DOI:
10.1039/C7AY02249B
(Paper)
Anal. Methods, 2018,
10, 123-130
Validation of a lateral flow immunoassay for the rapid determination of aflatoxins in maize by solvent free extraction
Received
19th September 2017
, Accepted 26th November 2017
First published on 27th November 2017
Abstract
The analytical performances of a lateral flow immunoassay (AFLA-V AQUA™, Vicam a Waters Business) for the determination of aflatoxins in maize were evaluated according to Commission Regulation (EU) No. 519/2014. This case study showed how a critical evaluation of validation results can provide a quite informative picture of kit performances for its use for different purposes. The validation study, performed at aflatoxin B1 levels encompassing EU maximum permitted levels (up to 8 μg kg−1), provided information on the method precision profile, cut off values, and false suspect and false negative rates. The total precision expressed as relative standard deviation varied from 14 to 29% for contaminated samples. Cut off values calculated considering 2 μg kg−1 or 4 μg kg−1 as the screening target concentration were 1.24 and 2.18 μg kg−1, respectively, whereas the resultant false suspect rate for blanks was 42% and 8%, respectively. The false negative rate for samples containing the analyte at higher concentrations was found to be lower than 1%. Analysis of naturally contaminated maize samples performed by the lateral flow immunoassay and the AOAC official method revealed a good correlation (parameters of the linear regression: r = 0.97, slope = 0.96) between the obtained results. A satisfactory agreement between reference values and test results was also obtained in the analysis of reference materials. Finally, a QC protocol based on guidelines suggested in the EU regulation was designed to verify kit performances over time and in new production lots.
Introduction
Aflatoxins are secondary toxic metabolites occurring in a wide range of crops, including major staple cereals (e.g. maize), edible nuts, spices, dried fruits, and relevant derived products. The main fungal producers of aflatoxins are Aspergillus flavus, which produces aflatoxins B1 and B2 (AFB1 and AFB2), and Aspergillus parasiticus, which produces all four aflatoxin main forms, namely AFB1, AFB2, and aflatoxins G1 and G2 (AFG1 and AFG2). Aflatoxin contamination can occur before and/or after harvest. Recent overviews of aflatoxin global occurrence have been published by Rodrigues et al.,1 and Schatzmayr and Streit.2 In general, among the four major aflatoxins, AFB1 occurs at the highest levels and is the most toxic. Aflatoxins are a cause of human liver cancer and, in high doses, have caused deaths from aflatoxicosis.3,4
To protect consumers for the risk of exposure to aflatoxins by ingestion of contaminated foods, maximum permitted levels have been established by regulatory bodies in many countries. With respect to maize intended for human consumption, in the United States, an action level of 20 μg kg−1 of aflatoxins has been set by the US Food and Drug Administration,5 whereas within the European Union, maximum permitted levels of 2 μg kg−1 AFB1 and 4 μg kg−1 total aflatoxins are in force.6 Enforcement of risk-based food laws not only safeguards public health but also impacts economic viability, and drives the development and sustained use of risk monitoring and intervention technologies.
Notwithstanding a strict and detailed regulation for aflatoxins worldwide, a lack of data on exposure to aflatoxins mainly outside developed countries has been highlighted during the last meeting of the International Agency for Research on Cancer (IARC) Working Group (WG) in 2014.3 In its report the WG underlined that the problem of aflatoxin exposure is most acute in developing countries, also because of the lack of resources and analytical capacity for analyses. Consequently, few data are reported from these countries and those available are usually based on only a limited number of samples of uncertain quality. To cope with this situation, it is of utmost importance to have available reliable sampling and analytical tools that are fit for specific purposes, including rapid, inexpensive, and user friendly screening methods for use at the field/farm level. This could additionally help to support the already existing rapid alert system in the EU to immediately spread information and to speed up appropriate actions for food safety.
In addition to safety issues, from the market perspective, strict regulations result in the need for testing for mycotoxins at many points in the food chain. As a consequence, besides limited yields, restricted storage options, and restricted end markets, food and feed producers are also affected by the cost of testing raw materials and relevant processed products.
Based on the above considerations, it is easily understandable that screening tests can play an important role in the safety monitoring of food and feed chains, allowing rapid decision making and interventions, also affecting the final price of food products. Screening tests based on lateral flow devices (LFDs) represent one of the most common formats in the market, and their applications in food safety has consistently increased over the last 10 years,7–12 starting from rapid tests for antibiotic detection in dairy products and then spreading rapidly to the detection of other food contaminants such as pesticides and mycotoxins.12
The use of LFDs in combination with water-based extraction protocols, when available, represents a further advantage of these kits, eliminating the use of hazardous solvents, thus making them more practical for assays to be performed outside the laboratory. Indeed, organic solvent-free extraction protocols result in safer and cheaper analysis, eliminating costs associated with organic solvent management (safety measures for storage and disposal). With respect to aflatoxins, the proof of concept of their possible extraction with an aqueous-based medium has been reported for maize,13 plant oil,14 and soy-based foods.15 Further prominent research fields aimed at improving the analytical performances of LDFs, such as the use of alternative labels and alternative receptors, and the development of multiparametric assays have been reviewed by Dzantiev et al.12 and Anfossi et al.8 While using alternative receptors and labels can improve the sensitivity of the test, the development of multi-mycotoxin assays can have a great impact on the characterization of the risk of exposure. For instance, the risk of co-exposure to aflatoxins and fumonisins in developing countries due to the co-occurrence of these mycotoxins has been widely discussed in the last IARC report.3 In such a case, the availability of cost-effective and high throughput multi-mycotoxin rapid methods would enhance the ability to collect co-occurrence data to better understand the risk of co-exposure of humans and animals.
On the other hand, industrial and commercial developments of LFDs claim for validated protocols of analysis to comply with performance criteria set in official guidelines. At the EU level, validation guidelines for mycotoxin screening methods are specified in the recently issued Commission Regulation (EU) No. 519/2014.16,17 According to this regulation, commercial test kits of which the result of the measurement is a numerical value – and not exclusively an indication of the presence/absence of the analyte – should include an applicability statement containing information on the target matrix, mycotoxin concentration, cut off values, and false suspect rate. All this information shall be obtained by conducting a validation study. It is worth pointing out that performing validation studies is not only essential for official control purposes, but more and more a stakeholder request to better understand the performances and limitations of commercial kits before applying them for specific purposes. Examples of validation studies to evaluate the performances of commercial lateral flow test kits for aflatoxins according to the AOAC Research Institute Performance Tested Methods™ program and guidelines set in the Commission Decision 2002/657/EC can be found in the literature.18–20 Even though based on commercial products, these validation studies are highly informative on the general performances of immunochromatographic assays.
The aim of this work was to evaluate the analytical performances of a commercial LFD (AFLA-V AQUA™, Vicam a Waters Business) for the determination of aflatoxins in maize after water-based extraction, according to Commission Regulation (EU) No. 519/2014. The validation study was performed at aflatoxin levels encompassing EU maximum permitted levels, and provided information on the method precision profile, cut off values, and false suspect and false negative rates. The trueness of generated data was assessed either by analysis of reference materials, or by comparison with the official AOAC reference method in the analysis of naturally contaminated maize samples. In addition, a quality control (QC) protocol based on guidelines suggested in the EU regulation was designed to verify kit performances over time and in new production lots.
Experimental
Reagents
Methanol (HPLC grade) was purchased from Mallinckrodt Baker (Milan, Italy). Ultrapure water was produced by using a Millipore Milli-Q system (Millipore, Bedford, MA, USA). Sodium chloride and ammonium acetate (for mass spectrometry) were from Sigma-Aldrich (Milan, Italy). AflaTest WB immunoaffinity columns were from VICAM (A Waters Business, Milford, MA, USA). Paper filters (Whatman 4) and glass microfiber filters (Whatman GF/A) were obtained from Whatman International Ltd (Maidstone, UK). Standard aflatoxins (AFB1, AFB2, AFG1, AFG2 and U-[13C17]-AFB1) were purchased from Romer Labs (Getzersdorf, Austria).
Maize samples
Blank and naturally contaminated maize samples were collected from local producers in Italy in the period March–July 2016. All samples were finely ground with a Tecator Cyclotec 1093 (International PBI, Milan, Italy) laboratory mill equipped with a 500 μm sieve. The following reference materials were also used for this study: batch no. AC-285 (AFB1 reference value 5.9 μg kg−1), batch no. AC-274 (AFB1 reference value 7.3 μg kg−1), batch no. AC-276 (AFB1 reference value 1.7 μg kg−1), and batch no. A-C-2204 (AFB1 reference value 301.1 μg kg−1) from Trilogy Analytical Laboratory, Inc. (Washington, USA); batch no. BRM003027 (AFB1 reference value 4.52 μg kg−1) from Romer Labs (Getzersdorf, Austria).
Test and equipment
The strip test (AFLA-V AQUA™), AQUA Premix solution (extraction buffer), incubator, and reader (Vertu Reader) were from VICAM (A Waters Business, Milford, MA, USA). Newly produced kit lots were used within this study. According to provider instructions, the kits were stored at 4 °C until use. Under these conditions kits were stable over a period of 12 months. Kit lot 035-006ECQ.1 was used for validation experiments, whereas kit lots 035-009ECQ.0, 035-012ECQ.0, 035-013ECQ.1, 035-014ECQ.2, 035-015ECQ.1, and 0132016ECQ.1 were used for method verification by the QC protocol.
The strip test format is based on an indirect competitive immunoassay. Line intensities developed on the strip membrane (test line and control line) are measured using the photometric reader. The test response is the ratio between the signal intensity of the test line and that of the control line and it is converted into aflatoxin concentration through a lot specific calibration curve.
Samples for LFD analysis were prepared as follows. Five grams of ground maize were extracted with 25 ml of AQUA Premix solution by magnetic stirring for 3 min. The sample extract was filtered through a paper filter. Then, 100 μl of filtered extract were slowly pipetted onto the strip (about one drop per second) and allowed to run for 5 min. After running, the lateral flow was immediately placed into the reader holder. The lot specific calibration curve was uploaded onto the reader system by using the corresponding barcode provided by the supplier. The calibration curve was generated by spiking uncontaminated maize at seven AFB1 levels over the range 0–100 μg kg−1, performing triplicate measurements for each calibration level.
Confirmatory analysis (AOAC 991.31)
To calculate the reference value of the aflatoxin concentration in test materials used for validation and in naturally contaminated samples used for method comparison purposes, samples were extracted and purified according to the AOAC Official Method 991.31. Subsequently, aflatoxins were determined by liquid chromatography coupled to tandem mass spectroscopy (LC-MS/MS). Briefly, 25 g of sample, added with 5 g NaCl, were extracted with 125 ml of methanol/water (70
:
30). Then 15 ml of filtered extract were diluted with 30 ml water. Fifteen ml of diluted extract (equivalent to 1 g test portion) were passed through the immunoaffinity column. After column washing with 2 × 10 ml water, aflatoxins were eluted with 1 ml methanol. The eluate was spiked with an appropriate amount of U-[13C17]-AFB1 and dried at 50 °C under a stream of air. The residue was re-dissolved in 200 μl of methanol/water 80
:
20.
LC-MS/MS analysis was performed with a QTrap MS/MS system from Applied Biosystems (Foster City, CA, USA), equipped with an electrospray (ESI) interface and a 1100 series micro-LC system (Agilent Technologies, Waldbronn, Germany). The analytical column was a Gemini® C18 column (150 mm × 2 mm, 5 μm particles, Phenomenex, Torrance, CA, USA), the column oven was set at 40 °C, the flow rate of the mobile phase was 200 μl min−1 and the injection volume was 20 μl. The mass spectrometer was operated in SRM (selected reaction monitoring) mode by monitoring 2 transitions (1 quantifier and 1 qualifier) for each compound, in detail: 313–241 and 313–213 for AFB1; 315–287 and 315–259 for AFB2; 329–311 and 329–243 for AFG1; 331–313 and 331–245 for AFG2; 330–255 and 330–245 for U-[13C17]-AFB1. The quantification limit was 0.5 μg kg−1 for AFB1, AFB2, AFG1, and AFG2 individually.
Validation
Preparation of test materials.
For the validation experiments three sets of maize samples were prepared: (a) blank samples, (b) samples contaminated with AFB1 at about 2 μg kg−1, (c) samples contaminated with AFB1 at about 4 μg kg−1. To take the effect of matrix-to-matrix variation into account in precision estimation, sample sets were prepared using three maize batches having different origins. To obtain the desired AFB1 levels, blank maize was mixed with highly contaminated maize. In particular, a certified reference material containing AFB1: 267.4 μg kg−1, AFB2: 27.1 μg kg−1, AFG1: 6.2 μg kg−1, and AFG2: 0.3 μg kg−1 (Trilogy batch no. A-C-2204) was used. After mixing and homogenization in a laboratory mixer, aflatoxin levels in each material were characterized by LC-MS/MS analysis, according to the above described procedure. In addition, two maize samples naturally contaminated with 5.8 ± 0.4 and 7.7 ± 1.3 μg kg−1 AFB1, respectively, were used for the evaluation of false negative results.
Validation design.
The main purpose of the method is to classify samples into two classes, namely compliant and suspect non-compliant. Therefore the format of the method is qualitative in this respect, since it delivers a binary result for a specific sample. Qualitative methods can be validated according to the corresponding AOAC guidelines21 applying the concept of probability of detection (POD) introduced by Wehling et al.22 One of the major drawbacks of this approach however is the need for a very high number of replicate analyses to obtain a reliable estimate of the rate of false positive and false negative results of the method. Moreover, the exclusive use of binary information does not allow for establishing a cut off value. Therefore the quantitative profile of the method was utilised in this study to evaluate the performance of the method when used for screening purposes. In consequence, the validation experiments were designed to fulfil the corresponding specifications in Regulation 519/2014/EU in terms of minimum sample set, and minimum number of validation levels and days. For each validation level, measurements were spread over 5 different days, using three different maize batches. In addition, two of the maize batches were analysed in duplicate on each day under repeatability conditions, resulting in five independent and complete analyses per day and 25 measurements in total per each tested AFB1 concentration. This nested design was repeated separately for three AFB1 levels, namely negative (blank) samples and samples containing AFB1 at 2 and 4 μg kg−1 (see the Preparation of test materials). The results of analysis expressed in terms of μg kg−1 aflatoxins returned by the reader were then subjected to statistical assessment to calculate validation parameters as described in the following. To evaluate the range of applicability of the method, the cut off values and false suspect rate were calculated either by considering 2 or 4 μg kg−1 as the screening target concentration (STC).
To evaluate the precision profile of the method, data generated by the nested design, performed for each validation level, were subjected to analysis of variance (ANOVA) as specified by ISO 5725.23 According to this model each of the measurements of the response Yijk is defined as the true value (TV) plus the contribution of 3 components:
| Yijk = TV + Di + Mij + Rijk | (1) |
where
Di is the between-day variability,
Mij is the between-matrix (maize batches from different origins in the present case) variability, and
Rijk is the within-day variability. The within-day variability gives the precision under repeatability conditions, whereas the sum of all components (including the between-day and between-matrix variability) gives the intermediate precision. The statistical assessment was done with the software package MINITAB™ Statistical Software for Windows (Version 15).
The measured levels (μg kg−1) of samples containing AFB1 at the STC were taken as basis for the calculation of the cut off value. According to Regulation 519/2014/EU the following equation was used:
| Cut off value = RSTC − t-value(0.05) × SDSTC | (2) |
where
RSTC is the mean level of AFB
1 (μg kg
−1) calculated from all 25 experiments performed on samples containing AFB
1 at the STC, SD
STC is the corresponding standard deviation of intermediate precision as defined in the previous paragraph, and
t-value
(0.05) is the one tailed
t-value for a rate of false negative results of 5%.
Using the results from the analysis of negative samples the rate of false suspect results was estimated by first calculating the t-value as follows:
| 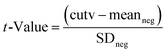 | (3) |
where mean
neg is the mean value of the results obtained from the 25 experiments on the negative samples, cutv is the cut off value established as above, and SD
neg is the corresponding standard deviation of intermediate precision.
From the obtained t-value, based on the degrees of freedom calculated from the number of experiments (24 in the present case), the false suspect rate results (probability) for a one tailed distribution was calculated using the spread sheet function “TDIST” in Microsoft Excel.
The false suspect rate for samples containing AFB1 at 50% STC was calculated by applying the same procedure using the mean value of the results obtained from the 25 experiments on samples containing AFB1 at 50% STC and the relevant standard deviation of intermediate precision.
Finally, the false negative rate for samples containing AFB1 at levels above the STC was estimated by calculating the t-value as specified below
| 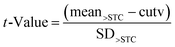 | (4) |
where mean
>STC is the mean value of the results obtained from the experiments on the samples containing the analyte above the STC, cutv is the cut off value established as above, and SD
>STC is the corresponding standard deviation of the intermediate precision. The probability corresponding to the calculated
t-value with a one-tailed distribution gives the rate of false negative results for the samples containing the analyte at levels higher than the STC.
Results and discussion
Preparation of test materials
The validation of a screening method is accomplished by estimating a set of parameters that can be calculated using the method response obtained for at least one positive (at the STC) and one negative sample set. Additional sample sets can be analyzed to evaluate the method performance at other levels of interest. In this study each sample set included 25 samples, thus fulfilling the requirement of a minimum sample number of 20, belonging to three maize batches having different origins. Negative samples contained AFB1 at levels lower than 0.5 μg kg−1 (i.e. the LOQ of the LC-MS/MS method), whereas two positive sample sets were prepared containing AFB1 at about 2 and 4 μg kg−1, respectively. To reach the desired AFB1 levels, test materials were prepared by blending blank and highly contaminated samples. Batches of 500 g were prepared and homogenized for each test material, and aflatoxin levels were characterized by LC-MS/MS analysis taking 3 subsamples from each batch. The mean value of the aflatoxin levels of the three subsamples is reported in Table 1. The results of the analyses showed that for the target screening concentrations of 2 and 4 μg kg−1 the reference values of the samples differed slightly from these levels. To obtain an unbiased estimate of the cut off values, the concentrations obtained with the LFD analysing the samples at the screening target concentrations were corrected considering the corresponding reference values. The correction was comprised of the following two steps. First, sample specific recovery rates for the concentration obtained with the LFD were calculated separately for each sample using the corresponding reference values. In the second step these recovery rates were then applied for the target screening concentrations of 2 and 4 μg kg−1, respectively. These corrected concentrations were then subjected to statistical assessment to estimate the cut off values for the two different screening target concentrations.
Table 1 Aflatoxin B1 levels in test materials used for validation experiments. Values in the table are the average concentration of three independent determinations by LC-MS/MS ± the uncertainty calculated as standard error of the mean
Sample |
AFB1a (μg kg−1) |
Group A (negative) |
Group B (2 μg kg−1) |
Group C (4 μg kg−1) |
AFB2, AFG1, and AFG2 concentrations were <0.5 μg kg−1 in each material.
|
Maize batch 1 |
<0.5 |
2.3 ± 0.4 |
3.4 ± 0.1 |
Maize batch 2 |
<0.5 |
2.7 ± 0.4 |
4.4 ± 0.6 |
Maize batch 3 |
<0.5 |
2.4 ± 0.01 |
3.8 ± 0.7 |
Precision, cut off values, and false suspect and false negative rates
The validation experiments were conducted according to a five day nested design as described in the Experimental section. Screening target concentration values of 2 and 4 μg kg−1 were set taking into account regulatory requirements and levels of interest to customers for process management purposes. The test results obtained from the 25 measurements performed for each validation level were taken as basis for the calculation of validation parameters. The overall results of the statistical assessment are shown in Table 2.
Table 2 Performances of the lateral flow immunoassay for AFB1 determination in maize. Statistical analysis to evaluate the range of applicability of the method and relevant performances, cut off values and the false positive rate were calculated either by considering 2 μg kg−1 or 4 μg kg−1 as the Screening Target Concentration (STC)
|
|
STC 2 μg kg−1 |
STC 4 μg kg−1 |
The mean values of the response (μg kg−1) are calculated from the results of 25 experiments.
RSDip (%) is the total relative standard deviation of the measurements (intermediate precision).
|
Blank
|
Mean response (μg kg−1)a |
1.09
|
|
|
RSDip (%)b |
69.8
|
|
|
Source of errors (% of total variance)
|
Day |
0 |
|
|
Maize variety |
97 |
|
|
Repeatability |
3 |
|
|
Rate of false suspect% |
|
42 |
8 |
![[thin space (1/6-em)]](https://www.rsc.org/images/entities/char_2009.gif) |
2 μg kg
−1
|
Mean response (μg kg−1)a |
1.8
|
|
|
RSDip (%)b |
18.4
|
|
|
Source of errors (% of total variance)
|
Day |
0 |
|
|
Maize variety |
62 |
|
|
Repeatability |
38 |
|
|
Rate of false suspect% |
|
|
14 |
![[thin space (1/6-em)]](https://www.rsc.org/images/entities/char_2009.gif) |
4 μg kg
−1
|
Mean response (μg kg−1)a |
4.3
|
|
|
RSDip (%)b |
28.7
|
|
|
Source of errors (% of total variance)
|
Day |
0 |
|
|
Maize variety |
44 |
|
|
Repeatability |
56 |
|
|
Rate of false negative% |
|
1 |
|
Cut off (μg kg
−1
)
|
1.24
|
2.18
|
First, precision data were calculated for all three tested concentrations. For contaminated samples, the intermediate precision expressed as relative standard deviation was 18 and 29% for 2 and 4 μg kg−1, respectively. With respect to the blank samples, a high relative standard deviation of the test response was observed. However, in the following it will be shown that notwithstanding this high value, an acceptable rate of false positive results for the blank samples was obtained when considering 4 μg kg−1 as the STC. In addition, analysis of variance (ANOVA) gave information on the error contribution due to the considered sources of variability (day-to-day, matrix effect and repeatability) (Table 2). In all examined cases, day-to-day variability had no impact on the total variance, which resulted to be mainly affected by repeatability and matrix-to-matrix variation. The contribution of the matrix-to-matrix variation was not surprising when considering that the extract was applied to the LFD without any dilution. On the other hand, in previous studies reporting on the validation of LFDs for deoxynivalenol analysis in wheat, the contribution of a matrix effect was found to be definitely negligible,17 probably because this method included extract dilution. This would suggest the need for checking kit performances before applying the method to unusual or new maize varieties. However the overall precision <30% indicated an acceptable robustness of the method, also taking into consideration the low target levels of AFB1 considered for validation.
Once intermediate precision data were available, it was possible to calculate the cut off values. This value is defined as the response, signal, or concentration obtained with the screening method, above which the sample is classified as “suspect”, with a false negative rate of 5%. In terms of statistical terminology, the false classification of non-compliant samples as compliant corresponds to the β error, a key performance criterion for screening methods already specified in the Commission Decision 657/2002/EC. In the present study, to evaluate the range of applicability of the method, the cut off value and false suspect rate were calculated either by considering 2 μg kg−1 or 4 μg kg−1 as the STC. Taking into account the mean responses and relevant RSDip for samples contaminated at the STC, the calculated cut off values were 1.24 μg kg−1 and 2.18 μg kg−1 considering 2 or 4 μg kg−1 as the STC respectively (Table 2). In both cases the assay sensitivity was considered satisfactory for assessing maize contamination at the two target levels. Fig. 1 shows a graphical presentation of the results for the measurements from the blank maize samples and samples containing AFB1 at 2 and 4 μg kg−1. A clear separation of the results from the responses obtained from samples fortified at the different tested levels was observed. Furthermore, 24 out of the 25 results from the blank samples were below the calculated cut off value of 2.18 μg kg−1.
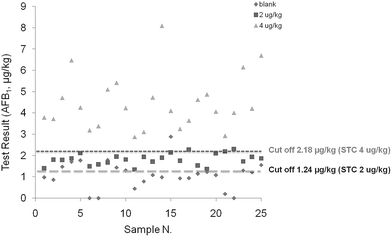 |
| Fig. 1 Test results (μg kg−1) for the AFB1 measurements from the blank maize samples and samples contaminated with 2 and 4 μg kg−1. | |
Based on the cut off values, the rate of false positive results was estimated for samples containing mycotoxins below the target levels (Table 2). If considering 2 μg kg−1 as the STC a false suspect rate of 42% was obtained for the blank samples, whereas if considering 4 μg kg−1 as the STC the false suspect rate was 8% for the blank samples and 14% for the samples contaminated at 2 μg kg−1 (in this case equivalent to 50% STC). The false negative rate for samples containing AFB1 above the STC was estimated using two maize samples contaminated with AFB1 at 5.8 and 7.7 μg kg−1. Each sample was analyzed twice (replicated independent analysis) for 5 days, for a total of 10 measurements. False negative rates, calculated as described in the Experimental section, was found to be lower than 1% in all considered cases (Table 3).
Table 3 False negative results of the lateral flow immunoassay for aflatoxin determination in maize, calculated for two maize materials contaminated with AFB1 at 5.8 and 7.7 μg kg−1, respectively. Statistical evaluations were done either by considering 2 μg kg−1 (the EU maximum permitted level for maize) or 4 μg kg−1 as the Screening Target Concentration (STC)
Reference value (μg kg−1) |
|
|
STC 2 μg kg−1 |
STC 4 μg kg−1 |
The mean values of the response (μg kg−1) are calculated from the results of 10 experiments (two independent replicates for 5 days).
RSDip (%) is the total relative standard deviation of the measurements (intermediate precision).
|
5.8 ± 0.4 |
Mean response (μg kg−1)a |
7.9
|
|
|
RSDip (%)b |
14.0
|
|
|
Rate of false negative% |
|
0.01 |
0.03 |
7.7 ± 1.3 |
Mean response (μg kg−1)a |
8.9
|
|
|
RSDip (%)b |
21.3
|
|
|
Rate of false negative% |
|
0.14 |
0.06 |
The overall results indicated satisfactory kit reliability in discriminating samples contaminated at different AFB1 levels set in a very narrow working range (from n.d. to 8 μg kg−1) as required by EU regulatory limits. As an example, setting a STC of 4 μg kg−1 could be suitable when screening aflatoxin contamination of maize to be subjected for sorting or physical treatments before human consumption, for which a maximum permitted level 5 μg kg−1 AFB1 is set in EU.
With respect to performances at the STC of 2 μg kg−1, in spite of having an adequate cut off value, the screening method showed a high rate of false suspect results (42%) for the blanks. However, to establish if these performances are fit-for-purpose or not, considerations should also be based on the fact that the risk of false positives is not a safety issue, but an economical one. A high false positive rate may affect the economic benefit of the test, because false suspect results require the application of confirmatory methods to samples that are actually compliant with legal or target levels. The lateral flow test being less expensive and organic solvent free, and requiring less time and skills, the overall balance of the pros (costs and time) and cons (42% false positive) could be still positive.
Analysis of naturally contaminated samples and certified reference materials
The trueness of data generated by the validated LFD was assessed either by analysis of naturally contaminated samples and comparison with the AOAC 991.31 reference method, or by analysis of certified reference materials. For comparison purposes a set of 48 naturally contaminated maize samples was analyzed. AFB1 concentration ranged from n.d. to 36 μg kg−1, whereas AFB2, AFG1, and AFG2 concentrations were lower than 0.5 μg kg−1 (the LC-MS/MS LOQ) in all samples. In Fig. 2 the results of the analysis performed by the LFD and the AOAC Official Method 991.31 are shown, and the obtained correlation (parameters of the linear regression: r = 0.97, slope = 0.96) was considered acceptable taking into account the intrinsic variation of results obtained with screening methods. The kit performance was established exclusively based on results for AFB1 in the investigated concentration range (n.d. to 36 μg kg−1) since LC-MS/MS data showed that within this range, the contribution of the other regulated aflatoxins (AFB2, AFG1, and AFG2) to the total AF contamination was negligible. Finally, the reference values were also compared with the mean values of LFD test results for a set of four 4 maize reference materials containing aflatoxins in the range 1.7–7.3 μg kg−1 (Table 4). The results indicated that there was a slight bias of the LFD method towards higher values compared to the reference method, but the differences were considered acceptable for a screening method.
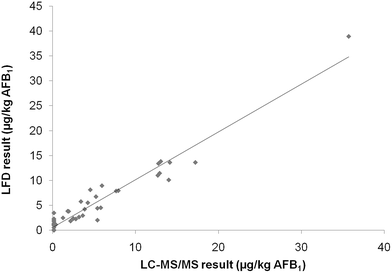 |
| Fig. 2 Correlation between results (AFB1 concentration, μg kg−1) obtained by the lateral flow immunoassay and a confirmatory method based on the AOAC Official Method 991.31 for sample preparation and LC-MS/MS detection. | |
Table 4 Analysis of reference materials: comparison between reference values and AFB1 concentrations measured with the validated LFD
|
Reference value (μg kg−1) |
Test resulta (μg kg−1) |
Test results are mean values ± standard error of the mean calculated for 6 independent measurements performed on 3 different days.
|
Trilogy A-C-285 |
5.9 ± 1.2 |
5.8 ± 0.2 |
Trilogy A-C-274 |
7.3 ± 0.9 |
9.3 ± 0.4 |
Trilogy A-C-276 |
1.7 ± 0.3 |
2.3 ± 0.4 |
Biopure BRM003027 |
4.52 ± 1.20 |
4.9 ± 0.2 |
Validation follow up – QC procedure
To verify kit performances over time and in new production lots, a QC protocol based on guidelines suggested in the Commission Regulation (EU) No. 519/2014 was designed. For QC purposes a minimum of six negative control and six positive control samples shall be analyzed. To consider validation performances confirmed at the QC stage, the positive control samples shall all be classified by the method above the cut off value determined during validation. QC results for different LDF kit lots produced over the year 2016 are reported in Table 5. For QC purposes three test materials were selected from the validation set (Table 1), including one negative material and two contaminated materials having AFB1 concentration close to the two STC values considered in this study (i.e. 2 and 4 μg kg−1). The results in Table 5 are the average and standard deviation of six independent measurements for each test material. Data (mean test response ± standard deviation) were evaluated against the two cut off values determined in this study, i.e. 1.24 μg kg−1 (considering 2 μg kg−1 as the STC) and 2.18 μg kg−1 (considering 4 μg kg−1 as the STC). If considering the cut off value of 1.24 μg kg−1, all lots but two (no. 3 and 5) were able to correctly classify as negative the blank material, whereas with respect to the cut off value of 2.18 μg kg−1 also values given by lot no. 3 and 5 would be correctly classified as negative. All lots were able to correctly classify as suspect the contaminated materials. The overall data collected by QC measurements confirmed lot-to-lot consistency of analytical performances obtained at the validation stage.
Table 5 QC results for different LFD kit lots produced over the year 2016. Results are the average and standard deviation of six independent measurements for each test material. Data were evaluated against the two cut off values determined by validation experiments, i.e. 1.24 μg kg−1 (considering 2 μg kg−1 as the STC) and 2.18 μg kg−1 (considering 4 μg kg−1 as the STC)
Kit lot |
STC 2 μg kg−1 − cut off 1.24 μg kg−1 |
Ref. value: blank |
Ref. value: 2.4 ± 0.6 |
Measured value |
Measured value |
035-009ECQ.0 |
0.8 ± 0.4 |
1.7 ± 0.2 |
035-012ECQ.0 |
0.0 ± 0.0 |
1.5 ± 0.3 |
035-013ECQ.1 |
1.8 ± 0.4 |
2.7 ± 0.1 |
035-014ECQ.2 |
0.6 ± 0.6 |
2.2 ± 0.1 |
035-015ECQ.1 |
1.3 ± 0.3 |
1.8 ± 0.4 |
0132016ECQ.1 |
0.5 ± 0.5 |
1.8 ± 0.2 |
Kit lot |
STC 4 μg kg−1 − cut off 2.18 μg kg−1 |
Ref. value: blank |
Ref. value: 3.6 ± 0.7 |
035-009ECQ.0 |
0.8 ± 0.4 |
3.2 ± 0.9 |
035-012ECQ.0 |
0.0 ± 0.0 |
2.2 ± 0.5 |
035-013ECQ.1 |
1.8 ± 0.4 |
3.4 ± 0.2 |
035-014ECQ.2 |
0.6 ± 0.6 |
5.1 ± 0.1 |
035-015ECQ.1 |
1.3 ± 0.3 |
4.4 ± 0.2 |
0132016ECQ.1 |
0.5 ± 0.5 |
4.4 ± 0.5 |
Conclusions
The analytical performances and fitness for purpose of a commercial test kit for the determination of aflatoxins in maize at EU maximum permitted levels have been evaluated, according to guidelines set in Regulation 519/2014/EU. The total precision for contaminated samples was lower than 29%. Cut off values calculated considering 2 μg kg−1 or 4 μg kg−1 as the STC were 1.24 and 2.18 μg kg−1, respectively, whereas the false suspect rate for blanks was 42% and 8%, respectively. The false negative rate for samples containing the target analytes above the screening target concentrations was found to be <1% in all statistical evaluation, thus complying with the criterion of maximum 5% set in Commission Decision 657/2002/EC and Regulation 519/2014/EU. Analysis of naturally contaminated maize samples performed by using a LFD and the AOAC official method revealed a good correlation (parameters of the linear regression: r = 0.97, slope = 0.96) between the obtained results. Furthermore a satisfactory agreement between reference values and test results was also obtained in the analysis of reference materials. The overall results of validation experiments gave a quite informative picture of kit performances for its use for different purposes, such as robustness among different maize varieties, acceptable performances for compliance testing at EU maximum permitted levels, satisfactory accuracy and precision in discriminating different contamination levels in the narrow concentration of interest for AFB1 (in the low μg kg−1 range). Finally, a QC protocol based on guidelines suggested in the EU regulation was designed to verify kit performances over time and in new production lots. The same protocol shall be applied when implementing the method in any new laboratory.
Conflicts of interest
There are no conflicts to declare.
References
- I. Rodrigues, J. Handl and E. M. Binder, Food Addit. Contam., Part B, 2011, 4(3), 168 CrossRef CAS PubMed.
- G. Schatzmayr and E. Streit, World Mycotoxin J., 2013, 6(3), 213 CrossRef CAS.
-
IARC, Working Group Report No. 9. Mycotoxin Control in Low- and Middle-income Countries, ed. C. P. Wild, J. D. Miller and J. D. Groopman, 2016, available from: http://www.iarc.fr/en/publications/pdfs-online/wrk/wrk9/index.php, accessed September 2017 Search PubMed.
-
WHO-FAO, WHO Technical Report Series 947. Evaluation of certain food additives and contaminants, Sixty-eighth Report of the Joint FAO/WHO Expert Committee on Food Additives, 2017, available from: http://apps.who.int/iris/bitstream/10665/43870/1/9789241209472_eng.pdf, accessed September 2017 Search PubMed.
-
Food and Drugs Administration (FDA), Guidance for Industry: Action Levels for Poisonous or Deleterious Substances in Human Food and Animal Feed, 2000, available from: http://www.fda.gov/food/guidanceregulation/ucm077969.htm, accessed September 2017 Search PubMed.
- European Commission, Commission Regulation (EU) No. 165/2010 of 26 February 2010 amending Regulation (EC) No. 1881/2006 setting maximum levels for certain contaminants in foodstuffs as regards aflatoxins, Official Journal of the European Union, 2010, L50, 8–12 Search PubMed.
- W. Li, S. Powers and S. Y. Dai, World Mycotoxin J., 2014, 7(4), 417 CrossRef CAS.
- L. Anfossi, C. Baggiani, C. Giovannoli, G. D'Arco and G. Giraudi, Anal. Bioanal. Chem., 2013, 405, 467 CrossRef CAS PubMed.
- E. Anklam, J. Stroka and A. Boenke, Food Control, 2002, 13, 173 CrossRef CAS.
- R. Krska and A. Molinelli, Anal. Bioanal. Chem., 2009, 393, 67 CrossRef CAS PubMed.
- C. M. Maragos and M. Busman, Food Addit. Contam., Part A, 2010, 27(5), 688 CrossRef CAS PubMed.
- B. B. Dzantiev, N. A. Byzova, A. E. Ursov and A. V. Zherdev, TrAC, Trends Anal. Chem., 2014, 55, 81 CrossRef CAS.
- L. Anfossi, G. D'Arco, M. Calderara, C. Baggiani, C. Giovannoliand and G. Giraudi, Food Addit. Contam., Part A, 2011, 28(2), 226 CrossRef CAS PubMed.
- S. Qing, Z. Zhu, D. Qian-min, L. Jie-min and S. Guo-qing, Anal. Methods, 2016, 8(3), 564 RSC.
- V. O. Santos, P. B. Pelegrini, F. Mulinari, A. F. Lacerda, R. S. Moura, L. P. V. Cardoso, S. Bührer-Sékula, R. N. G. Miller and M. F. Grossi-de-Sa, Anal. Methods, 2017, 9(18), 2715 RSC.
- European Commission, Commission Regulation (EU) No. 519/2014 of 16 May 2014 amending Regulation (EC) No. 401/2006 as regards methods of sampling of large lots, spices, and food supplements, performance criteria for T-2 and HT-2 toxin and citrinin and screening methods of analysis, Official Journal of the European Union, 2014, L147, 29–43 Search PubMed.
- V. M. T. Lattanzio, J. AOAC Int., 2016, 99(4), 906 CrossRef CAS PubMed.
- S. Polakowski, R. W. Roberts, K. Tanguay, C. Bailey, A. H. Davis and B. Gow, J. AOAC Int., 2015, 98(6), 1571 CrossRef CAS PubMed.
- B. E. Roman, D. Driksna, M. M. Abouzied, F. Klein and J. Rice, J. AOAC Int., 2017, 100(4), 1131 CrossRef CAS PubMed.
- W. Reybroeck, S. Ooghe, S. J. Saul and R. S. Salter, Food Addit. Contam., Part A, 2014, 31(12), 2080 CrossRef CAS PubMed.
-
AOAC, Guidelines for Validation of Qualitative Binary Chemistry Methods, 2013, http://www.aoac.org/aoac_prod_imis/AOAC_Docs/ISPAM/Qual_Chem_Guideline_Final_Approved_031412.pdf Search PubMed.
- P. Wehling, R. A. LaBudde, S. L. Brunelle and M. T. Nelson, J. AOAC Int., 2011, 94(1), 335 CAS.
-
ISO, Accuracy (Trueness and Precision) of Measurement Methods and
Results – Part 3: Intermediate measures of the Precision of a Standard Measurement Method, ISO 5725–3, ISO Standards, Geneva, 1994(E), ICS 03.120.30 Search PubMed.
|
This journal is © The Royal Society of Chemistry 2018 |