DOI:
10.1039/C8CS90055H
(Editorial)
Chem. Soc. Rev., 2018,
47, 3391-3394
Peptide and protein nanotechnology into the 2020s: beyond biology
Received
8th May 2018
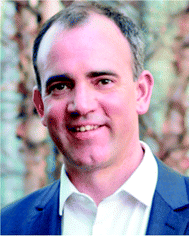
Rein V. Ulijn
| Rein Ulijn is the founding Director of the Nanoscience Initiative at the Manhattan-based Advanced Science Research Center (ASRC) at the Graduate Center of the City University of New York (CUNY). He is also a Professor of Nanochemistry at Hunter College and a visiting Professor at the University of Strathclyde, UK. The Ulijn research lab researches peptide nanotechnology. We are interested in how function arises from interactions and reactions of (mixtures of) peptide components that can access multiple configurations for the design of new active materials. |
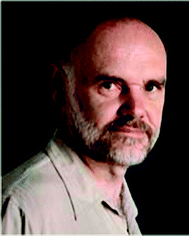
Roman Jerala
| Roman Jerala is head of the Department of Synthetic Biology and Immunology at the National Institute of Chemistry in Ljubljana, Slovenia, and Professor at the University of Ljubljana. Within synthetic biology he is investigating designed modular bionanostructures, particularly coiled-coil based protein origami, mammalian cell synthetic biology and medical applications of synthetic biology, and within immunology he focuses on the molecular mechanisms of signaling in innate immunity and on cancer immunotherapy. |
Why this themed issue?
Proteins and peptides are the most versatile programmable polymers known. They hold the key to fundamental working principles of biology, and, at least in principle, allow the design of materials and structures with functions that match, or even surpass, those of the living world.
Eight years have passed since Chem. Soc. Rev. published a themed issue on Peptide and protein based materials in 2010. Given the tremendous progress made in the design and engineering of protein- and peptide-based (polypeptide-based) functional nanoscopic structures and materials, we felt the time was right for an up-to-date themed issue. In recognition of a notable expansion in scope of the area over the past decade, including a shift from designed structures to designed function, this special issue is entitled Peptide and protein nanotechnology.
Expanding the known universe of polypeptide assemblies
Peptide and protein nanotechnology has its foundations in protein folding by design, either as supramolecular assemblies1 or single chain protein folds,2 and in the recognition that intramolecular (quaternary) interactions can be exploited for the molecular self-assembly of nano- and macroscopic materials, using either biologically derived peptide sequences,3 engineered proteins4 or newly designed sequences not known in the biological world.5
The number of possible polypeptide sequences exceeds the number of atoms in the universe, with a large number of sequences able to fold into defined structures, resembling the unknown potentially habitable planets in the universe. Nature only uses a fraction of the available chemical space, with the vast remainder now becoming accessible through experimental searching and rational design, and through a productive marriage between nature and science.
Advancing tools for understanding, searching, prediction and characterization
In a field that is now well into its third decade, we still cannot predict the tertiary structure of many polypeptides based on amino acid sequence alone, but the design of new structural and functional polypeptide-based nanostructures is now increasingly possible. Indeed, by taking advantage of advanced characterization techniques and continuously improving computational approaches, guiding principles and rules now inform the design of structures with ever-increasing complexity. See also an excellent review on designed proteins by the Baker group.6
The advances in designed polypeptide nanostructure rely a great deal on the development of methodological tools. The last decade has seen many exciting developments in this regard, including the 2013, 2014, 2016 and 2017 Nobel prizes for computational chemistry, superresolution, molecular machines and cryo-electron microscopy. There have also been tremendous developments in molecular simulations relevant to peptide and protein interactions, where the contribution of Marrink's group (Frederix et al., DOI: 10.1039/C8CS00040A) reviews the state of the art in simulations of self-assembling bio-inspired supramolecular systems. Computation and experimentation are increasingly used to complement each other to enable efficient searching of the sequence space for new functions (Lampel et al., DOI: 10.1039/C8CS00177D), including combining simulation protocols, new sampling techniques and force fields as well as simulation of experimentally measurable quantities obtained by scattering, spectroscopic or microscopic techniques. Recently, attention in experimental biophysics has focused on the ability to measure and interpret effects of mechanical forces on proteins during biological processes, which play an important role in the physiological environment of proteins in cells. Perez-Jimenez and co-workers (DOI: 10.1039/C7CS00820A) review the effects of mechanical forces in all steps of the protein lifecycle, from biosynthesis with folding to targeting in order to fight disease. Mechanochemistry can be experimentally applied to single molecules, where it provides insights into many steps of the protein life cycle.
Multi-component systems and complexity
While peptide and protein design typically focuses on identifying specific polypeptide sequences that give rise to certain structures with desirable properties, in the living world, functionality is often obtained by combining, and co-assembling, multiple components, where functionality results from their cooperative assembly. Raymond and Nilsson (DOI: 10.1039/C8CS00115D) review approaches for co-assembly and cooperative effects on structure formation in oligo-peptide assembly, including examples that include non-natural amino acids. Makam and Gazit (DOI: 10.1039/C7CS00827A) review how functionality can be significantly enhanced by using such multi-component peptide ensembles, including those with functions not normally associated with biological systems. When combining multiple building blocks, different modes of assembly are possible and the analysis and characterization of these systems poses new challenges. For example, formation of a self-supporting material is based on crosslinking and entanglement of components, which can be based either on the random or specific associations between the components. Draper and Adams (DOI: 10.1039/C7CS00804J) describe the state of the art of the understanding and control over the assembly of fibrous structures and their entanglement that critically determine the properties of materials. New functional materials can be constructed by multicomponent polypeptide assemblies and various strategies can be used, such as covalent conjugation, specific binding, templated/directed assembly and non-specific co-assembly, which are presented by Okesola and Mata (DOI: 10.1039/C8CS00121A). Beyond the synthesis of functional assemblies per se, peptide based systems provide model systems to address questions around origin of life chemistry.
Measuring, influencing and directing biology
Due to their versatile properties, designed polypeptides have numerous potential biomedical applications for therapy, prevention and diagnostics. Li, Wang and Cui (Zhang et al., DOI: 10.1039/C7CS00793K) summarise recent strategies and approaches adopted for the identification of functional peptides in the context of molecular imaging and disease diagnostics. An important feature of such systems is the ability to transfer through physiological barriers for targeted delivery and improved specificity. Stevens and co-workers (Spicer et al., DOI: 10.1039/C7CS00877E) focus on the use of peptides and proteins as functional components of (inorganic) nanoparticles, for applications in diagnostics and therapeutics based on specific engineerable interactions that underlie the delivery, cellular and tissue distribution and control of biological processes. Within biological and particularly medical applications, cytosolic delivery of proteins remains an important challenge. A contribution by Rotello and co-workers (Scaletti et al., DOI: 10.1039/C8CS00008E) describes strategies for the cellular delivery of proteins including direct assembly, encapsulation and formation of composites, which have to be tailored for the selected protein cargo.
Cages and catalytic materials by design
Natural proteins can form various types of compartments, from viral capsids to micro-compartments in cells. These nanocages are highly complex but can be repurposed and reengineered using genetic tools to introduce new features. Hilvert and co-workers (Azuma et al., DOI: 10.1039/C8CS00154E) describe the engineering of lumazine synthase protein cages that have been modified by targeted engineering of amino acid residues that resulted in cages of different sizes, physicochemical properties that enabled generation of nanoreactors, artificial organelles, delivery vehicles and virus mimetics. Assembly of protein cages, but also the construction of higher order assemblies from cages, as novel functional materials are described by Douglas and co-workers (Aumiller et al., DOI: 10.1039/C7CS00818J). They discuss the properties of protein cages, mechanisms of their assembly and functionalization either of their inner or exterior surfaces. Jerala and co-workers (Lapenta et al., DOI: 10.1039/C7CS00822H) describe the modular de novo construction of protein cages termed coiled-coil protein origami (CCPO), which can produce new protein folds forming diverse polyhedral cages with edges composed of coiled-coil dimer modules. This represents a unique intersection between mathematical concepts formulated by graph theory and protein design. Protein cages are produced in the same way as natural protein folds and can self-assemble in vivo, which suggests that they may find interesting technological and therapeutic applications.
Protein engineering has been used for several decades, aiming to engineer new catalytic and other properties into natural and designed proteins, mainly targeting directly interacting residues. In addition to the formation of precisely defined structures, Korendovych and co-workers (Zozulia et al., DOI: 10.1039/C8CS00080H) demonstrate that short peptides can self-assemble into structures that show significant catalytic activity in model reactions reaching efficiencies comparable to those found in natural enzymes by weight. They discuss different strategies used to rationally develop self-assembled peptide catalysts with natural and unnatural backbones as well as with metal-containing cofactors.
Peptide and protein materials beyond biology
Peptide and protein nanotechnology not only holds tremendous promise for the mimicry of components of living matter, by combining peptides with non-biological components, it is possible to achieve materials that go beyond biological applications.
One exciting application of peptide and protein nanotechnology is in the construction of charge or energy transporting wires that may find uses in energy harvesting and in interfacing electronics and biology. Tovar's group (Panda et al., DOI: 10.1039/C7CS00817A) summarise recent examples of electron and energy transport as mediated through protein-based macromolecules. These attractive bioinspired architectures have enabled several important applications that draw on their functional electrical properties, ranging from field-effect transistors to piezoelectrics.
The future: life-like nanotechnology
Looking back to the Chem. Soc. Rev. issue in 2010,7 the challenges listed included playing catch-up with the DNA nanotechnology community. The community has made tremendous progress in producing nanoscale objects with precisely designed shapes, and organizing these in programmable ways to form nanoscopic and macroscopic materials with precisely tunable properties. The development of methods to design and characterize multi-component systems introduces functions not normally associated with living matter. Protein and peptide nanotechnology is perhaps the ultimate green technology, in that materials can ultimately be produced from sunlight and CO2 and are inherently biodegradable. We have reached the point where polypeptide nanotechnology is not only treading the footsteps of DNA nanotechnology and natural proteins, but is starting to take full advantage of the chemical versatility of its building blocks and is already introducing new features, unexplored by evolution, thereby transcending biology.
And finally…
We are proud of this themed issue, and we are extremely grateful to our contributors who have taken on this mammoth task with us and showed incredible dedication and enthusiasm to create what we believe to be a very special issue with outstanding breadth as well as depth. Our contributors are leading scientists from biomedicine, biology, chemistry, engineering, physics, materials science, and nanotechnology, and we believe that they have covered their respective areas with authority and clarity. In such a diverse field, we have without question missed some areas. Indeed, the special issue could easily have contained two or three times as many contributions and we do apologize if certain critical areas were missed. Nonetheless, we hope that this themed issue provides an overview of the state of this interdisciplinary field which moves with confidence into the 2020s, and that it will be used as a source of reference and inspiration for the growing community of peptide and protein nanotechnologists. We look forward to what the next ten years will bring!
References
- L. Regan and W. F. DeGrado, Characterization of a helical protein designed from first principles, Science, 1988, 241, 976–978 Search PubMed.
- B. Kuhlman, G. Dantas, G. C. Ireton, G. Varani, B. L. Stoddard and D. Baker, Design of a novel globular protein fold with atomic-level accuracy, Science, 2003, 302, 1364–1368 CrossRef PubMed.
- S. Zhang, T. Holmes, C. Lockshin and A. Rich, Proc. Natl. Acad. Sci. U. S. A., 1993, 90, 3334–3338 CrossRef.
- W. A. Petka, J. L. Harden, K. P. McGrath, D. Wirtz and D. A. Tirrell, Reversible hydrogels from self-assembling artificial proteins, Science, 1998, 281, 389–392 CrossRef PubMed.
- M. R. Ghadiri, J. R. Granja, R. A. Milligan, D. E. McRee and N. Khazanovich, Self-assembling organic nanotubes based on a cyclic peptide architecture, Nature, 1993, 366, 324–327 CrossRef PubMed.
- Huang,
et al., The coming of age of de novo protein design, Nature, 2016 DOI:10.1038/nature19946.
- R. V. Ulijn and D. N. Woolfson, Peptide and Protein Based Materials in 2010: From Design and Structure to Function and Application, Chem. Soc. Rev., 2010, 39, 3349–3350 RSC.
|
This journal is © The Royal Society of Chemistry 2018 |