 was considered significant.
3. Results and discussion
3.1 The 3D-germination array box and seed planter improves the efficiency and throughput of the seed germination test
Three dimensional seed germination array boxes of different sizes were designed according to the sizes of the seeds. In the present study, three types of seeds were selected as models: Sainfoin, Seasonal cabbage, and Liuye amaranth. The diameters of these seeds after pre-soaking were 5.10, 3.30, and 2.25 mm, respectively. The germination arrays and seed planters were designed and printed according to the sizes of the three types of seeds (Table 1). In order to deliver only one seed in each germination well and ensure that part of the seed had sufficient contact with the moistened filter paper, which was needed for bud germination, an inverted hollow cone-shape germination well was designed. The diameter of the top side was 6.0, 4.5, and 3.0 mm, while the diameter of the bottom side was 3.00, 2.25, and 1.50 mm for the three types of seeds, respectively (Fig. 2A and Table 1). Because of the difference of the individual well, the size of the array chip for three types of seed, Sainfoin, Seasonal cabbage, and Liuye amaranth, are 6.8, 5.1, and 3.4 cm, respectively. The upper hole of the cone well is used for seed inclusion, and the small hole at the bottom well prevents the seed from slipping out. At the same time, the seed was able to be in contact with the lower filter paper via the hole at the bottom (Fig. 2B, bottom and side view). The area of the seed that can direct contact with the moistened paper is determined by the size of the hole at the bottom (Fig. 2B, side view of the array box with seeds). The hollow cone-shape well also facilities changing of the moistened filter paper without moving the individual seed. If replacement filter paper was required during the experiment, the only action that was needed was to lift the upper boxes and change the paper in the square tray. The seeds in the array chip will not be moved individually. Moreover, because the relative position of the seeds within the array-box will not change during the entire germination test, precise tracking of the budding process of a single seed can be achieved and the counting of seed germination by means of image processing becomes possible.
 |
| Fig. 2 Three types of 3D-printed germination array (A), seeds in the array-wells (B), and the coupling of the germination array and the seed planter (C). | |
The seed planters were designed according to the sizes of the seeds and 3D-germination arrays (Fig. 2C and Table 1). For instance, the size of a Sainfoin seed is 5.1 mm, while the dimension of an individual well of this array chip is 6 mm. The entrance hole that connects the seed storage chamber and delivering unit is 5.5 mm in diameter, which can permit only one seed to fall into the delivering blade (Fig. 2C). When the blade is rotated facing the exit hole of the delivering body, the seed can be safely placed into the individual well. There are 10 delivering units on the seed planter. Thus, by simply rotating the crank, 10 seeds can be easily placed into the arrayed wells. The coupling of the germination array and the seed planter can facilitate the placement of single seeds in a high-throughput manner (Fig. 2C). Because the entrance and exit hole within the planter are all designed as round, the filling efficiency of the planter would be affected by the shape of the seed. A round-shape seed is more likely be precisely processed. Three model seeds were processed by the planter. The filling efficiency for Sainfoin, Seasonal cabbage and Liuye amaranth is 98 ± 1.0%, 97 ± 1.8% and 94 ± 2.1%, respectively. Compared with commercial vacuum seed planting, the 3D printed seed planter can achieve delivery of single seeds in an arrayed format without using equipment. This equipment-free, energy/power consumption free approach would favor its in-field applications.
Precisely counting the germinated seeds is one key step in germination testing. Previously, specific devices, such as a vacuum seed counter, have been used to count seed number. In the absence of counting devices, the work may be accomplished manually, which is a time-consuming process. To prevent the breeding of mold or fungus, the filter paper needs to be replaced regularly during the experiment. With previous methods, this made it difficult to count the germinated seeds, especially for the accurate tracking of germinating seeds. In our device, the filter paper could be replaced by simple extraction without moving the seeds. The seed position was fixed throughout the germination experiment cycle, which provided a basis for rapid seed germination statistics through image analysis. As shown in Fig. 3, the image processing software Image Pro Plus (IPP6.0, MediaCybernetics, USA) was applied. Since the color of the sprouts was lighter than that of the seed coat, the number of new sprouts could be counted after the gray images were processed instead of by manual counting. The number of extracted sprouts that were automatically calculated by image processing was close to that of manual counting (Fig. 3). To note, the manual counting of a large number of seeds, such as 400, is time-consuming and labor-intensive. Moreover, the random placement of seeds in a Petri dish increases the difficulty of fast and accurate counting. The combination of array-positioned seeds and computer-based image analysis will improve the efficiency and accuracy of germination evaluation.
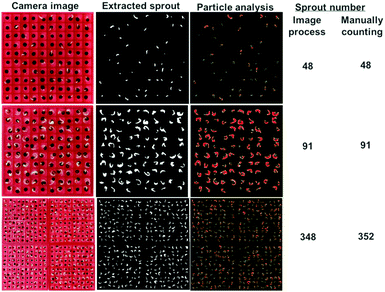 |
| Fig. 3 Computer-assisted image analysis for high-throughput counting of sprouts. | |
3.2 The 3D-germination array improves the efficiency of germination tests
The 3D array-based germination test was performed with three different types of seeds. A standard paper-bed germination test was performed as a reference. As shown in Fig. 4A, the sprouts can be clearly observed from an array of Seasonal cabbage seeds. Because the position of the seed remains the same during the whole test, the growth of the sprout can be tracked well. The same phenomena were observed for Liuye amaranth and Sainfoin seeds (Fig. S1, ESI†). The calculated GPs, from both the 3D-germination array and standard paper-bed assay, were compared in Fig. 4B. The GPs of Sainfoin, Seasonal cabbage and Luiye amaranth calculated from the 3D array were 95 ± 1.0%, 97 ± 1.7% and 82 ± 2.0%, respectively. These GPs of the three types of seeds are only 0.7% (p = 0.64), 1% (p = 0.55) and 5% (p = 0.15) different from those obtained from the paper-bed assay. No statistical difference is shown in the two experiment settings. The results demonstrate that the 3D-printing resin is biocompatible and does not inhibit seed germination. In addition, changing of the filter paper during the entire period of testing avoids frequent movement of the fragile sprouts, ensuring healthy growth of the seedlings. The growing speed of individual seedlings can also be well documented (Fig. 4C). The 3D printed array box and seed planter are compatible with a sterilization process, such as autoclave and ethanol immersion. The tools can be sterilized to avoid contamination.
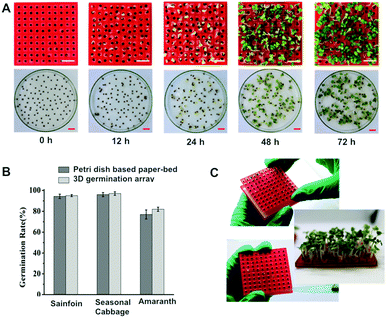 |
| Fig. 4 Comparison of germination tests conducted in a 3D-germination array and a Petri dish-based paper-bed assay. (A) Photograph of Seasonal cabbage seeds sprouting after different periods of incubation. Scale bar denotes 10 mm; (B) germination rate obtained from the 3D-array chip and paper-bed in a petri dish; the germination percentage was given as mean ± SD (standard deviation) of triplicate tests with 100 seeds; (C) ease of solution and filter-paper handling. | |
Compared with the standard paper-bed germination test, the merits of the 3D array-based germination test can be summarized as (Table 2) follows. (1) Single-seed inoculation in an array format avoids spreading of pathogens between seeds. (2) Individual seeds are held in hollow cone-shaped wells, facilitating the easy replacement of the filter paper during the assay. Moreover, the potential damage to the seed or sprout because of repeated manual handling is also eliminated. (3) Because of the regular distribution of seeds in an array format, image process-assisted germination counting is ensured, while the growth trend of an individual seed can be effectively tracked. The 3D-germination array is a low cost, easy-to-use miniaturized platform for high-throughput seed evaluation.
Table 2 Comparison of the 3D germination array and petri dish-based paper bed
|
3D-germination array |
Petri dish-based paper-bed |
Filter paper replacement |
The filter paper in the trays can be replaced by lifting the upper box without disturbing the seeds. |
The seeds need to be transferred to a new filter paper and resituated; thus, the relative positions can easily be changed. |
Pathogen spread |
Individual seeds are separated to avoid spread of contact-mediated pathogens. |
The seeds can easily be in direct contact with one another, increasing the rate of pathogen spreading. |
Sprout counting |
Arrayed seeds facilitate computer-based image analysis to count the sprouts. |
Counting of seeds germinated in a single Petri dish is time-consuming. |
Number of tested seeds |
A large number of seeds can be processed by using a greater number of 3D-germination array units. |
This method is only suitable for processing less than 100 seeds. |
3.3 The effect of different salt concentrations on seed germination was rapidly determined using the 3D-germination array
Most studies suggest that salt stress has a significant inhibitory effect on seed germination. In the present study, NaCl was used as a salt stress factor model, and the effects of different concentrations of NaCl on the germination of Seasonal cabbage seeds were studied. 4 array chips (10 × 10) were put together to analyze 400 seeds to increase the sample volume. As shown in Fig. 5, the germination was inhibited to different degrees after being treated with different concentrations of NaCl. With an increase in the NaCl concentration, the GP and relative GP of the seeds greatly decreased. When the NaCl concentration increased from 0 to 0.2 mol L−1, the GPs decreased from 92.2 ± 2.9% to 66.75 ± 4.8% (Fig. 5B). Further increasing the salt concentration to 0.4 mol L−1, only 8.5 ± 1.0% of seeds can germinate. In addition, the relative germination percentage was calculated by comparing to the germination rate of seeds cultured under normal conditions (without NaCl stress). The relative GP is 83.9 ± 3.0%, 71.8 ± 5.7%, 48.6 ± 3.8% and 9.1 ± 0.9% under NaCl concentrations of 0.1, 0.2, 0.3 and 0.4 mol L−1 (Fig. 5C). Changes in GP with time (within 96 hours) under different NaCl concentrations are shown in Fig. 5D. A germination delay appeared when the NaCl concentration was increased. In the control group (no NaCl), the germination reached a plateau after 12 h of incubation. For increasing NaCl concentrations of 0.1, 0.2, and 0.3 mol L−1, the plateau of the germination curve was delayed to 48 h. Furthermore, because 4 array chips (10 × 10) were used to test 400 seeds, the germination percentage on an individual array chip was compared to evaluate the uniformity of the assay with a large number of seeds. As shown in Table S1 (ESI†), the variation (standard deviation) between individual array chips is in the range of 0.82% to 7.07%. For example, when the seeds were treated with salt solution (0.1 mol L−1) for 24 h, the germination rates of seeds tested on 4 individual chips (10 × 10) are 88%, 93%, 92% and 90%, respectively. The comparable value obtained from each array chip would ensure the accuracy and reproducibility of assays with a large number of seeds. It is clear that the 3D-germination array is a low cost, easy-to-use miniaturized device that evaluates the germination potential of seeds. This equipment-free method could be conducted in the field to quickly evaluate the quality of seeds. Moreover, it provides a high-throughput method of investigating the impact of environment changes on seedlings.
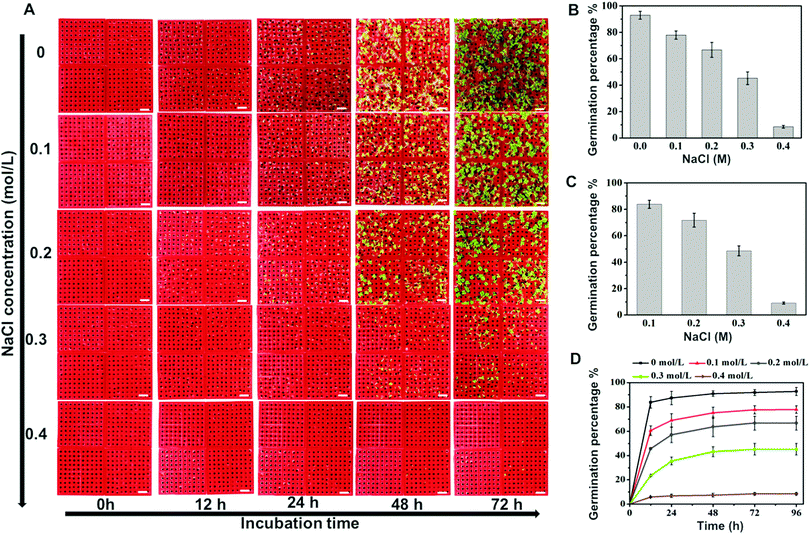 |
| Fig. 5 Fast screening of the effect of various salt concentrations on seed germination in a 3D-germination array. (A) Images of the germination assay on a 3D well-array: the salt stress was established by NaCl concentrations of 0, 0.1, 0.2, 0.3, and 0.4 M; scale bar denotes 10 mm; (B) germination percentage of Seasonal cabbage at different concentrations of salt stress 96 h after seeding; the germination percentage was given as mean ± SD (standard deviation) of triplicate tests with 400 seeds; (C) relative germination percentage of Seasonal cabbage at different concentrations of salt 96 h after seeding; (D) the germination percentage vs. seeding time. | |
4. Conclusions
A germination array and seed planter were designed and fabricated by 3D printing. An inverted hollow cone-shaped germination well was printed as a single-seed container. Simply rotating the crank of the seed planter can easily deliver 10 seeds into the arrayed wells. The combination of the germination array and seed planter can facilitate placement of a single seed in a high-throughput manner. A seed germination test was conducted with the 3D-printed miniaturized device. Compared with standard Petri dish-based paper-bed germination, the 3D-germination array shows the advantages of requiring less manual handling of seeds and counting of sprouts. The combination of the germination array with image processing can be used to study the effects of various external stresses on seed germination. The apparatus is well-suited for in-field studies of seed quality and screening of conditions for seedlings.
Conflicts of interest
There are no conflicts to declare.
Acknowledgements
This work was financially supported by the National Key Scientific Instrument and Equipment Development Projects of China under Contract No. 2013YQ03062909, the National Natural Science Foundation of China (No. 31200700 and 21375108), Fundamental Research Funds for the Central Universities (XDJK2015B020, XDJK2016C152 and XDJK2016A010), and Chongqing Engineering Research Center for Micro-Nano Biomedical Materials and Devices.
References
- W. M. Waterworth, C. M. Bray and C. E. West, J. Exp. Bot., 2015, 66, 3549–3558 CrossRef CAS PubMed.
- L. Rajjou, M. Duval, K. Gallardo, J. Catusse, J. Bally, C. Job and D. Job, Annu. Rev. Plant Biol., 2012, 63, 507–533 CrossRef CAS PubMed.
- R. Angelovici, G. Galili, A. R. Fernie and A. Fait, Trends Plant Sci., 2010, 15, 211–218 CrossRef CAS PubMed.
- H. W. M. Hilhorst and C. M. Karssen, Plant Growth Regul., 1992, 11, 225–238 CrossRef CAS.
- J.-B. Pouvreau, Z. Gaudin, B. Auger, M.-M. Lechat, M. Gauthier, P. Delavault and P. Simier, Plant Methods, 2013, 9, 32 CrossRef CAS PubMed.
- R. W. McEwan, L. G. Arthur-Paratley, L. K. Rieske and M. A. Arthur, Flora, 2010, 205, 475–483 CrossRef.
- K. Tajiri, H. Kishi, Y. Tokimitsu, S. Kondo, T. Ozawa, K. Kinoshita, A. Jin, S. Kadowaki, T. Sugiyama and A. Muraguchi, Cytometry, Part A, 2007, 71A, 961–967 CrossRef CAS PubMed.
- W. C. Lee, S. Rigante, A. P. Pisano and F. A. Kuypers, Lab Chip, 2010, 10, 2952–2958 RSC.
- D. D. Carlo, L. Y. Wu and L. P. Lee, Lab Chip, 2006, 6, 1445–1449 RSC.
- Y.-H. Park, N. Lee, G. Choi and J.-K. Park, Lab Chip, 2017, 17, 3071 RSC.
- S. Waheed, J. M. Cabot, N. P. Macdonald, T. Lewis, R. M. Guijt, B. Paull and M. C. Breadmore, Lab Chip, 2016, 16, 1993 RSC.
- C. M. B. Ho, S. H. Ng, K. H. H. Li and Y.-J. Yoon, Lab Chip, 2015, 15, 3627–3637 RSC.
- Y. He, Q. Gao, W. B. Wu, J. Nie and J. Z. Fu, Micromachines, 2016, 7, 108 CrossRef.
Footnote |
† Electronic supplementary information (ESI) available. See DOI: 10.1039/c7ib00178a |
|
This journal is © The Royal Society of Chemistry 2018 |
Click here to see how this site uses Cookies. View our privacy policy here.