DOI:
10.1039/C7FO00662D
(Paper)
Food Funct., 2018,
9, 552-560
Fucoidan inhibits amyloid-β-induced toxicity in transgenic Caenorhabditis elegans by reducing the accumulation of amyloid-β and decreasing the production of reactive oxygen species
Received
4th May 2017
, Accepted 30th November 2017
First published on 20th December 2017
Abstract
Alzheimer's disease (AD) is the most common age-related neurodegenerative disorder. As the aging population is increasing, AD is becoming one of the leading causes of disability and death among the elderly. However, currently there is no cure for this disease. Fucoidan is a complex sulfated polysaccharide mainly found in brown seaweed. Recent studies have shown that fucoidan is neuroprotective and may have potential to be used for treating and/or preventing neurodegenerative diseases such as AD. In this study, we investigated the effects and possible mechanisms of fucoidan on Abeta induced toxicity in a transgenic Caenorhabditis elegans (C. elegans) AD model. The results showed that the supplementation of fucoidan alleviated the paralyzed phenotype induced by Abeta. The number of Abeta deposits in the AD animals was reduced by fucoidan treatment. Further analysis of the levels of Abeta showed that fucoidan significantly decreased the accumulation of Abeta in transgenic AD C. elegans. It was found that fucoidan treatment elevated the activity of proteosomes; therefore, fucoidan might decrease Abeta accumulation by promoting proteolysis. In addition, fucoidan treatment reduced the production of reactive oxygen species (ROS) stimulated by Abeta induction. These results suggested that fucoidan might exert its protective effects against Abeta-induced toxicity in transgenic AD C. elegans by reducing the accumulation of toxic Abeta and decreasing Abeta-induced production of ROS, thus ameliorating the progression of the AD phenotype.
Introduction
Alzheimer's disease (AD) is the most common age-related neurodegenerative disorder. Patients suffer brain tissue damage and recognition dysfunction, including depression and memory impairment.1 As the aging population increases, the prevalence of AD has increased remarkably worldwide and AD has become one of the leading causes of disability and death among the elderly.2,3 Although different therapeutic approaches have been developed for AD, currently, there is no cure for the disease.4
The major pathological characteristics of AD brain are the presence of senile plaques that are mainly composed of the amyloid-β (Abeta) peptide, and neurofibrillary tangles (NFT), which primarily contain self-aggregated hyperphosphorylated tau protein.5,6 According to the Abeta hypothesis, the imbalance between Abeta production and clearance in the central nervous system (CNS) plays a key role in the neurodegeneration of AD patients.7–9 Increased production and/or decreased clearance of Abeta peptides lead to the accumulation of Abeta, which stimulates diverse cell signaling pathways, causing the impairment of neuronal synapse, oxidative stress and inflammation, and eventually resulting in synaptic degeneration, neuronal loss and decline in cognitive function.7–12 Thus, Abeta has been considered as one of the targets for the prevention and treatment of AD.7,11,12
Fucoidan is a complex sulfated polysaccharide mainly found in brown seaweed that is distributed widely in East Asia and consumed as a marine vegetable. It has numerous biological activities including anti-inflammatory, anti-coagulative, anti-cancer and antioxidative effects.13–18 Recent studies have also shown that fucoidan has neuroprotective properties. It was found that fucoidan treatment inhibited oxidative stress-induced apoptosis in PC12 cells19 and prevented N-methyl-D-aspartate (NMDA)-induced neuronal cell death in organotypic hippocampal slice culture.20 Moreover, fucoidan was shown to decrease neuronal death resulted from exposure of Abeta in rat basal forebrain neuronal cultures by blocking Abeta-induced reactive oxygen species (ROS) generation and inhibiting Abeta-stimulated caspase activation.21 These studies suggest that fucoidan is neuroprotective and it may have a potential to be used for treating and/or preventing neurodegenerative diseases such as AD.
In the present study, we evaluated the protective effects of fucoidan in an AD transgenic Caenorhabditis elegans (C. elegans) model in which the Abeta42 peptide is induced in the muscle cells of the worm upon a temperature shift, resulting in the deposition of Abeta that leads to a paralyzed phenotype of the worm that can be easily scored.22 It was found that fucoidan effectively protected AD transgenic C. elegans from Abeta-induced toxicity. Fucoidan treatment significantly ameliorated the development of paralyzed phenotype induced by Abeta. The number of Abeta deposits in the AD animals was reduced by fucoidan treatment. Further analysis of the levels of the Abeta peptide showed that fucoidan significantly decreased the accumulation of Abeta in AD transgenic C. elegans. It was found that fucoidan treatment elevated the activity of proteosomes; therefore, fucoidan might decrease Abeta accumulation by promoting proteosome activity. In addition, fucoidan treatment reduced the production of ROS stimulated by Abeta induction. These results suggested that fucoidan might exert its protective effect against Abeta-induced toxicity in AD transgenic C. elegans by reducing the accumulation of toxic Abeta and decreasing Abeta-induced production of ROS.
Materials and methods
Chemicals and reagents
Fucoidan polysaccharide sulfate (Fucoidan, FPS) was obtained from Kangyue Biotech (Xian, China). Thioflavine S, Suc-LLVY-AMC, 1,1,1,3,3,3-hexafluoro-2-propanol and 2′,7′-dichlorodihydrofluorescein diacetate (DCFH-DA) were purchased from Sigma Chemical (St Louis, MO, USA). The reverse-transcription reaction system was purchased from Invitrogen (Eugene, OR, USA). Congo red was purchased from Solarbio Science & Technology (Beijing, China). The Brandford protein assay kit and qPCR reaction system were purchased from Sangon Biotech (Shanghai, China). The BeyoECL plus Western blotting detection system and Tween-20 were purchased from Beyotime Institute of Biotechnology (Haimen, China). 4% paraformaldehyde was purchased from Beijing Cellchip Biotechnology.
Nematode strains and maintenance
AD transgenic worms GMC101 were cultured at 16 °C on solid nematode growth medium (NGM) seeded with E. coli (OP50). To harvest age-synchronized animals, worms were transferred to the NGM plate without OP50 to lay eggs overnight and were then removed. Synchronized worms newly hatched from the eggs were transferred to a new NGM plate until they reached the L4-stage.
Paralysis assays
Synchronized L1-stage worms were cultured on NGM plates for 41 h at 16 °C. The L4-stage worms were then cultured with or without different concentrations of fucoidan for 31 h at 16 °C before the temperature shifted from 16 °C to 25 °C. Worms that could move the head but not the body in response to a gentle touch by using a platinum loop were scored as paralyzed.
Fluorescence staining of β-amyloid plaques
For detecting amyloid plaques in the transgenic C. elegans, age-synchronized worms cultured with or without fucoidan as described before were harvested in PBS containing 1% Tween-20 (PBS-T), washed 3 times with 1% PBS-T and fixed in 4% paraformaldehyde in PBS for 24 h at 4 °C. The worms were then permeabilized in 125 mM Tris (pH 7.5) containing 1% Triton X-100 and 5% β-mercaptoethanol for 24 h at 4 °C. The worms were washed 3 times with 1% PBS-T, mounted on a glass slide and stained with 0.125% thioflavin S in 50% ethanol for 2 min. For Congo red staining, the fixed worms were frozen at −80 °C and thawed at 37 °C 3 times before permeabilizing in 125 mM Tris (pH 7.5) containing 1% Triton X-100 and 5% β-mercaptoethanol. After permeabilization, the worms were incubated first in a borate buffer solution (25 mM H3BO3 and 0.01% Triton X-100, pH 9.5) containing 10 mM DTT for 15 min. The worms were then washed and incubated in borate buffer solution containing 0.3% H2O2 for 15 min. The worms were stained with 0.5% Congo red in 50% ethanol/10 mM Tris (ph7.5) at room temperature for 3 h. The staining solution was removed and the worms were destained in 0.5% potassium hydroxide in 80% ethanol. The fluorescence was examined using an Olympus BX53 fluorescence microscope. For quantification, the number of amyloid plaques in the head region (anterior of the pharyngeal bulb) of individual worms was counted. A total of 25 worms were counted for each group. The data were the representatives of at least 3 independent experiments.
Assay of ROS production
The production of ROS was measured using 2′,7′-dichlorodihydrofluorescein diacetate (H2DCF-DA). Synchronized AD transgenic worms cultured on NGM plates with or without fucoidan (500 ng ml−1) as described before were collected at 60 h after Abeta was induced. The worms were washed 3 times with M9 buffer and incubated in PBS-T containing H2DCF-DA (50 μM) for 3 h in the dark with vortexing every ten minutes. The fluorescence intensity was measured by using a fluorospectrophotometer (F-2700 Techcomp (China) Ltd) at 37 °C (excitation wavelength: 485 nm; emission wavelength: 530 nm).
RT-PCR analyses in AD transgenic C. elegans
Synchronized AD transgenic worms cultured on NGM plates with or without fucoidan (500 ng ml−1) as described before were harvested at 60 h after Abeta was induced. Total RNA was extracted with TRIzol Reagent according to the manufacturer's protocol. cDNA was synthesized with 1 μg RNA using reverse transcriptase and the quantitative real-time PCR was performed to determine the gene expression of pbs-1, pbs-2, pbs-5 and hsp16.2-2. The expression of pmp-3 gene was used as the internal control. The sequences of forward and reverse primers used were: TGTAGAGGAATTGGCGGA and TGTGCCGTTCTGGATCTG for pbs-1; TCTCGGCTTCATCTTTCGT and GCCCACATCTTTATATGTGCTC for pbs-2; GGCAAACTCGTACCACAG and CCACTTATCGGGATTCTGG for pbs-5; ACGCCAATTTGCTCCAGTCT and GATGGCAAACTTTTGATCATTGTTA for hsp 16.2; AGTGTCGGCGTTCCAGATTTC and GTCGACGAATCTTGCGAATCA for skn-1; gttcccgtgttcatcactcat and acaccgtcgagaagctgtaga for pmp-3.
Dot blotting analyses of Abeta species in transgenic C. elegans and in vitro amyloid fibrillization
Protein extraction was performed following a previously published method.23 Worms were boiled at 100 °C for 10 min in a lysis buffer (62 mM Tris-HCl pH 6.8, 2% SDS (w/v), 10% glycerol (v/v), 4% β-mercaptoethanol (v/v) and 1× protease inhibitor cocktail) and the lysates were centrifuged at 13
000g at 4 °C for 10 min. The supernatant was collected and the protein concentration was determined using the Bradford Reagent. Equal amounts of protein were loaded on a 0.22 μm nitrocellulose filter membrane (EMD Millipore Corporation) and incubated at 37 °C for 40 min. The membrane was blocked for 1 h at room temperature with 5% (w/v) BSA in 1× Tris buffered saline with Tween-20 (TBS-T, 0.05 M Tris-HCl, 0.15 M NaCl, 0.1% (v/v) Tween-20, pH 7.5) and then incubated with the primary antibody (1
:
1000 dilution, 6E10, Convance) overnight at 4 °C. For measuring amyloid fibrillization in vitro, Abeta42 peptide (GL Biochem Ltd, Shanghai, China) was dissolved in hexafluoropropanol. The solvent was evaporated and the peptide was redissolved in ammonium hydroxide. The Abeta 42 peptide solution was then diluted with PBS to 30 μM and filtered through a 0.22 μm filter. The prepared Abeta 42 solution was mixed with or without different concentrations of fucoidan and incubated at 37 °C for 6 d. After incubation, 1.5 μl of samples were spotted onto a 0.22 μm nitrocellulose filter membrane and incubated at 37 °C for 40 min. The membrane was blocked for 1 h at room temperature with 5% (w/v) milk in 1× TBS-T and then incubated with an anti-amyloid fibril antibody (Millipore) for 2 h at room temperature. For detection, the membrane was incubated with a horseradish peroxidase-coupled secondary antibody, followed by an enhanced chemiluminescence substrate reaction using the BeyoECL plus Western blotting detection system. Ponceau S (0.1%, w/v) solution (Beyotime) was used to stain the blotted membranes for visualization of total proteins. The densitometry analyses were performed with Quantity One software.
Proteasome activity assay
Proteasome activity assay was performed following a previously publish method with slight modification.24 Worms were sonicated in a lysis buffer (50 mM HEPES, 150 mM NaCl, 5 mM EDTA, 2 mM DTT, 2.5 mM sodium pyrophosphate, 20 mM NaF, 1 mM α-glycerophosphoric acid and 1 mM sodium orthovanadate) and then centrifuged at 13
000g at 4 °C for 10 min. The supernatant was collected and the protein concentration was determined using the Bradford Reagent. 20 μg of total protein was mixed with 140 μM Suc-LLVY-AMC in a proteasome activity assay buffer (50 mM HEPES pH 7.4, 150 mM NaCl, 5 mM EDTA, and 5 mM ATP) at room temperature. The fluorescence was measured every 10 min over 3 h with an excitation at 360 nm and an emission at 460 nm, using a Synergy HTX Multi-Mode Microplate Reader (BioTek Instruments, Inc.). To calculate the proteasome-specific enzyme activity, the assay was performed in the absence and presence of the proteasome inhibitor MG132 (10 μM, Selleck Chemicals).
Statistical analyses
Statistical analysis of the data was performed using one-way ANOVA. The least significant difference (LSD) test was used for pairwise comparisons. P values of 0.05 were considered statistically significant.
Results
Fucoidan alleviates Abeta-induced paralysis in the transgenic AD C. elegans
To evaluate the effects of fucoidan on Abeta-induced toxicity, transgenic C. elegans was treated with different concentrations of fucoidan (0–2500 ng ml−1) and Abeta-induced paralysis was examined. The results showed that fucoidan (100–500 ng ml−1) was effective in delaying the onset of paralyzed phenotype of the transgenic AD animals induced by Abeta (Fig. 1A). And 500 ng ml−1 of fucoidan appeared to provide the best protection against the Abeta-induced paralyzed phenotype. As shown in Fig. 1B, control treated worms exhibited a paralyzed phenotype at 24 h after Abeta induction, while the fucoidan (500 ng ml−1) treated group did not show a significant increase in the number of paralyzed animals until 36 hours after Abeta induction. And starting at 36 h after a temperature up-shift, the percentage of the animals with the paralyzed phenotype was significantly lower in fucoidan treated group in comparison with the control group. At 96 h after Abeta-induction, it was found that approximately 78% of worms were paralyzed in the control treated group, while only about 46% of worms were paralyzed in the fucoidan treated group. The data also suggested that fucoidan had a biphasic effect on the inhibition of Abeta-induced toxicity in AD C. elegans. The optimal dose for the protective effects of fucoidan was around 500 ng ml−1. The lower or higher doses of fucoidan were less effective for inhibiting Abeta-induced toxicity.
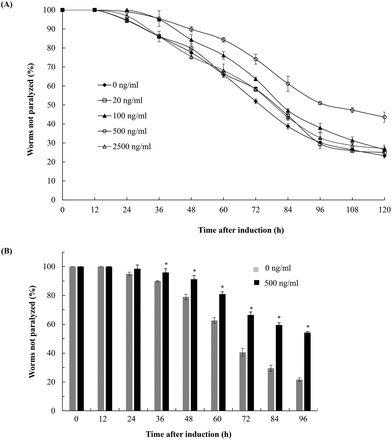 |
| Fig. 1 Effects of fucoidan on the Abeta-induced paralysis of transgenic AD C. elegans. (A) Worms synchronized to the L4 stage were treated with different concentrations of fucoidan (0, 20, 100, 500 and 2500 ng ml−1) for 31 h at 16 °C. The temperature was then up-shifted from 16 °C to 25 °C to induce Abeta. The number of paralyzed worms was counted at a 12 h interval. (B) Synchronized AD worms were cultured with or without 500 ng ml−1 fucoidan for 31 h before the temperature shift. The number of paralyzed worms was then counted at a 12 h interval. Data are expressed as mean ± SD. *Significantly different from untreated worms, p < 0.05. | |
Fucoidan effectively decreases Abeta deposition in the transgenic C. elegans
Next, we studied whether fucoidan affected the accumulation of Abeta by examining the amyloid deposits in the transgenic C. elegans using thioflavin S staining. As shown in Fig. 2, in the transgenic worms, there was a significant increase in the number of amyloid deposits when the temperature was up shifted from 16 °C to 25 °C. At 60 h after Abeta induction, fucoidan treatment caused an approximate 47% of decrease in the number of amyloid deposits when compared with the control group (Fig. 2A). Similarly, the decrease of amyloid deposition by fucoidan treatment was observed at 108 h after Abeta induction. As shown in Fig. 2B, the number of amyloid deposits in the fucoidan treated group was about 52% lower than that in the control group. To confirm the results obtained from thioflavin S staining, we stained the worms with Congo red. As demonstrated in Fig. 2C, the number of Congo red positive Abeta deposits was significantly elevated after the temperature upshift, while fucoidan treatment prevented the increase of the number of Abeta deposits. Overall, these data indicated that fucoidan treatment might have a significant inhibitory effect on the accumulation of Abeta deposit in AD C. elegans.
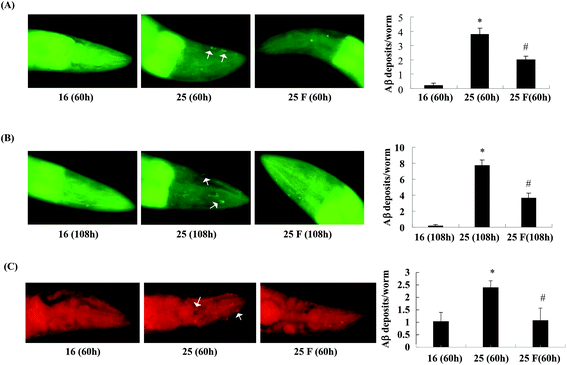 |
| Fig. 2 Effects of Fucoidan on amyloid deposition in transgenic AD C. elegans. Worms synchronized to the L4 stage were treated with or without fucoidan (500 ng ml−1) for 31 h at 16 °C. The temperature was then up-shifted from 16 °C to 25 °C. The worms were collected at 60 h (A) or 108 h (B) after the temperature up shift and stained with thioflavin S. (C) The worms were collected at 60 h after the temperature up shift and stained with Congo red. The number of amyloid plaques in the head region of individual worms was counted. Data are expressed as mean ± SE. Arrows point to the representative amyloid deposits. *Significantly different from control worms that were cultured at 16 °C (p < 0.05); #significantly different from untreated worms (p < 0.05). F: fucoidan. | |
To investigate the effect of fucoidan on amyloid aggregation in vitro, we incubated the Abeta42 peptide with fucoidan and performed dot blot analyses to estimate the level of Abeta aggregation using an antibody that recognizes amyloid fibrils but not prefibrillar oligomers or natively folded proteins. We observed that the level of amyloid fibrils was elevated after 6 d of incubation, the increase of amyloid fibrils was prevented when fucoidan was presented during Abeta incubation (Fig. 3). The data suggested that fucoidan might affect amyloid aggregation in vitro. Thus, it was possible that the reduction of Abeta deposition observed in fucoidan-treated AD C. elegans was caused by a direct decrease of Abeta fibrillization by fucoidan.
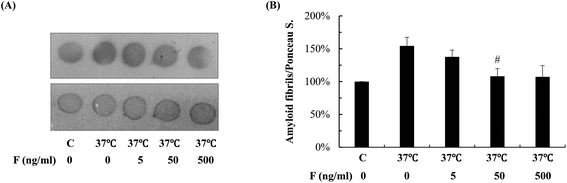 |
| Fig. 3 Effects of fucoidan on Abeta aggregation in vitro. (A) Abeta42 peptide prepared as described in “Materials and methods” was incubate with or without different concentrations of fucoidan at 37 °C for 6 d. After incubation, 1.5 μl of samples were spotted onto the nitrocellulose filter membrane and the dot blotting was performed using an anti-amyloid fibrils antibody (top panel). Total protein on the blotted membranes was stained with 0.1% Ponceau S. solution (bottom panel). Results are representative from three experiments. (B) The intensities of the dots were quantified by densitometric analyses. Data are expressed as mean ± SE. (n = 3). The levels of amyloid fibrils in Abeta42 peptide solution before incubation at 37 °C were set as 100%. #Significantly different from samples incubated with 0 ng ml−1 of fucoidan (p < 0.05). F: fucoidan. C: Abeta42 peptide solution stored at −20 °C without incubation at 37 °C. | |
Fucoidan decreases Abeta levels in the transgenic C. elegans and promotes proteasome activity
The reduction of Abeta deposition might also be a result from the decrease of Abeta levels in fucoidan-treated AD C. elegans. We next examined Abeta levels in the transgenic AD worms treated with or without fucoidan using dot blot analyses. As shown in Fig. 4A, the amount of Abeta protein significantly increased upon the temperature shift from 16 °C to 25 °C, while fucoidan significantly decreased the protein level of Abeta, causing 15% and 50% decrease by treatment with 100 and 500 ng ml−1 fucoidan, respectively (Fig. 4B). C. elegans express an APP ortholog, APL-1 (amyloid precursor-like-1), which lacks beta-site amyloid precursor protein cleaving enzyme (BACE) sites. The BACE ortholog has not been found in the genome of C. elegans.22 Therefore, the reduction of Abeta in fucoidan-treated AD C. elegans was not likely a result from changes in APP processing. The proteasome is a large protein complex responsible for the degradation of misfolded proteins by proteolysis.25 To examine the effect of fucoidan on proteasome activity, the chymotrypsin-like activity of the proteasome was determined by measuring the hydrolysis of fluorogenic peptide Suc-LLVY-AMC. It was found that Abeta induction caused a significant reduction in the activity of proteasome, while fucoidan treatment prevented the Abeta-induced decrease of proteasome activity (Fig. 5A). When compared to that of the untreated group, 100 and 500 ng ml−1 fucoidan treatment increased the activity of proteasomes by 2 and 3.1-fold, respectively (Fig. 5B). Moreover, it was found that the expression of the catalytic 20S core proteasome subunits pbs-1, pbs-2 and pbs-5 genes was reduced by Abeta-induction, while fucoidan treatment significantly inhibited the decrease of pbs-2 and pbs-5 gene expression caused by Abeta-induction (Fig. 5C).
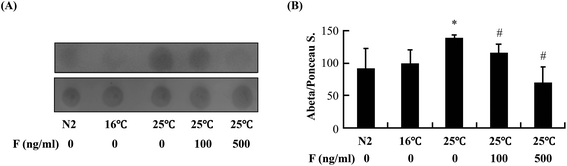 |
| Fig. 4 Effects of fucoidan on the levels of Abeta in transgenic AD C. elegans. (A) Worms treated with different concentrations of fucoidan (0, 100 ng ml−1, 500 ng ml−1) as described in “Materials and methods”. The worms was collected at 60 h after the temperature was up-shifted from 16 °C to 25 °C and protein extracts were prepared. Equal amounts of proteins were loaded on nitrocellulose membrane and dot blotting was performed using 6E10 antibody (top panel). Total proteins on the blotted membranes were stained with a 0.1% Ponceau S. solution (bottom panel) and were used as the protein control. Results are representative from three independent experiments. (B) The intensities of the dots were quantified by densitometric analyses. Data are expressed as mean ± SD. *Significantly different from control worms that were cultured at 16 °C (p < 0.05); #significantly different from worms treated with 0 ng ml−1 of fucoidan (p < 0.05). N2: wild type animal; F: fucoidan. | |
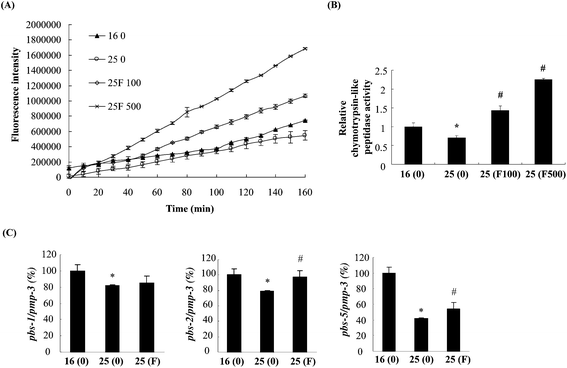 |
| Fig. 5 Effects of fucoidan on proteasome activities in transgenic AD C. elegans. (A) Worms treated with different concentrations of fucoidan (0, 100 ng ml−1, 500 ng ml−1) as described in “Materials and methods”. The worms were collected at 60 h after the temperature was up-shifted from 16 °C to 25 °C. Protein extracts were prepared and incubated with Suc-LLVY-AMC. The cleavage of the fluorogenic peptide was examined by measuring the fluorescence every 10 min. (B) Proteasomal activities were calculated as the rate of the cleavage of the substrate. (C) Worms treated with or without fucoidan (500 ng ml−1) were collected at 60 h after the temperature was up-shifted. Total RNA was prepared and the quantitative real-time RT-PCR was performed to examine the gene expression of pbs-1, pbs-2 and pbs-5. The gene pmp-3 was used as the internal control. Data are expressed as mean ± SD. *Significantly different from control worms that were cultured at 16 °C (p < 0.05); #significantly different from worms treated with 0 ng ml−1 of fucoidan (p < 0.05). Values obtained from control worms that were cultured at 16 °C were set to 100%. F100: fucoidan 100 ng ml−1; F500: fucoidan 500 ng ml−1. | |
Fucoidan suppresses Abeta-induced elevation of ROS production and ameliorates Abeta-induced reduction of hsp16.2 expression in transgenic C. elegans
Evidence has shown that oxidative stress plays an important role in the toxicity induced by Abeta.26 As fucoidan has been reported to have antioxidative properties,15,19 we next examined the effects of fucoidan on Abeta-induced elevation of ROS. As shown in Fig. 6A, the levels of ROS were dramatically increased at 60 h after the temperature up-shift, while treatment with 500 ng ml−1 fucoidan significantly reduced Aβ-induced elevation of ROS by approximately 90%. Skinhead-1 (skn-1) is a transcription factor that plays an important role in antioxidant defense. Abeta-induction slightly increased the expression of skn-1 while fucoidan treatment reduced the increase of skn-1 expression induced by Abeta; however, neither of the effects was statistically significant (Fig. 6B).
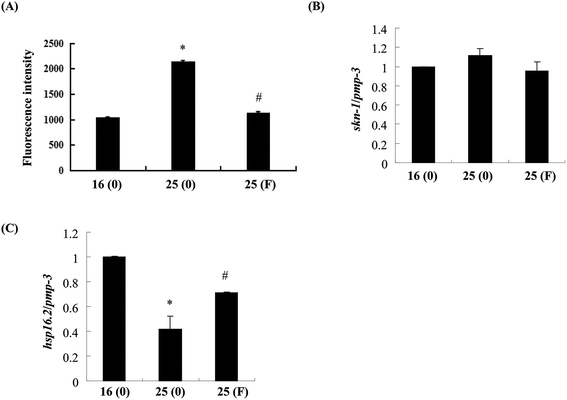 |
| Fig. 6 Effects of fucoidan on ROS production and hsp16.2 gene expression in transgenic AD C. elegans. (A) The levels of ROS were measured in AD worms treated with or without fucoidan (500 ng ml−1) as described in “Materials and methods”. Data are expressed as mean ± SD. *Significantly different from control worm that were cultured at 16 °C (p < 0.05); #significantly different from worms treated with 0 ng ml−1 of fucoidan (p < 0.05). Worms treated with or without fucoidan (500 ng ml−1) were collected at 60 h after the temperature was up-shifted from 16 °C to 25 °C. Total RNA was prepared and the quantitative real-time PCR was performed to examine the gene expression of skn-1 (B) and hsp16.2 (C). The gene pmp3 was used as the internal control. Data are expressed as mean ± SD. Values obtained from control worms that were cultured at 16 °C were set to 1. *Significantly different from control worm that were cultured at 16 °C (p < 0.05); #significantly different from worms treated with 0 ng ml−1 of fucoidan (p < 0.05). F: fucoidan. | |
Small heat shock proteins are chaperon proteins induced by stress conditions including oxidative stress and protein damage.27 Quantitative real-time PCR results showed that Abeta induction decreased the expression of small heat shock protein hsp16.2-2 by approximately 51%, while fucoidan treatment ameliorated Abeta-induced suppression of hsp16.2-2 expression by about 46% (Fig. 6C).
Discussion
Abeta is the major component of senile plaque that is one of the most prominent pathological characteristics of AD. The imbalance between Abeta production and clearance in the CNS leads to the accumulation of Abeta, which has been shown to stimulate diverse cell signaling pathways, eventually resulting in synaptic degeneration, neuronal loss and decline in cognitive function.7–9,28 Fucoidan, a complex sulfated polysaccharide that was first identified in brown seaweeds, has been shown to have a wide variety of biological activities.13–18 Here, we evaluated the effects of fucoidan on Abeta-induced toxicity in a transgenic C. elegans AD model GMC101. It was found that fucoidan effectively protected the AD transgenic C. elegans against the Abeta-induced paralyzed phenotype.
Further analysis revealed that fucoidan treatment significantly decreased the level of Abeta and reduced the number of Abeta deposits in the transgenic C. elegans. Thus, the reduction of Abeta by fucoidan might be an important mechanism by which fucoidan ameliorates the paralyzed phenotype in AD animals. When proteasome activity was examined, we observed that fucoidan treatment significantly elevated the activity of proteasome in AD C. elegans, which was compromised by Abeta induction. Proteasome, which functions in catalyzing intracellular protein degradation, is the major component of the ubiquitin-proteasome system (UPS) that plays an essential role in maintaining protein homeostasis in eukaryotic cells.25,29 It has been reported that proteasome activity is decreased in the brains of patients with neurodegenerative diseases such as AD,30,31 while Abeta treatment can markedly decrease the proteasome activity in cultured neuronal cells.32 The impaired proteasome function may prevent the degradation of oxidized/damaged/misfolded proteins, causing the accumulation of unwanted proteins and their aggregation.25,29 Therefore, the decrease of proteasome activity decelerates the clearance of Abeta deposition in the pathogenesis of AD, creating a vicious cycle that increases Abeta accumulation. Evidence suggests that targeting proteasome activity may offer a strategy to reduce the levels of aberrant proteins such as Abeta in AD.29,33,34 By promoting the activity of proteasomes, fucoidan might enhance the degradation of Abeta, reducing the number of Abeta deposits and eventually delaying the development of the paralyzed phenotype. In fact, it was reported that the overexpression of proteasome subunits pbs-5 delayed the paralyzed phenotype in transgenic AD C. elegans.35 In our study, it was found that fucoidan treatment significantly inhibited the reduction of the gene expression of the catalytic 20S core proteasome subunits pbs-2 and pbs-5 by Abeta-induction. Thus, the enhancement of proteasome activity and inhibition of Abeta-induced paralysis may be contributed partly by the increase of the expression of proteasome subunits.
The result from in vitro assay of amyloid fibrillization suggested that fucoidan might also directly affect the fibrillization of Abeta. Therefore, it was possible that the reduction of Abeta deposition observed in fucoidan-treated AD C. elegans was contributed by a direct interference of fucoidan on Abeta fibrillization.
Oxidative stress plays a key role in the pathological development of AD.26,36 Multiple lines of evidence indicate that cellular damage resulting from oxygen free radicals may be a major contributor to the toxicity of Abeta.26,36,37 Here, we observed that fucoidan treatment significantly reduced the production of ROS induced by Abeta in the AD worms. This may be an important mechanism by which fucoidan inhibits the development of the paralyzed phenotype induced by Abeta. SKN-1 is a transcription factor that has been implicated in the induction of genes involved in resistance to oxidative stress.38 We did not see a significant impact of fucoidan on the gene expression of skn-1 under the current experimental condition. It is possible that the reduction of ROS levels in the AD worms by fucoidan treatment is at least partly contributed by a direct antioxidant action of fucoidan.
Small heat shock proteins are part of an integrated network that is crucial for protein quality control and the maintenance of proteome homeostasis.27 These chaperone proteins play key roles in preventing the formation of toxic aggregates of aberrant proteins and assisting their degradation. The overexpression of small heat shock protein HSP-16.2 in C. elegans AD models has been shown to partially suppress the Abeta-induced paralyzed phenotype.39 Thus, the up-regulation of hsp-16.2 gene expression by fucoidan also contributes to the protective effects of fucoidan against Abeta-induced toxicity in the AD C. elegans.
Conclusion
In summary, our results showed that fucoidan treatment protected Abeta-induced toxicity in the transgenic AD C. elegans model. And fucoidan may exert its function mainly through the promotion of proteosome activity thus reducing the accumulation of Abeta and by alleviating the oxidative stress. Recently, it was reported that oral administration of fucoidan significantly improved the spatial cognition in a rat model that learning and memory impairment was induced by the infusion of Abeta (1–40) into hippocampus.40 Collectively, the evidence suggests that fucoidan may be useful as part of pharmaceuticals or functional foods to provide neuroprotection in neurodegenaration diseases such AD.
Conflicts of interest
The authors declare that there is no conflict of interest.
Acknowledgements
This work is supported by the National Natural Science Foundation of China (31201338 and 31371082), Shandong province key R & D Program project (2015GSF115014), research fund from Harbin Institute of Technology at Weihai [HIT(WH)Y200902] and Weihai Science and Technology Development Program (2009-3-93).
References
- H. W. Querfurth and F. M. LaFerla, Alzheimer's disease, N. Engl. J. Med., 2010, 362(4), 329–344 CrossRef CAS PubMed.
- R. N. Kalaria, G. E. Maestre, R. Arizaga, R. P. Friedland, D. Galasko, K. Hall, J. A. Luchsinger, A. Ogunniyi, E. K. Perry, F. Potocnik, M. Prince, R. Stewart, A. Wimo, Z. X. Zhang and P. Antuono, Alzheimer's disease and vascular dementia in developing countries: prevalence, management, and risk factors, Lancet Neurol., 2008, 7(9), 812–826 CrossRef PubMed.
- Alzheimer's Association, Alzheimer's disease facts and figures, Alzheimers Dement., 2010, 6(2), 158–194.
- M. Awasthi, S. Singh, V. P. Pandey and U. N. Dwivedi, Alzheimer's disease: An overview of amyloid beta dependent pathogenesis and its therapeutic implications along with in silico approaches emphasizing the role of natural products, J. Neurol. Sci., 2016, 361, 256–271 CrossRef CAS PubMed.
- S. Cinzia, P. Carla and C. Pietro, Substance P and Alzheimer's Disease: Emerging Novel Roles, Curr. Alzheimer Res., 2016, 13(9), 964–972 CrossRef.
- J. C. Vickers, T. C. Dickson, P. Adlard, H. L. Saunders, C. E. King and G. McCormack, The cause of neuronal degeneration in Alzheimer's disease, Prog. Neurobiol., 2000, 60(2), 139–165 CrossRef CAS PubMed.
- J. Hardt and D. J. Selkoe, The amyloid hypothesis of Alzheimer's disease: progress and problems on the road to therapeutics, Science, 2002, 297(5580), 353–356 CrossRef PubMed.
- G. Kwasi, W. Sigurdson, V. Ovod, L. Munsell, T. Kasten, J. C. Morris, K. E. Yarasheski and R. J. Bateman, Decreased clearance of CNS beta-amyloid in Alzheimer's disease, Science, 2010, 330(6012), 1774–1777 CrossRef PubMed.
- V. Nimmrich and U. Ebert, Is Alzheimer's disease a result of presynaptic failure? Synaptic dysfunctions induced by oligomeric beta-amyloid, Rev. Neurosci., 2009, 20(1), 1–12 CrossRef CAS PubMed.
- B. A. Yankner and T. Lu, Amyloid beta-protein toxicity and the pathogenesis of Alzheimer disease, J. Biol. Chem., 2009, 284(8), 4755–4759 CrossRef CAS PubMed.
- E. D. Roberson and L. Mucke, 100 years and counting: prospects for defeating Alzheimer's disease, Science, 2006, 314(5800), 781–784 CrossRef PubMed.
- K. Hsiao, P. Chapman, S. Nilsen, C. Eckman, Y. Harigaya, S. Younkin, F. Yang and G. Cole, Correlative memory deficits, Abeta elevation, and amyloid plaques in transgenic mice, Science, 1996, 274(5284), 99–102 CrossRef CAS PubMed.
- B. Li, F. Lu, X. Wei and R. Zhao, Fucoidan: Structure and bioactivity, Molecules, 2008, 13(8), 1671–1695 CrossRef CAS PubMed.
- W. Zhang, T. Oda, Q. Yu and J. O. Jin, Fucoidan from Macrocystis pyrifera Has Powerful Immune-Modulatory Effects Compared to Three Other Fucoidans, Mar. Drugs, 2015, 13(3), 1084–1104 CrossRef CAS PubMed.
- J. Wang, Q. Zhang, Z. Zhang, H. Song and P. Li, Potential antioxidant and anticoagulant capacity of low molecular weight fucoidan fractions extracted from Laminaria japonica, Int. J. Biol. Macromol., 2010, 46(1), 6–12 CrossRef CAS PubMed.
- L. Yang, P. Wang, H. Wang, Q. Li, H. Teng, Z. Liu, W. Yang, L. Hou and X. Zou, Xiangyang, Fucoidan Derived from Undaria pinnatifida Induces Apoptosis in Human Hepatocellular Carcinoma SMMC-7721 Cells via the ROS-Mediated Mitochondrial Pathway, Mar. Drugs, 2013, 11(6), 1961–1976 CrossRef PubMed.
- O. Berteau and B. Mulloy, Sulfated fucans, fresh perspectives: structures, functions, and biological properties of sulfated fucans and an overview of enzymes active toward this class of polysaccharide, Glycobiology, 2003, 13(6), 29R–40R CrossRef CAS PubMed.
- H. Y. Park, M. H. Han, C. Park, C. Y. Jin, G. Y. Kim, I. W. Choi, N. D. Kim, T. J. Nam, T. K. Kwon and Y. H. Choi, Anti-inflammatory effects of fucoidan through inhibition of NF-κB, MAPK and Akt activation in lipopolysaccharide-induced BV2 microglia cells, Food Chem. Toxicol., 2011, 49(8), 1745–1752 CrossRef CAS PubMed.
- Y. Gao, C. Dong and J. Yin, Neuroprotective effect of fucoidan on H2O2-induced apoptosis in PC12 cells via activation of PI3 K/Akt pathway, Cell. Mol. Neurobiol., 2012, 32(4), 523–529 CrossRef CAS PubMed.
- Y. Ha, Y. Kim, H. Lee, J. Seo, Y. Sung, S. Kim, M. Shin and C. Kim, Neuroprotective Effect of Fucoidan against N-methyl-D-aspartate-Induced Excitotoxicity in Rat Hippocampus, Exp. Neurobiol., 2009, 18(2), 123–128 CrossRef.
- J. H. Jhamandas, M. B. Wie, K. Harris, D. MacTavish and S. Kar, Fucoidan inhibits cellular and neurotoxic effects of beta amyloid (A beta) in rat cholinergic basal forebrain neurons, Eur. J. Neurosci., 2005, 21(10), 2649–2659 CrossRef PubMed.
- G. McColl, B. R. Roberts, T. L. Pukala, V. B. Kenche, C. M. Roberts, C. D. Link, T. M. Ryan, C. L. Masters, K. J. Barnham, A. I. Bush and R. A. Cherny, Utility of an improved model of amyloid-beta (Aβ1–42) toxicity in Caenorhabditis elegans for drug screening for Alzheimer's disease, Mol. Neurodegener., 2012, 7, 57 CrossRef CAS PubMed.
- J. S. Sangha, X. Sun, O. Wally, K. Zhang, X. Ji, Z. Wang, Y. Wang, J. Zidichouski, B. Prithiviraj and J. Zhang, Liuwei Dihuang (LWDH), a Traditional Chinese Medicinal Formula, Protects against β-Amyloid Toxicity in Transgenic Caenorhabditis elegans, PLoS One, 2012, 7(8), e43990 CAS.
- J. Pispa, S. Palmén, C. I. Holmberg and J. Jäntti, C. elegans dss-1 is functionally conserved and required for oogenesis and larval growth, BMC Dev. Biol., 2008, 8(1), 1–13 CrossRef PubMed.
- E. K. Schrader, K. G. Harstad and A. Matouschek, Targeting proteins for degradation, Nat. Chem. Biol., 2009, 5(11), 815–822 CrossRef CAS PubMed.
- W. R. Markesbery, Oxidative stress hypothesis in Alzheimer's disease, Free Radical Biol. Med., 1997, 23(1), 134 CrossRef CAS PubMed.
- M. R. Leroux, R. Melki, B. Gordon, G. Batelier and E. P. Candido, Structure-function studies on small heat shock protein oligomeric assembly and interaction with unfolded polypeptides, J. Biol. Chem., 1997, 272(39), 24646–24656 CrossRef CAS PubMed.
- S. Tu, S. Okamoto, S. A. Lipton and H. Xu, Oligomeric Aβ-induced synaptic dysfunction in Alzheimer's disease, Mol. Neurodegener., 2014, 9, 48 CrossRef PubMed.
- C. McKinnon and S. J. Tabrizi, The Ubiquitin-Proteasome System in Neurodegeneration, Antioxid. Redox Signaling, 2014, 21(17), 2302–2321 CrossRef CAS PubMed.
- J. N. Keller, K. B. Hanni and W. R. Markesbery, Impaired Proteasome Function in Alzheimer's Disease, J. Neurochem., 2000, 75(1), 436–439 CrossRef CAS PubMed.
- M. López Salon, L. Morelli, E. M. Castaño, E. F. Soto and J. M. Pasquini, Defective ubiquitination of cerebral proteins in Alzheimer's disease, J. Neurosci. Res., 2000, 62(2), 302 CrossRef.
- S. Oh, H. Hong, E. Hwang, H. Sim, W. Lee, S. Shin and I. Mook-Jung, Amyloid peptide attenuates the proteasome activity in neuronal cells, Mech. Ageing Dev., 2005, 126(12), 1292–1299 CrossRef CAS PubMed.
- B. Lee, M. Lee, S. Park, D. Oh, S. Elsasser, P. Chen, C. Gartner, N. Dimova, J. Hanna, S. P. Gygi, S. M. Wilson, R. W. King and D. Finley, Enhancement of proteasome activity by a small-molecule inhibitor of USP14, Nature, 2010, 467(7312), 179–184 CrossRef CAS PubMed.
- F. H. Dal Vechio, F. Cerqueira, O. Augusto, R. Lopes and M. Demasi, Peptides that activate the 20S proteasome by gate opening increased oxidized protein removal and reduced protein aggregation, Free Radical Biol. Med., 2014, 67, 304–313 CrossRef CAS PubMed.
- N. Chondrogianni, K. Georgila, N. Kourtis, N. Tavernarakis and E. S. Gonos, 20S proteasome activation promotes life span extension and resistance to proteotoxicity in Caenorhabditis elegans, FASEB J., 2015, 29(2), 611–622 CrossRef CAS PubMed.
- V. Shukla, S. K. Mishra and H. C. Pant, Oxidative Stress in Neurodegeneration, Adv. Pharmacol. Sci., 2011, 2011(1–2), 572634–572634 Search PubMed.
- D. A. Butterfield, B. Howard, S. Yatin, T. Koppal, J. Drake, K. Hensley, M. Aksenova, R. Subramaniam, S. Varadarajan, M. E. Harris-White, N. W. Pedigo Jr. and J. M. Carney, Elevated oxidative stress in models of normal brain aging and Alzheimer's disease, Life Sci., 1999, 65(18–19), 1883–1892 CrossRef CAS PubMed.
- J. H. An and T. K. Blackwell, SKN-1 links C. elegans mesendodermal specification to a conserved oxidative stress response, Genes Dev., 2003, 17(15), 1882–1893 CrossRef CAS PubMed.
- V. Fonte, D. R. Kipp, J. Yerg 3rd, D. Merin, M. Forrestal, E. Wagner, C. M. Roberts and C. D. Link, Suppression of in vivo beta-amyloid peptide toxicity by overexpression of the HSP-16.2 small chaperone protein, J. Biol. Chem., 2008, 283(2), 784–791 CrossRef CAS PubMed.
- Y. Gao, C. Li, J. Yin, J. Shen, H. Wang, Y. Wu and H. Jin, Fucoidan, a sulfated polysaccharide from brown algae, improves cognitive impairment induced by infusion of Aβ peptide in rats, Environ. Toxicol. Pharmacol., 2012, 33(2), 304–311 CrossRef CAS PubMed.
|
This journal is © The Royal Society of Chemistry 2018 |