DOI:
10.1039/C7GC02906C
(Communication)
Green Chem., 2018,
20, 141-147
Visible-light-induced regioselective sulfenylation of imidazopyridines with thiols under transition metal-free conditions†
Received
25th September 2017
, Accepted 30th November 2017
First published on 1st December 2017
Abstract
A metal-free visible-light-promoted regioselective C-3 sulfenylation of imidazo[1,2-a]pyridines and indoles using thiols has been developed via C(sp2)–H functionalization. This method provides direct access to a wide range of structurally diverse 3-sulfenylimidazopyridines of biological interest. The operational simplicity, eco-energy source, high atom efficiency, and the use of green solvents under ambient conditions are some of the attractive features of this methodology.
Introduction
The introduction of a sulfur moiety into organic molecules is one of the fundamental processes in organic synthesis. Sulfur-containing compounds are of much importance as they are present in many biologically and medicinally active molecules.1 Among bioactive azaheterocycles, pyridine derivatives play a significant role in medicinal chemistry.2
Over the past few decades, considerable attention has been paid to imidazo[1,2-a]pyridines as these are core units of many natural products3 and have substantial applications in pharmaceutical and biological activities such as antiviral, antibacterial, fungicidal, cytotoxic, and anti-inflammatory activities,4 including GABA inhibitors.5 Imidazo[1,2-a]-pyridine derivatives are also used as commercially available drugs including alpidem, olprinone, minodronic acid (to treat anxiety, heart failure, and osteoporosis), zolimidine (peptic ulcer), zolpidem, necopidem, saripidem, and optically active GSK812397 (HIV infection) (Fig. 1).6 In addition, these are used as charge transporters in materials science.7 Moreover, due to their antiproliferative activity against melanoma cells, functionalized imidazo[1,2-a]pyridines have also been recognized in medicinal chemistry, used in tubulin polymerization, and as protein kinase inhibitors.8
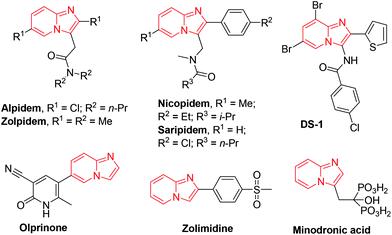 |
| Fig. 1 Examples of imidazo[1,2-a]pyridine-based therapeutics. | |
A combination of the structural unity between imidazo[1,2-a]pyridine and an organosulfur group was successfully used in the design of a new class of inhibitors of human rhinovirus (A),9 with similar effects to enviroxime, and the new anthelminthic B (Fig. 2).10
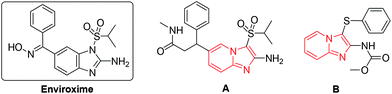 |
| Fig. 2 3-Thioimidazo[1,2-a]pyridines A and B with similar effects to enviroxime. | |
Thus sulfenylation at C3 of imidazo[1,2-a]pyridines is of much significance and has been accomplished using copper,11 iodine derivatives,12 hypervalent iodine reagents,13 strong acidic conditions,14 ionic liquids,15 or silica-supported CeCl3·7H2O/NaI,16 NCS,17 NBS,18 and ArSCl derivatives.19 Recently, Adimurthy et al. described a catalyst-free 3-sulfenylation of imidazopyridine with sulfonothioate as the sulfur source at high temperature.20 These methods involve thiolating agents such as thiols, disulfides, arylsulfonyl chlorides, sulfonyl hydrazides, sodium sulfinates, sulfur powder and sulfonothioate (Scheme 1). Though these strategies were successfully employed for the sulfenylation of imidazo[1,2-a]pyridines, most of them have various disadvantages. Some of the sulfenylating reagents such as sulfonyl hydrazides are not commercially available and are also expensive. In addition, the use of metals and bases limited the further application of these protocols in industry and pharmacy.11a,21 The separation of the metal catalyst from products is of particular importance for the synthesis of pharmaceuticals because of their residual toxicity in the target compound which is a central issue to consider. Moreover, transition-metal-catalyzed reactions also generate environmentally problematic hazardous waste, and hence, should be avoided wherever possible. So, it is highly desirable to develop environmentally benign chemical processes to access pharmacologically potent thiolated imidazoheterocyclic compounds without the use of any metal catalysts. The direct sulfenylation of aromatic C–H bonds, which does not require the presence of reactive functional groups (such as metal moieties or halogens), is an attractive strategy in organic synthesis.22
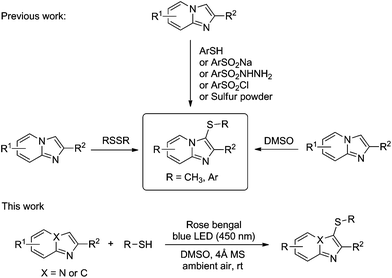 |
| Scheme 1 Different routes for the synthesis of 3-sulfenylimidazopyridine derivatives. | |
Thiols and disulfides are the most frequently employed thiolating reagents for the sulfenylation of imidazopyridines and indoles.23 Due to their ready availability, low cost as well as general functional group tolerance thiols and disulfides have been utilized as attractive starting materials for sulfenylation reactions. The main problem of using disulfide as the thiolating reagent is that disulfides need to be synthesized from thiols via the oxidative coupling reaction, which includes an additional operation step and low atom economics.24 Thus we consider thiol as our choice of reagent.
In the past decade, a novel application of visible-light-mediated photoredox catalysis in synthetic organic chemistry has been extensively exploited because of its environmental compatibility, inexpensiveness, excellent functional group tolerance, high reactivity and versatility in promoting a large number of synthetically important reactions.25 Organic dyes have been used as an effective alternative to transition metal photoredox catalysts.26 Recently, Hajra et al. developed the thiocyanation of imidazoheterocycles through visible light photoredox catalysis.27 Very recently, Guo reported a visible-light promoted protocol for the synthesis of 3-arylthioindoles with thiols as the sulfenylating agent and rose bengal as the photocatalyst.28 However, there is no report on visible-light induced sulfenylation of imidazo[1,2-a]pyridine derivatives with thiols.29 As a part of our continued studies focusing on the synthesis of sulfur-containing compounds,30 herein, we wish to report a novel and mild protocol for the sulfenylation of imidazo[1,2-a]pyridines using thiol in the presence of rose bengal as the photosensitizer under ambient conditions through a visible-light irradiated process (Scheme 1).
Results and discussion
We began our investigation with 2-phenylimidazo[1,2-a]pyridine (1a) and thiophenol (2a) as the model substrate to find suitable reaction conditions, as summarized in Table 1. Initially, the reaction was carried out by employing 0.5 equiv. of 1a with 0.5 equiv. of thiophenol (2a) and eosin Y (5 mol%) as the photocatalyst in CH3CN in open air in the presence of blue LED light. The desired product 3a was obtained in 35% yield after 6 h (Table 1, entry 1). The structure of 3a was characterized by 1H and 13C NMR spectroscopy. A higher yield of 3a was obtained when 1.1 equiv. of 2a was employed (Table 1, entry 2). When the reaction was performed under nitrogen the desired sulfenylation product 3a was obtained in 30% yield (Table 1, entry 3). Other photocatalysts such as rose bengal, rhodamine B, Ru(bpy)3Cl2·6H2O and acridine red were also examined (Table 1, entries 4–7). Among these, rose bengal demonstrated the best catalytic activity to give the desired product 3a in 75% yield (Table 1, entry 4). Among a variety of solvents such as DMF, DMSO, THF, methanol, DCE, toluene, 1,4-dioxane and acetone investigated (Table 1, entries 8–15) the best result (90%) was obtained in DMSO (Table 1, entry 9). An improved yield of 3a was obtained when 4 Å molecular sieves were added (Table 1, entry 16). When green LED (4 W) was used instead of blue LED, a decrease in yield (65%) was observed even after 12 h (Table 1, entry 17). Moreover, no product formation was observed in the absence of visible-light irradiation, and only a trace amount of 3a was obtained without any photocatalysts (Table 1, entries 18 and 19). The loading of the photocatalyst was also optimized and the results are summarized in Table 1 (entry 20).
Table 1 Optimization of the reaction parametersa
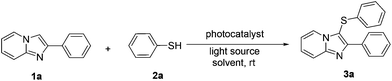
|
Entry |
1a/2a (equiv.) |
Photocatalyst (mol %) |
Light source |
Solvent |
Yieldb (%) |
Reaction conditions: 1a (0.5 mmol), 2a (0.6 mmol), photocatalyst (5.0 mol%), solvent (2.0 mL), 4 Å molecular sieves (80 mg), r.t., air, blue LED (450 nm) for 6 h.
Isolated yield.
Nitrogen atmosphere.
Rose bengal (1.0 mol%) was used.
Rose bengal (3.0 mol%) was added.
Rose bengal (10 mol%) was used.
|
1 |
0.5/0.5 |
Eosin Y |
Blue LED |
CH3CN |
35 |
2 |
0.5/0.6 |
Eosin Y |
Blue LED |
CH3CN |
55 |
3 |
0.5/0.6 |
Eosin Y |
Blue LED |
CH3CN |
30c |
4 |
0.5/0.6 |
Rose bengal |
Blue LED |
CH3CN |
75 |
5 |
0.5/0.6 |
Rhodamine B |
Blue LED |
CH3CN |
45 |
6 |
0.5/0.6 |
Ru(bpy)3Cl2·6H2O |
Blue LED |
CH3CN |
20 |
7 |
0.5/0.6 |
Acridine red |
Blue LED |
CH3CN |
55 |
8 |
0.5/0.6 |
Rose bengal |
Blue LED |
DMF |
60 |
9 |
0.5/0.6 |
Rose bengal |
Blue LED |
DMSO |
90 |
10 |
0.5/0.6 |
Rose bengal |
Blue LED |
THF |
35 |
11 |
0.5/0.6 |
Rose bengal |
Blue LED |
Methanol |
18 |
12 |
0.5/0.6 |
Rose bengal |
Blue LED |
Chloroform |
15 |
13 |
0.5/0.6 |
Rose bengal |
Blue LED |
DCE |
45 |
14 |
0.5/0.6 |
Rose bengal |
Blue LED |
Toluene |
25 |
15 |
0.5/0.6 |
Rose bengal |
Blue LED |
1,4-Dioxane |
20 |
16
|
0.5/0.6
|
Rose bengal
|
Blue LED
|
DMSO
|
95
|
17 |
0.5/0.6 |
Rose bengal |
Green LED |
DMSO |
65 |
18 |
0.5/0.6 |
Rose bengal |
— |
DMSO |
— |
19 |
0.5/0.6 |
— |
Blue LED |
DMSO |
Trace |
20 |
0.5/0.6 |
Rose bengal |
Blue LED |
DMSO |
35d, 65e, 85f |
A reaction between unsubstituted imidazo[1,2-a]pyridine 4 with thiophenol under optimized reaction conditions (Table 1, entry 16) produced C-3 sulfenylated product 4a in 85% yield (Scheme 2). When C3 substituted 3-methylimidazo[1,2-a]pyridine 5 was subjected to the reaction under the same conditions no C2 sulfenylated product 5a was observed. These experiments (Scheme 2) indicate that when the C-3 position of imidazo[1,2-a]pyridine is blocked by any group, no reaction takes place to yield the thiolated product. The reported method proceeds with only C-3 unsubstituted imidazopyridine core units to yield 3-sulfenylated imidazo[1,2-a]pyridines in a selective manner.
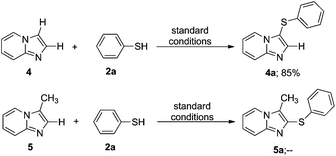 |
| Scheme 2 Selective sulfenylation. | |
To explore the generality of this direct sulfenylation reaction, different substituted imidazo[1,2-a]pyridines 1 were reacted with various thiols 2 under optimized conditions (Table 1, entry 16). The results are listed in Table 2. A variety of imidazo[1,2-a]pyridines underwent the direct sulfenylation with thiols (2) smoothly to afford the desired products 3 in good to excellent yields. The imidazopyridine moiety bearing electron-donating groups (–Me, –Et and –OMe) of the phenyl ring at the C-2 position provided the C-3 sulfenylated products in excellent yields (3b–3d). Similarly, the presence of electron-withdrawing groups (–Cl, –Br, and –CN) of the phenyl ring produced the corresponding products with good yields (3e–3g). As evident from the results 3a–3g, the electronic effects associated with electron-donating/-withdrawing substituents on the phenyl ring at the C-2 position of imidazopyridines do not affect the efficiency of the reaction. The reaction between 2 with various substituted imidazo[1,2-a]pyridines (substituents on the pyridine ring of imidazopyridine, such as –Br, –Me, and –OMe) under the optimized conditions provided the corresponding C3-sulfenylated products in moderate to excellent yields (3h–3k). Interestingly, important heterocycles such as 3-(phenylthio)-2-(thiophene-2-yl)imidazo[1,2-a]-pyridine gave the product in excellent yields under the present conditions (3l). Alkyl substituents imidazopyridine such as 2-Me and 2-tert-butyl also worked well in this reaction to give the expected products without any difficulties (3m–3o). Interestingly, when indole was used as a substrate instead of imidazopyridine, under the standard conditions C3-sulfenylated indole was obtained in high yields (3q–3t).
Table 2 Scope of imidazo[1,2-a]pyridines 1 and thiols 2
a
Reaction conditions: 1 (0.5 mmol), 2 (0.6 mmol), rose bengal (5.0 mol%), DMSO (2.0 mL), 4 Å molecular sieves (80 mg), r.t., air, blue LED (450 nm) for 6–7 h. Isolated yield.
|
|
Subsequently, the scope of thiols (2) was also investigated with 1 under the optimized reaction conditions. Thiols containing an electron-rich group (–OMe, –Me) on the para-positions of the benzene rings reacted with 1 to give the corresponding products (3u, 3v and 3ab) in 85–88% yields. Meanwhile, the reactions of 1 with thiols attached to relatively electron-deficient groups such as –Cl and –Br on the para-positions of the phenyl ring afforded the corresponding products (3w and 3x) in excellent yields. The reactions of naphthyl thiol with 1a also gave the desired product 3y in good yield. Besides aryl thiols this protocol was also successfully applied to alkyl thiols such as methanethiol and butanethiol which gave good to excellent conversions to the desired products (3z, and 3aa).
The gram-scale reaction was also performed under the optimized reaction conditions as shown in Scheme 3, which demonstrates the practical applicability of the new protocol. The reaction between 2-phenylimidazo[1,2-a]pyridine (1a) and thiophenol (2a) in the presence of rose bengal (5 mol%), 4 Å molecular sieves in DMSO irradiated by blue LED (450 nm) for 9 h under an open atmosphere afforded the desired product 3a in 87% yield.
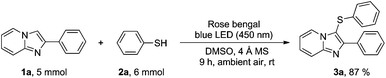 |
| Scheme 3 Gram-scale reaction. | |
To understand this transformation, some selective and control experiments were conducted and the results are listed in Scheme 4. The sulfenylation reaction did not proceed when 2.5 equiv. of 2,2,6,6-tetramethyl-1-piperidinyloxy (TEMPO), a radical scavenger, was present in the reaction system (Scheme 4, entry 1). This indicates that a radical pathway might be involved in this transformation. We also carried out the reaction in the dark (Scheme 4, entry 2) and found that the reaction did not proceed at all, which also suggests the radical mechanism. Moreover, the reaction was performed in the presence of DABCO with diphenyl disulfide under the standard conditions (Scheme 4, entry 3). The reaction delivered product 3a with equal ease, which indicates that singlet oxygen is not involved in the reaction.26m In addition, when 2-phenylimidazo[1,2-a]pyridine (1a) reacted with 1,2-diphenyldisulfane under standard conditions, none of the product 3a was detected, which showed that the disulfide might not be involved in this transformation.
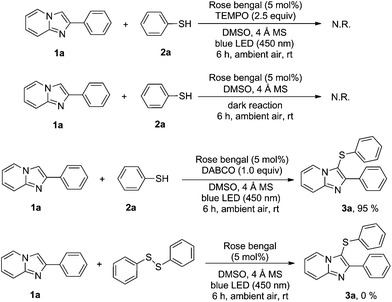 |
| Scheme 4 Control experiments. | |
On the basis of previous reports25p,26i,o,p,q,27,28 and the control experiments, we proposed a plausible reaction pathway for the photocatalytic sulfenylation of imidazo[1,2-a]pyridine as shown in Scheme 5. Initially, rose bengal (RB) was excited under the presence of blue LED light to produce RB*. Then, a single electron transfer (SET) from thiol 2a to RB* afforded the radical cation A and RB˙−. RB˙− oxidized by air generated the ground state rose bengal and O2˙−. The deprotonation of radical cation A by O2˙− leads to the formation of stabilized thiyl radical B. The resulting thiyl radical (B) reacts with 1a to produce the radical intermediate C. Finally, C is oxidized to intermediate D along with the generation of HOO−. The deprotonation (aromatization process) of D afforded the desired product 3a along with the release of H2O2. To complete the photoredox cycle aerobic oxygen probably plays a crucial role to oxidize RB radical anions to the ground state.
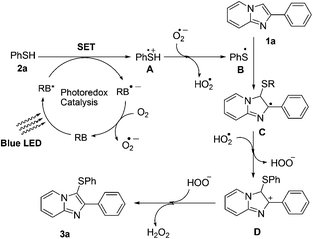 |
| Scheme 5 Plausible reaction mechanism. | |
Conclusions
In summary, we have developed an efficient and facile strategy for the regioselective C-3 sulfenylation of imidazo[1,2-a]pyridine derivatives and indoles with thiols at room temperature through a visible-light-promoted process in the presence of rose bengal. A series of biologically important C-3 thiolated imidazo[1,2-a]pyridines was obtained conveniently and efficiently in good to excellent yields using readily available materials. The reported method is endowed with several important features including operational simplicity, eco-energy source, high atom efficiency, and green solvent under ambient conditions. Most importantly, the present methodology is scalable. Notably, this methodology in contrast to the previous reports is free from using transition metal catalysts and strong oxidants. To the best of our knowledge, there is no report on the C-3 sulfenylation of imidazopyridines using thiol under a photo-catalysed process. Because of the importance of thiolated imidazopyridines for biological and pharmaceutical sciences this procedure will find wider applications in industry as well as in academia.
Conflicts of interest
There are no conflicts to declare.
Acknowledgements
The authors are thankful to Prof. Brindaban C. Ranu, Indian Association for the Cultivation of Science, Kolkata, for valuable discussion and suggestions. MHRD, Govt. of India is acknowledged for the doctorate fellowship (MHRD GATE fellowship) received by R. R. The authors also acknowledge CBMR, Lucknow and SAIC, Tezpur University for spectral analysis.
Notes and references
-
(a) J. Dumas, D. Brittelli, J. Chen, B. Dixon, H. Hatoum-Mokdad, G. Konig, R. Sibley, J. Witowsky and S. Wong, Bioorg. Med. Chem. Lett., 1999, 9, 2531 CrossRef CAS PubMed;
(b) J. Koci, V. Klimesova, K. Waisser, J. Kaustova, H.-M. Dahse and U. Mollmann, Bioorg. Med. Chem. Lett., 2002, 12, 3275 CrossRef CAS PubMed;
(c) R. Paramashivappa, P. Phani Kumar, P. V. Subba Rao and A. Srinivasa Rao, Bioorg. Med. Chem. Lett., 2003, 13, 657 CrossRef CAS PubMed;
(d) W. Huang and G.-F. Yang, Bioorg. Med. Chem., 2006, 14, 8280 CrossRef CAS PubMed;
(e) L. Zhang, J. Fan, K. Vu, K. Hong, J.-Y. Le Brazidec, J. Shi, M. Biamonte, D. J. Busch, R. E. Lough, R. Grecko, Y. Ran, J. L. Sensintaffar, A. Kamal, K. Lundgren, F. J. Burrows, R. Mansfield, G. A. Timony, E. H. Ulm, S. R. Kasibhatla and M. F. Boehm, J. Med. Chem., 2006, 49, 5352 CrossRef CAS PubMed;
(f) J. Shanmugapriya, K. Rajaguru, S. Muthusubramanian and N. Bhuvanesh, Eur. J. Org. Chem., 2016, 1963 CrossRef CAS.
-
(a)
J. A. Joule and K. Mills, in Heterocyclic Chemistry, Blackwell, Oxford, U.K., 4th edn, 2000 Search PubMed;
(b) J. P. Michael, Nat. Prod. Rep., 2005, 22, 627 RSC.
-
(a)
F. Couty and G. Evano, in Comprehensive Heterocyclic Chemistry III, Elsevier, Oxford, U.K., 2008, vol. 11, p. 409 Search PubMed;
(b) J. Liu and Q. Chen, Huaxue Jinzhan, 2010, 22, 631 CAS;
(c) C. Enguehard-Gueiffier and A. Gueiffier, Mini-Rev. Med. Chem., 2007, 7, 888 CrossRef CAS PubMed;
(d) E. F. DiMauro and J. M. Kennedy, J. Org. Chem., 2007, 72, 1013 CrossRef CAS PubMed.
-
(a) J. J. Kaminski and A. M. Doweyko, J. Med. Chem., 1997, 40, 427 CrossRef CAS PubMed;
(b) K. C. Rupert, J. R. Henry, J. H. Dodd, S. A. Wadsworth, D. E. Cavender, G. C. Olini, B. Fahmy and J. J. Siekierka, Bioorg. Med. Chem. Lett., 2003, 13, 347 CrossRef CAS PubMed;
(c) S. K. Kotovskaya, Z. M. Baskakova, V. N. Charushin, O. N. Chupakhin, E. F. Belanov, N. I. Bormotov, S. M. Balakhnin and O. A. Serova, Pharm. Chem. J., 2005, 39, 574 CrossRef CAS.
-
(a) K. F. Byth, J. D. Culshaw, S. Green, S. E. Oakes and A. P. Thomas, Bioorg. Med. Chem. Lett., 2004, 14, 2245 CrossRef CAS PubMed;
(b) C. Hamdouchi, B. Zhong, J. Mendoza, E. Collins, C. Jaramillo, J. E. De Diego, D. Robertson, C. D. Spencer, B. D. Anderson, S. A. Watkins, F. Zhang and H. B. Brooks, Bioorg. Med. Chem. Lett., 2005, 15, 1943 CrossRef CAS PubMed.
-
(a) S. Z. Langer, S. Arbilla, J. Benavides and B. Scatton, Adv. Biochem. Psychopharmacol., 1990, 46, 61 CAS;
(b) T. Okubo, R. Yoshikawa, S. Chaki, S. Okuyama and A. Nakazato, Bioorg. Med. Chem., 2004, 12, 423 CrossRef CAS PubMed;
(c) K. Mizushige, T. Ueda, K. Yukiiri and H. Suzuki, Cardiovasc. Drug Rev., 2002, 20, 163 CrossRef CAS PubMed;
(d) H. Mori, M. Tanaka, R. Kayasuga, T. Masuda, Y. Ochi, H. Yamada, K. Kishikawa, M. Ito and T. Nakamura, Bone, 2008, 43, 840 CrossRef CAS PubMed;
(e) L. Almirante, L. Polo, A. Mugnaini, E. Provinciali, P. Rugarli, A. Biancotti, A. Gamba and W. Murmann, J. Med. Chem., 1965, 8, 305 CrossRef CAS PubMed;
(f) R. J. Boerner and H. J. Möller, Psychopharmakotherapy, 1997, 4, 145 Search PubMed;
(g)
K. Gudmundsson and S. D. Boggs, PCT Int. Appl. WO2006026703, 2006 Search PubMed.
-
(a) J. Wan, C.-J. Zheng, M. K. Fung, X.-K. Liu, C.-S. Lee and X.-H. Zhang, J. Mater. Chem., 2012, 22, 4502 RSC;
(b) A. J. Stasyuk, M. Banasiewicz, M. K. Cyran and D. T. Gryko, J. Org. Chem., 2012, 77, 5552 CrossRef CAS PubMed;
(c) H. Shono, T. Ohkawa, H. Tomoda, T. Mutai and K. Araki, ACS Appl. Mater. Interfaces, 2011, 3, 654 CrossRef CAS PubMed.
-
(a) R. Garamvölgyi, J. Dobos, A. Sipos, S. Boros, E. Illyés, F. Baska, L. Kékesi, I. Szabadkai, C. Szántai-Kis, G. Kéri and L. Őrfi, Eur. J. Med. Chem., 2016, 108, 623 CrossRef PubMed;
(b) M. Lawson, J. Rodrigo, B. Baratte, T. Robert, C. Delehouze, O. Lozach, S. Ruchaud, S. Bach, J. D. Brion, M. Alami and A. Hamze, Eur. J. Med. Chem., 2016, 123, 105 CrossRef CAS PubMed.
- C. Hamdouchi, J. Blas, M. Prado, J. Gruber, B. A. Heinz and L. Vance, J. Med. Chem., 1999, 42, 50 CrossRef CAS PubMed.
- R. J. Bochis, L. E. Olen, M. H. Fisher and R. A. Reamer, J. Med. Chem., 1981, 24, 1483 CrossRef CAS PubMed.
-
(a) D. C. Mohan, S. N. Rao, C. Ravi and S. Adimurthy, Asian J. Org. Chem., 2014, 3, 609 CrossRef CAS;
(b) W. Liu, S. Wang, Y. Jiang, P. He, Q. Zhang and H. Cao, Asian J. Org. Chem., 2015, 4, 312 CrossRef CAS;
(c) Z. Li, J. Hong and X. Zhou, Tetrahedron, 2011, 67, 3690 CrossRef CAS;
(d) C. Hamdouchi, J. de Blas and J. Ezquerra, Tetrahedron, 1999, 55, 541 CrossRef CAS;
(e) H. Cao, L. Chen, J. Liu, H. Cai, H. Deng, G. Chen, C. Yan and Y. Chen, RSC Adv., 2015, 5, 22356 RSC;
(f) C. Ravi, D. Chandra Mohan and S. Adimurthy, Org. Biomol. Chem., 2016, 14, 2282 RSC;
(g) Z. Zheng, D. Qi and L. Shi, Catal. Commun., 2015, 66, 83 CrossRef CAS;
(h) J. Li, C. Li, S. Yang, Y. An, W. Wu and H. Jiang, J. Org. Chem., 2016, 81, 7771 CrossRef CAS PubMed.
-
(a) M.-A. Hiebel and S. Berteina-Raboin, Green Chem., 2015, 17, 937 RSC;
(b) A. K. Bagdi, S. Mitra, M. Ghosh and A. Hajra, Org. Biomol. Chem., 2015, 13, 3314 RSC;
(c) X. Huang, S. Wang, B. Li, X. Wang, Z. Ge and R. Li, RSC Adv., 2015, 5, 22654 RSC;
(d) Y. Ding, W. Wu, W. Zhao, Y. Li, P. Xie, Y. Huang, Y. Liu and A. Zhou, Org. Biomol. Chem., 2016, 14, 1428 RSC;
(e) D. Wang, S. Guo, R. Zhang, S. Lin and Z. Yan, RSC Adv., 2016, 6, 54377 RSC;
(f) X.-M. Ji, S.-J. Zhou, F. Chen, X.-G. Zhang and R.-Y. Tang, Synthesis, 2015, 659 CAS;
(g) K. Yan, D. Yang, P. Sun, W. Wei, Y. Liu, G. Li, S. Lu and H. Wang, Tetrahedron Lett., 2015, 56, 4792 CrossRef CAS;
(h) W. Zhu, Y. Ding, Z. Bian, P. Xie, B. Xu, Q. Tang, W. Wu and A. Zhou, Adv. Synth. Catal., 2016, 358, 2215 Search PubMed.
- C. Hamdouchi, C. Sanchez and J. Ezquerra, Synthesis, 1998, 867 CrossRef CAS.
- S. M. Patil, S. Kulkarni, M. Mascarenhas, R. Sharma, S. M. Roopan and A. Roychowdhury, Tetrahedron, 2013, 69, 8255 CrossRef CAS.
- Z. Gao, X. Zhu and R. Zhang, RSC Adv., 2014, 4, 19891 RSC.
- W. Ge, X. Zhu and Y. Wei, Eur. J. Org. Chem., 2013, 6015 CrossRef CAS.
- C. Ravi, D. Chandra Mohan and S. Adimurthy, Org. Lett., 2014, 16, 2978 CrossRef CAS PubMed.
- R. R. Maddi, P. K. Shirsat, S. Kumar and H. M. Meshram, ChemistrySelect, 2017, 2, 1544 CrossRef CAS.
- R. J. Bochis, L. E. Olen, M. H. Fisher, R. A. Reamer, G. Wilks, J. E. Taylor and G. Olson, J. Med. Chem., 1981, 24, 1483 CrossRef CAS PubMed.
- C. Ravi, A. Joshi and S. Adimurthy, Eur. J. Org. Chem., 2017, 3646 CrossRef CAS.
-
(a) G. Wenlei, Z. Xun and W. Yunyang, Eur. J. Org. Chem., 2013, 6015 Search PubMed;
(b) G. Zhaochang, Z. Xun and Z. Ronghua, RSC Adv., 2014, 4, 19891 RSC.
-
(a) L. H. Zou, J. Reball, J. Mottweiler and C. Bolm, Chem. Commun., 2012, 48, 11307 RSC;
(b) S. R. Neufeldt and M. S. Sanford, Acc. Chem. Res., 2012, 45, 936 CrossRef CAS PubMed;
(c) C. Zhang, C. Tang and N. Jiao, Chem. Soc. Rev., 2012, 41, 3464 RSC;
(d) C. Shen, P. Zhang, Q. Sun, S. Bai, T. S. Andy Hor and X. Liu, Chem. Soc. Rev., 2015, 44, 291 RSC.
-
(a) Y. Maeda, M. Koyabu, T. Nishimura and S. Uemura, J. Org. Chem., 2004, 69, 7688 CrossRef CAS PubMed;
(b) K. M. Schlosser, A. P. Krasutsky, H. W. Hamilton, J. E. Reed and K. Sexton, Org. Lett., 2004, 6, 819 CrossRef CAS PubMed;
(c) J. A. Campbell, C. A. Broka, L. Gong, K. A. M. Walker and J.-H. Wang, Tetrahedron Lett., 2004, 45, 4073 CrossRef CAS;
(d) J. S. Yadav, B. V. S. Reddy and Y. J. Reddy, Tetrahedron Lett., 2007, 48, 7034 CrossRef CAS;
(e) Y. Liu, Y. Zhang, C. Hu, J.-P. Wana and C. Wen, RSC Adv., 2014, 4, 35528 RSC;
(f) S. Yi, M. Li, W. Mo, X. Hu, B. Hu, N. Sun, L. Jin and Z. Shen, Tetrahedron Lett., 2016, 57, 1912 CrossRef CAS;
(g) T. Gensch, F. J. R. Klauck and F. Glorius, Angew. Chem., Int. Ed., 2016, 55, 11287 CrossRef CAS PubMed;
(h) P. Wang, S. Tang, P. Huang and A. Lei, Angew. Chem., Int. Ed., 2017, 56, 3009 CrossRef CAS PubMed;
(i) S. Song, Y. Zhang, A. Yeerlan, B. Zhu, J. Liu and N. Jiao, Angew. Chem., Int. Ed., 2017, 56, 2487 CrossRef CAS PubMed;
(j) F. Xiao, J. Tian, Q. Xing, H. Huang, G.-J. Deng and Y. Liu, ChemistrySelect, 2017, 2, 428 CrossRef CAS;
(k) Z.-H. Yang, Y.-L. An, Y. Chen, Z.-Y. Shao and S.-Y. Zhao, Adv. Synth. Catal., 2016, 358, 3869 CrossRef CAS;
(l) X.-L. Fang, R.-Y. Tang, P. Zhong and J.-H. Li, Synthesis, 2009, 4183 CAS;
(m) Z. Li, J. Hong and X. Zhou, Tetrahedron, 2011, 67, 3690 CrossRef CAS;
(n) G. La Regina, V. Gatti, V. Famiglini, F. Piscitelli and R. Silvestri, ACS Comb. Sci., 2012, 14, 258 CrossRef CAS PubMed;
(o) W. Ge and Y. Wei, Green Chem., 2012, 14, 2066 RSC;
(p) P. Sang, Z. Chen, J. Zoua and Y. Zhang, Green Chem., 2013, 15, 2096 RSC;
(q) Ch. D. Prasad, S. Kumar, M. Sattar, A. Adhikary and S. Kumar, Org. Biomol. Chem., 2013, 11, 8036 RSC;
(r) J. Sun, D. Z. Negrerie and Y. Du, Adv. Synth. Catal., 2016, 358, 2035 CrossRef CAS.
-
(a) H. Wang, G. Huang, Y. Sun and Y. Liu, J. Chem. Res., 2014, 96 CrossRef;
(b) Y. Liu, H. Wang, C. Wang, J.-P. Wan and C. Wen, RSC Adv., 2013, 3, 21369 RSC.
-
(a) J.-C. Chen, X.-Q. Hu, L.-Q. Liu and W.-J. Xiao, Chem. Soc. Rev., 2016, 45, 2044 RSC;
(b) C. K. Prier, D. A. Rankic and D. W. C. MacMillan, Chem. Rev., 2013, 113, 5322 CrossRef CAS PubMed;
(c) J. M. R. Narayanam and C. R. J. Stephenson, Chem. Soc. Rev., 2011, 40, 102 RSC;
(d) T. P. Yoon, M. A. Ischay and J. Du, Nat. Chem., 2010, 2, 527 CrossRef CAS PubMed;
(e) M. N. Hopkinson, B. Sahoo, J.-L. Li and F. Glorius, Chem. – Eur. J., 2014, 20, 3874 CrossRef CAS PubMed;
(f) J. W. Tucker and C. R. J. Stephenson, J. Org. Chem., 2012, 77, 1617 CrossRef CAS PubMed;
(g) J. Xuan and W.-J. Xiao, Angew. Chem., Int. Ed., 2012, 51, 6828 CrossRef CAS PubMed;
(h) S. Maity and N. Zheng, Synlett, 2012, 1851 CAS;
(i) Y.-M. Xi, H. Yi and A.-W. Lei, Org. Biomol. Chem., 2013, 11, 2387 RSC;
(j) T. Koike and M. Akita, Top. Catal., 2014, 57, 967 CrossRef CAS;
(k) D. M. Schultz and T. P. Yoon, Science, 2014, 343, 1239176 CrossRef PubMed;
(l) T. Koike and M. Akita, Inorg. Chem. Front., 2014, 1, 562 RSC;
(m) E. Meggers, Chem. Commun., 2015, 51, 3290 RSC;
(n) M. A. Cismesia and T. P. Yoon, Chem. Sci., 2015, 6, 5426 RSC;
(o) D. Ravelli, S. Protti and M. Fagnoni, Chem. Rev., 2016, 116, 9850 CrossRef CAS PubMed;
(p) K. Ohkubo, K. Mizushima, R. Iwataa and S. Fukuzumi, Chem. Sci., 2011, 2, 715 RSC.
-
(a) M. A. Miranda and H. Garcia, Chem. Rev., 1994, 1063 CrossRef CAS;
(b) M. Fagnoni, D. Dondi, D. Ravelli and A. Albini, Chem. Rev., 2007, 107, 2725 CrossRef CAS PubMed;
(c) S. Fukuzumi and K. Ohkubo, Chem. Sci., 2013, 4, 561 RSC;
(d) M. Neumann, S. Füldner, B. König and K. Zeitler, Angew. Chem., Int. Ed., 2011, 50, 951 CrossRef CAS PubMed;
(e) J. Liu, S. Wen, Y. Hou, F. Zuo, G. J. O. Beran and P. Feng, Angew. Chem., Int. Ed., 2013, 52, 3241 CrossRef CAS PubMed;
(f) M. Majek and A. J. Wangelin, Chem. Commun., 2013, 49, 5507 RSC;
(g) D. P. Hari and B. König, Chem. Commun., 2014, 50, 6688 RSC;
(h) M. Filace, F. Majek and A. J. Wangelin, Beilstein J. Org. Chem., 2014, 10, 981 CrossRef PubMed;
(i) D. Kundu, S. Ahammed and B. C. Ranu, Org. Lett., 2014, 16, 1814 CrossRef CAS PubMed;
(j) D. P. Hari, P. Schroll and B. Konig, J. Am. Chem. Soc., 2012, 134, 2958 CrossRef CAS PubMed;
(k) D.-T. Yang, Q.-Y. Meng, J.-J. Zhong, M. Xiang, Q. Liu and L.-Z. Wu, Eur. J. Org. Chem., 2013, 7528 CrossRef CAS;
(l) Q.-Y. Meng, J.-J. Zhong, Q. Liu, X.-W. Gao, H.-H. Zhang, T. Lei, Z.-J. Li, K. Feng, B. Chen, C.-H. Tung and L.-Z. Wu, J. Am. Chem. Soc., 2013, 135, 19052 CrossRef CAS PubMed;
(m) T. Keshari, V. K. Yadav, V. P. Srivastava and L. D. S. Yadav, Green Chem., 2014, 16, 3986 RSC;
(n) L. Ye, J. Chen, P. Mao, X. Zhang and M. Yan, Tetrahedron Lett., 2017, 58, 2743 CrossRef CAS;
(o) H. Cui, W. Wei, D. Yang, Y. Zhang, H. Zhao, L. Wang and H. Wang, Green Chem., 2017, 19, 3520 RSC;
(p) S. S. Zalesskiy, N. S. Shlapakov and V. P. Ananikov, Chem. Sci., 2016, 7, 6740 RSC;
(q) Q. Shi, P. Li, X. Zhua and L. Wang, Green Chem., 2016, 18, 4916 RSC.
- S. Mitra, M. Ghosh, S. Mishra and A. Hajra, J. Org. Chem., 2015, 80, 8275 CrossRef CAS PubMed.
- W. Guo, W. Tan, M. Zhao, K. Tao, L.-Y. Zheng, Y. Wu, D. Chen and X.-L. Fan, RSC Adv., 2017, 7, 37739 RSC.
- During the submission process of our manuscript Yang and co-workers report a related work. However, our work has several distinctions compared to this in terms of using commercially available thiolating agent (thiols vs. sulfinic acids), oxidizing agent (nil vs. TBHP), and solvent (DMSO vs. DCE), photosensitizer (rose bengal vs. eosin B) and substrate scope (indole and imidazopyridine vs. imidazopyridine), see: P. Sun, D. Yang, W. Wei, M. Jiang, Z. Wang, L. Zhang, H. Zhang, Z. Zhang, Y. Wang and H. Wang, Green Chem., 2017, 19, 4785 RSC.
-
(a) R. Rahaman, N. Devi and P. Barman, Tetrahedron Lett., 2015, 56, 4224 CrossRef CAS;
(b) R. Rahaman, N. Devi, J. R. Bhagawati and P. Barman, RSC Adv., 2016, 6, 18929 RSC;
(c) N. Devi, R. Rahaman, K. Sarma and P. Barman, Eur. J. Org. Chem., 2016, 384 CrossRef CAS;
(d) R. Rahaman, N. Devi, K. Sarma and P. Barman, RSC Adv., 2016, 6, 10873 RSC;
(e) R. Rahaman and P. Barman, Synlett, 2017, 684 CAS;
(f) N. Devi, R. Rahaman, K. Sarma, T. Khan and P. Barman, Eur. J. Org. Chem., 2017, 1520 CrossRef CAS;
(g) R. Rahaman and P. Barman, Eur. J. Org. Chem., 2017, 6327 CrossRef CAS.
Footnote |
† Electronic supplementary information (ESI) available: General information, experimental procedures, characterization data and copies of 1H NMR and 13C NMR spectra of synthesized compounds. See DOI: 10.1039/c7gc02906c |
|
This journal is © The Royal Society of Chemistry 2018 |