DOI:
10.1039/C7FO01295K
(Paper)
Food Funct., 2018,
9, 344-354
Capsaicin inhibits the metastasis of human papillary thyroid carcinoma BCPAP cells through the modulation of the TRPV1 channel
Received
21st August 2017
, Accepted 11th November 2017
First published on 13th November 2017
Abstract
Capsaicin (CAP), a potent transient receptor potential vanilloid type 1 (TRPV1) agonist, is a major ingredient of red pepper. Recently, capsaicin has attracted increasing attention owing to its multiple biological activities. However, the anticancer effects of capsaicin against various types of cancers, especially on thyroid carcinoma, have not been completely elucidated. TRPV1, which can be activated by capsaicin, plays a key role in many biological and physiological processes. In the present study, the anticancer properties of capsaicin against papillary thyroid cancer BCPAP cells were investigated. Our results indicated that TRPV1 and TRPV6 were universally expressed in different types of thyroid cell lines. Capsaicin could inhibit multiple steps of metastasis without affecting the viability of BCPAP cells. The activation of TRPV1 by capsaicin (25–100 μM) significantly suppressed the migration and invasion of BCPAP cells as well as their adhesion. The protein levels of Snail1 and Twist1, two critical EMT transcription factors (EMT-TFs), dramatically decreased in a dose-dependent manner after capsaicin treatment, accompanied by the up-regulation of downstream protein E-cadherin. Subsequently, the activation of TRPV1 by capsaicin also caused significant inhibition of the expression of MMP-2 and MMP-9. Moreover, the inhibitory effects of capsaicin on the metastasis of BCPAP cells were abrogated by the pre-treatment of a specific TRPV1 antagonist (capsazepin). Our results suggest that the activation of TRPV1 by capsaicin is associated with the metastatic inhibition of papillary thyroid cancer BCPAP cells, indicating that targeting of TRPV1 functions remains a feasible strategy for cancer treatment.
1. Introduction
Capsaicin ((E)-N-[(4-hydroxy-3-methoxyphenyl)methyl]-8-methylnon-6-enamide, the chemical structure is shown in Fig. 1A) is the main ingredient of red or hot chili pepper of the genus Capsicum, which is principally responsible for the pungency of chili pepper.1Capsicum annuum has been widely consumed for centuries as a spicy food seasoning to stimulate appetite. In some cases, it serves as a kind of herbal medicine to treat deficiency cold. Recently, capsaicin has attracted increasing attention because of its antioxidant,2 anti-inflammatory,3 anti-hyperlipidemic4 and analgesic properties.5 Besides these, it possesses anticancer properties against various types of cancers.6 However, there is very limited information about the effects of capsaicin on thyroid carcinoma so far.
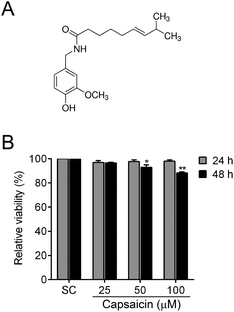 |
| Fig. 1 Effects of capsaicin on the cell viability of BCPAP cells. (A) Chemical structure of capsaicin. (B) Effect of capsaicin on the cell viability of BCPAP cells. BCPAP cells were treated with different dosages of capsaicin (25–100 μM) for 24 h or 48 h, and then the cell viability was determined by using the MTT assay. The data are represented as the mean ± SEM of three independent experiments, and statistical analysis was performed using an unpaired Student's t test. *P < 0.05 and **P < 0.01 vs. solvent control. | |
Thyroid cancer is one of the most common endocrine malignancies with its incidence stably increasing over the past few decades.7 Thyroid tumors mostly stem from thyroid follicular epithelial cells, which can be further subdivided into well-differentiated cancer, poorly differentiated cancer and anaplastic cancer according to their malignant behavior.8 Differentiated thyroid cancer is currently treated by thyroidectomy, subsequent radioactive iodine (RAI) therapy and long-term thyroid stimulating hormone suppression.9 Although a majority of thyroid cancers have a favorable prognosis, there are scarcely any effective therapeutic approaches for metastatic thyroid cancers. Traditional treatment strategies are usually not effective for those patients with nasty local invasion or distant metastasis,10 and patients harboring distant metastasis frequently deteriorate, with a nearly 50% 5-year survival rate.11 In addition, if dedifferentiation occurs, thyroid cancer patients will assume more risks of neoplasm metastasis and lose RAI avidity which is always related to a poor prognosis.12 Hence, it is urgent to seek a novel strategy to prevent thyroid cancer metastasis.
Cells are able to undergo a transition between the epithelial and mesenchymal states in a flexible and dynamic manner during embryonic development.13 When the balance tilts to the mesenchymal direction in a process which is called epithelial–mesenchymal transition (EMT), numerous genes related to adhesion, mobility and cytoskeleton will be regulated to facilitate cells adopting a migratory and invasive behavior.14 Tumor metastatic progression follows a well-defined sequence of events.15 To achieve migration, cell morphological changes will take place first. The moving cell becomes polarized and elongated, and extends its leading edge. Then a pseudopod is formed, which attaches to the extracellular matrix (ECM) substrate to generate a traction force to overcome the resistance on the way ahead.16 From a macro perspective, the metastatic cascade of tumor cells includes local invasion to the neighboring tissue following the disruption of the basement membrane as well as intra- and extravasation of blood or lymph vessels.
Transient receptor potential vanilloid type 1 (TRPV1) is a nonselective, ligand-gated cation-channel belonging to the transient receptor potential family. TRPV1 was first identified in a subset of peripheral sensory neurons and plentiful non-neuronal systems in the mammalian body, participating in the sensory conduction of pain. The channel can be activated by high temperature, low pH and multiple chemical substances, especially capsaicin.17 TRPV1 is one of the most well-known mammalian transient receptor potential channel superfamily members. The biological and physiological properties of TRPV1, including intracellular signaling regulation and its role in health and disease, have been extensively studied.18 Growing evidence manifests that ion channels not only modulate the membrane potential, ion flux and electric signaling in excitable cells but also play important roles in cell proliferation, migration, apoptosis and differentiation.19 Currently, the studies focused on the role of ion channels in cancer progression have undergone a rapid development.20 Recent research studies have revealed that some TRP channels are differentially expressed between normal and tumor tissues, demonstrating that TRP may be associated with cancer development.21
In the present study, we identified that capsaicin could directly activate TRPV1 receptors in BCPAP cells, consequently suppress the EMT and ultimately inhibit cell adhesion, migration and invasion. This report clarified the molecular mechanisms hiding behind the anti-metastatic effects of capsaicin and discussed the role of TRPV1, which further advanced the anti-cancer evidence of capsaicin. These findings may also contribute to the development of a treatment method against metastatic thyroid cancer.
2. Materials and methods
2.1 Chemicals, reagents and antibodies
Capsaicin, concanavalin A and poly-lysine were purchased from Sigma (St Louis, MO, USA). The Matrigel Basement Membrane Matrix was purchased from Becton Dickinson (San Jose, California, USA). Transwell inserts (8.0 μm) were obtained from Merck Millipore (Massachusetts, USA). Capsazepine (CPZ) was purchased from MedChem Express (New Jersey, USA). Albumin from bovine serum (BSA), methyl thiazolyl tetrazolium (MTT) and dimethyl sulfoxide (DMSO) were purchased from Sangon (Shanghai, China). Crystal violet staining solution was purchased from Beyotime Institute of Biotechnology (Nantong, China). The UltraSYBR mixture was obtained from CWBIO (Beijing, China). The antibodies used in this research are as follows: anti-E-cadherin (sc-8426), anti-β-actin (sc-8432), anti-GAPDH (sc-47724), anti-MMP-9 (sc-12759), goat anti-mouse (sc-2005) and rabbit (sc-2004) IgG-HRP were purchased from Santa Cruz Biotechnology (California, USA). Anti-MMP-2 (AF0234) and anti-β-tubulin (AF1216) were purchased from Beyotime Institute of Biotechnology (Nantong, China). Anti-TRPV1 (bs-1931R), anti-Snail1 (bs-1382R) and anti-Twist1 (bs-2441R) were purchased from BIOSS (Beijing, China). All of the other chemicals were of the highest analytical grade and purchased from common sources.
2.2 Cell culture, transfection and drug treatments
Normal thyroid follicular epithelial cell Nthy-ori-3.1, follicular thyroid cancer cell FTC-133 and anaplastic thyroid cancer cell 8505C were purchased from the European Collection of Cell Cultures (ECACC, Wiltshire, UK). Papillary thyroid carcinoma BCPAP cells were obtained from the German Collection of Micro-organisms and Cell Cultures (Braunschweig, Germany). Nthy-ori-3.1 cells were maintained in RPMI 1640 containing 10% fetal bovine serum (FBS). BCPAP cells were cultured in RPMI 1640 containing 10% new-born bovine serum (NBS). FTC-133 cells were cultured with a complete medium containing DMEM and Ham's F-12 (v
:
v = 1
:
1) supplemented with 10% NBS. 8505C cells were maintained in MEM containing 10% NBS, and all cell culture media contain 100 U ml−1 penicillin and 100 U ml−1 streptomycin. All cell lines were incubated under a humid atmosphere of 5% (v/v) CO2 at 37 °C. Cells were transfected with shTRPV1 plasmids with a Lipo6000 transfection reagent (Beyotime, C0528) according to the manufacturer's instructions. Capsaicin was dissolved in DMSO at 100 mM and stored at 4 °C until diluted before use. BCPAP was treated with capsaicin at different concentrations (25–100 μM) for an indicated time. The solvent control contains the corresponding amount of DMSO to the highest used concentration of capsaicin, and the final DMSO concentration was lower than 0.1%.
2.3 Cell viability assay
Cell viability was analyzed by the MTT assay. In brief, the cells were seeded into a 96-well plate (7500 cells per well). Capsaicin (final concentration of 25–100 μM) was added into the wells in sextuplicate, and the plate was incubated at 37 °C for 24 h. Subsequently, the cells were treated with 40 μl of MTT solution (2 mg ml−1) for another 4 h. The supernatants were aspirated carefully and 100 μl of DMSO was added, then the plate was placed on a vibrator for 5 min. The optical density of the cell suspension was measured at 490 nm using a microplate reader (Epoch, Biotek). The cell viability was expressed as a percentage of MTT reduction, assuming that the absorbance of the untreated cells was 100%.
2.4 Wound healing assay
Cells were seeded in a six-well plate and cultured until reaching single-layer confluence. Linear wounds were scratched on the monolayer of cells using pipette tips. To inhibit proliferation, the cells were cultured under serum-deprivation conditions. Wound images were taken with a digital camera at 0, 24 and 48 h. The wound gap widths were measured using Image J software. The wound closure rate was calculated according to the formula: wound closure rate (%) = (gap width in 0 h − the remaining gap width)/gap width in 0 h × 100%.
2.5 Cell migration and invasion assay
The migration and invasion of BCPAP cells were measured by using the transwell assay as described previously with some modifications.22 For the migration assay, after being pre-treated with different dosages of capsaicin for 24 h, 200 μl of BCPAP cell suspension were added to the upper chamber of a transwell chamber at a density of 5 × 105 cells per ml. The pore size of the transwell apparatus is 8.0 μm. The upper chamber cultivation system contained 1% NBS, and the lower contained 500 μl of complete medium supplemented with 10% NBS. After 1 h of incubation, the stranded cells were removed from the upper surface of the membrane by gently scrubbing using a cotton tipped swab. For the invasion assay, the upper chamber was pre-coated with 50 μl Matrigel (1
:
10 diluted with serum-free medium). 200 μl of BCPAP cell suspension was seeded into the upper chamber at a density of 106 cells per ml in a conditioned medium containing 1% FBS, and incubated for 24 h followed by the removal of the cells that remained in the upper chamber with cotton swabs. The cells in both migration and invasion assays that had moved to the lower surface of the chamber were fixed with methanol and stained with crystal violet. Nine random fields were selected and photographs of the fixed cells were captured under an inverted microscope. Photographs were analyzed by using Image J software.
2.6 Cell adhesion assay
The cell adhesion assay was performed as described previously with some modifications.22 96-Well plates were pre-coated with 100 μl Matrigel (1
:
10 diluted with serum-free medium), 100 μl poly-lysine (25 μg ml−1), 100 μl concanavalin A (25 μg ml−1) or 100 μl 0.2% (m/v) BSA overnight at 4 °C, respectively. Before use, the plates were coated with 0.2% (m/v) BSA for 2 h at room temperature followed by washing with PBS twice. Then 100 μl untreated or capsaicin-treated cell suspensions (2.5 × 105 cells per ml) were collected and seeded onto the matrix surface. The plate was incubated at 37 °C for 0.5 h and then it was gently washed three times with warm PBS to remove the unattached cells. The remaining attached cells were incubated with MTT (2 mg ml−1) at 37 °C for 4 h. After incubation, the supernatant was aspirated and 100 μl of DMSO was added. The absorbance of the color substrate was measured with a microplate reader (Epoch, BioTek) at 490 nm. Cells attaching to BSA-coated wells were considered as the background, and the rate of attachment was calculated by using the following formula: attachment rate (%) = (OD value of the matrix coated group − OD value of the BSA coated group)/OD value of the non-washing group.
2.7 Reverse transcriptase-polymerase chain reaction (RT-PCR)
Total RNA was extracted from the treated cells using the TRIzol Reagent (Invitrogen) according to the manufacturer's protocol. cDNA was synthesized from 2 μg of RNA with oligo(dT)18 primers using M-MLV reverse transcriptase (TaKaRa, Japan) according to the manufacturer's instructions. Following cDNA synthesis, the PCR was performed, using the conditions of 95 °C for 30 s, 53–65 °C (depending on the sequences of the primers) for 30 s and 72 °C for 30 s for 35 cycles. The specific primers are as follows: Human TRPV1 forward: 5′-ACACACCTGATGGCAAGGAC-3′ and reverse: 5′-AGACTGCCTATCTCGAGCAC-3′; Human TRPV2 forward: 5′-aagcggaggttggaaactcc-3′ and reverse: 5′-cactgactctgtggcattgg-3′; Human TRPV3 forward: 5′-tgcctgacttcctcatgcac-3′ and reverse: 5′-gactgaggatgtacttcagg-3′; Human TRPV4 forward: 5′-acattgtcaactacctgacgg-3′ and reverse: 5′-acaggtaggagaccacgttg-3′; Human TRPV5 forward: 5′-cagagaacaagactgggacc-3′ and reverse: 5′-tccatgctcaatgagcagc-3′; Human TRPV6 forward: 5′-GCTTTGCTTCAGCCTTCT-3′ and reverse: 5′-CAGTGAGTGTCGCCCATC-3′; Human Actin forward: 5′-GCCGGGACCTGACTGACTAC-3′ and reverse: 5′-CGGATGTCCACGTCACACTT-3′. The PCR products were electrophoresed in 1.5% agarose gel and visualized by ethidium bromide (EB) dyeing.
2.8 Real-time PCR
For real-time PCR assays, reverse transcribed cDNA samples were amplified using a 7500 Real-time PCR detection system (Applied Biosystems). The SYBR green mixture was used for PCR reactions. The 2−ΔΔCt (Livak) method was used to conduct data analysis, and GAPDH was used as an internal control. The primers are listed below. ZEB1 forward: 5′-GCACAAGAAGAGCCACAAGTA-3′ and reverse: 5′-GCAAGACAAGTTCAAGGGTTC-3′; ZEB2 forward: 5′-GACAGATCAGCACCAAATGC-3′ and reverse: 5′-GCTGATGTGCGAACTGTAGG-3′; Snail1 forward: 5′-TCGGAAGCCTAACTACAGCGA-3′ and reverse: 5′-GCTGGAAGGRAAACTCTGGATTA-3′; Snail2 forward: 5′-CCTTCTCCAGAATGTCTCTC-3′ and reverse: 5′-ATTTGGTTGGTCAGCACAGGAGA-3′; Twist1 forward: 5′-TACATCGACTTCCTCTACCAGGTC-3′ and reverse: 5′-TAGTGGGACGCGGACATGGA-3′; Twist2 forward: 5′-GGCGCGCCAGGAGGAGATTCT-3′ and reverse: 5′-TGCTCACTCCCGCCAACGTT-3′; GAPDH forward: 5′-TCAAGAAGGTGGTGAAGCA-3′ and reverse: 5′-AAAGGTGGAGGAGTGGGT-3′.
2.9 Western blotting
Western blotting was performed as previously described with some modifications.23 Cell lysates were extracted by using RIPA buffer containing a protease inhibitor cocktail. The protein concentration was determined using the BCA Protein Assay kit (Beyotime) according to the manufacturer's protocol. 50 μg of total protein was separated by 10% SDS-polyacrylamide gel electrophoresis (SDS-PAGE). The proteins were electroblotted onto a nitrocellulose (NC) membrane. The membranes were probed with appropriate primary antibodies, followed by incubation with a horseradish peroxidase conjugated secondary antibody. The blots were visualized using an ECL Western blot kit (ABXBio) and imaged using the ChemiDoc XRS+ detection system.
2.10 Construction of human LAMP2AshRNA plasmids
Vector pGenesil-1 (Yrbio) was modified to express an shRNA from the U6 promoter as described.24 Briefly, an oligonucleotide was synthesized that consisted of a sequence-specific 19 nucleotide stretch designed to target the TRPV1 (NM_018727.5) followed by the loop sequence (TTCAAGAGA) and finally the reverse complement of the targeting sequence. A termination sequence (TTTTTGGAA), a restriction enzyme recognition site (GAATTC), a 5′ cloning site (GATCC) and a 3′ cloning site (TTCGA) were added to facilitate directional cloning immediately downstream of the U6 promoter using BamH I/Hind III cloning sites. shRNA-TRPV1-#1 sense, 5′-![[G with combining low line]](https://www.rsc.org/images/entities/char_0047_0332.gif)
![[A with combining low line]](https://www.rsc.org/images/entities/char_0041_0332.gif)
![[T with combining low line]](https://www.rsc.org/images/entities/char_0054_0332.gif)
![[C with combining low line]](https://www.rsc.org/images/entities/char_0043_0332.gif)
GAAGACCTGTCTGCTGAAA TTCAAGAGA TTTCAGCAGACAGGTCTTC TTTTTGGAA ![[G with combining low line]](https://www.rsc.org/images/entities/char_0047_0332.gif)
![[A with combining low line]](https://www.rsc.org/images/entities/char_0041_0332.gif)
![[A with combining low line]](https://www.rsc.org/images/entities/char_0041_0332.gif)
![[T with combining low line]](https://www.rsc.org/images/entities/char_0054_0332.gif)
![[T with combining low line]](https://www.rsc.org/images/entities/char_0054_0332.gif)
A-3′ and antisense, 5′-![[A with combining low line]](https://www.rsc.org/images/entities/char_0041_0332.gif)
![[G with combining low line]](https://www.rsc.org/images/entities/char_0047_0332.gif)
![[C with combining low line]](https://www.rsc.org/images/entities/char_0043_0332.gif)
![[T with combining low line]](https://www.rsc.org/images/entities/char_0054_0332.gif)
![[T with combining low line]](https://www.rsc.org/images/entities/char_0054_0332.gif)
![[G with combining low line]](https://www.rsc.org/images/entities/char_0047_0332.gif)
![[A with combining low line]](https://www.rsc.org/images/entities/char_0041_0332.gif)
![[A with combining low line]](https://www.rsc.org/images/entities/char_0041_0332.gif)
![[T with combining low line]](https://www.rsc.org/images/entities/char_0054_0332.gif)
![[T with combining low line]](https://www.rsc.org/images/entities/char_0054_0332.gif)
TTCCAAAAA GAAGACCTGTCTGCTGAAA TCTCTTGAA TTTCAGCAGACAGGTCTTCG-3′; shRNA-TRPV1-#2 sense, 5′-![[G with combining low line]](https://www.rsc.org/images/entities/char_0047_0332.gif)
![[A with combining low line]](https://www.rsc.org/images/entities/char_0041_0332.gif)
![[T with combining low line]](https://www.rsc.org/images/entities/char_0054_0332.gif)
![[C with combining low line]](https://www.rsc.org/images/entities/char_0043_0332.gif)
CGAGCATGTACAATGAGAT TTCAAGAGA ATCTCATTGTACATGCTCG TTTTTGGAA ![[G with combining low line]](https://www.rsc.org/images/entities/char_0047_0332.gif)
![[A with combining low line]](https://www.rsc.org/images/entities/char_0041_0332.gif)
![[A with combining low line]](https://www.rsc.org/images/entities/char_0041_0332.gif)
![[T with combining low line]](https://www.rsc.org/images/entities/char_0054_0332.gif)
![[T with combining low line]](https://www.rsc.org/images/entities/char_0054_0332.gif)
A-3′ and antisense, 5′-![[A with combining low line]](https://www.rsc.org/images/entities/char_0041_0332.gif)
![[G with combining low line]](https://www.rsc.org/images/entities/char_0047_0332.gif)
![[C with combining low line]](https://www.rsc.org/images/entities/char_0043_0332.gif)
![[T with combining low line]](https://www.rsc.org/images/entities/char_0054_0332.gif)
![[T with combining low line]](https://www.rsc.org/images/entities/char_0054_0332.gif)
![[G with combining low line]](https://www.rsc.org/images/entities/char_0047_0332.gif)
![[A with combining low line]](https://www.rsc.org/images/entities/char_0041_0332.gif)
![[A with combining low line]](https://www.rsc.org/images/entities/char_0041_0332.gif)
![[T with combining low line]](https://www.rsc.org/images/entities/char_0054_0332.gif)
![[T with combining low line]](https://www.rsc.org/images/entities/char_0054_0332.gif)
TTCCAAAAA CGAGCATGTACAATGAGAT TCTCTTGAA ATCTCATTGTACATGCTCGG-3′; shRNA-TRPV1-#3 sense, 5′-![[G with combining low line]](https://www.rsc.org/images/entities/char_0047_0332.gif)
![[A with combining low line]](https://www.rsc.org/images/entities/char_0041_0332.gif)
![[T with combining low line]](https://www.rsc.org/images/entities/char_0054_0332.gif)
![[C with combining low line]](https://www.rsc.org/images/entities/char_0043_0332.gif)
CGCATCTTCTACTTCAACT TTCAAGAGA AGTTGAAGTAGAAGATGCG TTTTTGGAA ![[G with combining low line]](https://www.rsc.org/images/entities/char_0047_0332.gif)
![[A with combining low line]](https://www.rsc.org/images/entities/char_0041_0332.gif)
![[A with combining low line]](https://www.rsc.org/images/entities/char_0041_0332.gif)
![[T with combining low line]](https://www.rsc.org/images/entities/char_0054_0332.gif)
![[T with combining low line]](https://www.rsc.org/images/entities/char_0054_0332.gif)
A-3′ and antisense, 5′-![[A with combining low line]](https://www.rsc.org/images/entities/char_0041_0332.gif)
![[G with combining low line]](https://www.rsc.org/images/entities/char_0047_0332.gif)
![[C with combining low line]](https://www.rsc.org/images/entities/char_0043_0332.gif)
![[T with combining low line]](https://www.rsc.org/images/entities/char_0054_0332.gif)
![[T with combining low line]](https://www.rsc.org/images/entities/char_0054_0332.gif)
![[G with combining low line]](https://www.rsc.org/images/entities/char_0047_0332.gif)
![[A with combining low line]](https://www.rsc.org/images/entities/char_0041_0332.gif)
![[A with combining low line]](https://www.rsc.org/images/entities/char_0041_0332.gif)
![[T with combining low line]](https://www.rsc.org/images/entities/char_0054_0332.gif)
![[T with combining low line]](https://www.rsc.org/images/entities/char_0054_0332.gif)
TTCCAAAAA CGCATCTTCTACTTCAACT TCTCTTGAA AGTTGAAGTAGAAGATGCGG-3′. The control scrambled shRNA was constructed by the insertion of a similar structure but encoding a nonsense minigene with no homology to any known sequences in human and mouse genomes. The sequences for scrambled-siRNA are as follows: sense, 5′-![[G with combining low line]](https://www.rsc.org/images/entities/char_0047_0332.gif)
![[A with combining low line]](https://www.rsc.org/images/entities/char_0041_0332.gif)
![[T with combining low line]](https://www.rsc.org/images/entities/char_0054_0332.gif)
![[C with combining low line]](https://www.rsc.org/images/entities/char_0043_0332.gif)
CGCTTACGCTGAGTACTTCGA TTCAAGAGA GAATGCGACTCATGAAGCT TTTTTGGAA ![[G with combining low line]](https://www.rsc.org/images/entities/char_0047_0332.gif)
![[A with combining low line]](https://www.rsc.org/images/entities/char_0041_0332.gif)
![[A with combining low line]](https://www.rsc.org/images/entities/char_0041_0332.gif)
![[T with combining low line]](https://www.rsc.org/images/entities/char_0054_0332.gif)
![[T with combining low line]](https://www.rsc.org/images/entities/char_0054_0332.gif)
A-3′ and antisense, 5′-![[A with combining low line]](https://www.rsc.org/images/entities/char_0041_0332.gif)
![[G with combining low line]](https://www.rsc.org/images/entities/char_0047_0332.gif)
![[C with combining low line]](https://www.rsc.org/images/entities/char_0043_0332.gif)
![[T with combining low line]](https://www.rsc.org/images/entities/char_0054_0332.gif)
![[T with combining low line]](https://www.rsc.org/images/entities/char_0054_0332.gif)
![[G with combining low line]](https://www.rsc.org/images/entities/char_0047_0332.gif)
![[A with combining low line]](https://www.rsc.org/images/entities/char_0041_0332.gif)
![[A with combining low line]](https://www.rsc.org/images/entities/char_0041_0332.gif)
![[T with combining low line]](https://www.rsc.org/images/entities/char_0054_0332.gif)
![[T with combining low line]](https://www.rsc.org/images/entities/char_0054_0332.gif)
TTCCAAAAA AGCTTCATGAGTCGCATTC TCTCTTGAA TCGAAGTACTCAGCGTAAGCGG-3′. Recombinant chimeric plasmids were confirmed by restriction enzyme digestion (EcoRI) and DNA sequencing analysis (Sangong).
2.11 Statistical analysis
The resultant values are all presented as mean ± SEM. The Student's t test was performed to analyze the statistical significance. P < 0.05 was considered as statistically significant.
3. Results
3.1 Effects of capsaicin on the cell viability of BCPAP cells
To explore the potential function of capsaicin, we first determined its cytotoxicity on papillary thyroid cancer BCPAP cells. The cell viability was examined by MTT assay. As illustrated in Fig. 1B, when cells were exposed to different doses of capsaicin, the viability of the BCPAP cells showed no obvious change. Until treated with 50–100 μM of capsaicin for 48 h, the cell viability started to act out a decreasing trend. However, the cell viability was merely reduced to approximately 90% at 48 h after the maximum concentration of capsaicin treatment, although the data have shown a significant difference statistically (P < 0.01). According to these results, we considered that capsaicin showed no apparent inhibitory effect on the cell viability of BCPAP cells.
3.2 Capsaicin inhibits migration and invasion of BCPAP cells
The diffusion of cancer cells from the primary tumor to the surrounding or distant organs has become the most fatal risk to thyroid cancer patients. Next, we evaluated whether capsaicin has an efficacy in regulating the metastatic properties of BCPAP cells. Wound healing and transwell assay were performed to detect the migratory capability of BCPAP cells. As shown in Fig. 2A, capsaicin inhibited the migration of BCPAP cells in a dose-dependent manner. Compared with solvent control, 100 μM of capsaicin treatment for 48 h decreased the wound closure rate from 90.26 ± 5.35% to 18.04 ± 2.71% (P < 0.01) (Fig. 2B). To support these results, the transwell assay was carried out to further verify the suppression effect of capsaicin on the migration and invasion capability of BCPAP cells. As shown in Fig. 2C and D, in the transwell chamber setting, capsaicin decreased the migration and invasion rate of BCPAP cells significantly in a dose-dependent manner, which was consistent with the above-mentioned findings.
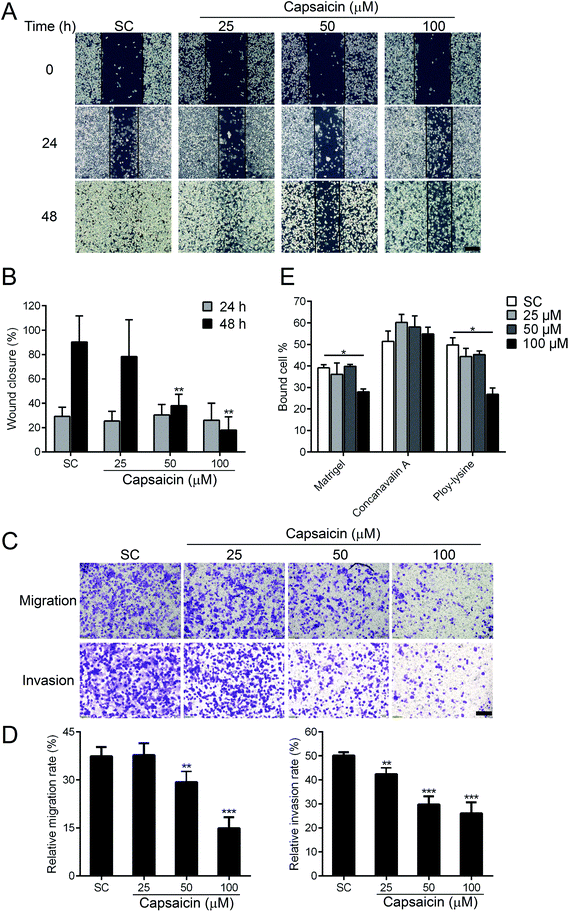 |
| Fig. 2 Capsaicin inhibits the migration and invasion of BCPAP cells. (A) Capsaicin exhibited inhibitory effects on the migration of BCPAP cells in the wound healing assay. Cells were exposed to different concentrations of capsaicin for 24 or 48 h after three perpendicular wounds were scratched, and the images were captured using a phase contrast microscope at indicated times. Representative images of three independent experiments are shown. Scale bar, 100 μm. (B) Quantitative analysis of wound closures in (A) is shown in the histogram. (C) The effects of capsaicin on the migration and invasion of BCPAP cells were determined by using transwell assays. Scale bar, 60 μm. (D) Graphs show quantitation of cell migration or invasion. (E) Capsaicin affects the adhesion of BCPAP cells to different types of ECM. The same number of capsaicin-treated cells was plated onto ECM-coated plates. Cells remaining attached to the plates were incubated with MTT after washing and the optical density was detected. The percent of bound cells was calculated. All data were represented as the mean ± SEM of three independent experiments. Statistical analysis was performed using an unpaired Student's t test. *P < 0.05, **P < 0.01 and ***P < 0.001 vs. solvent control. | |
For extravasation, it is necessary for the cancer cells to seed into distant organs to form distal foci. This process depends on altered adhesiveness.25 To investigate whether capsaicin could also influence the adhesion process of BCPAP cells to different ECM components, a cell adhesion assay was performed. We observed that capsaicin treatment for 24 h tremendously inhibited the cell adhesion to Matrigel, a reconstituted basement and poly-lysine. However, it did not affect the cell adhesion to concanavalin A (Fig. 2E). Above all, these results uncover a previously unrecognized function of capsaicin on thyroid cancer and suggest that capsaicin is an effective chemical against the metastasis of thyroid cancer cells.
3.3 Capsaicin regulates the expression of EMT-related genes
EMT is an important mechanism taking part in many cellular biological activities, such as embryonic development and tissue repair.13 Meanwhile, EMT is believed to be activated in carcinoma and is concerned with cancer cell dissociation from the primary tumor and their intravasation into blood vessels for further hematogenous metastasis.13 Several transcription factors such as Snail, ZEB and Twist families trigger EMT.26 The significant inhibition effect of capsaicin on the metastasis of thyroid cancer cells prompted us to investigate the molecular mechanisms with respect to EMT. Stimulated by capsaicin, the mRNA expression levels of some EMT-TFs in BCPAP cells dramatically reduced, such as Twist2, Snail1 and Snail2 (Fig. 3A). For other EMT-TFs, the mRNA level of Twist1 was increased to a certain extent. The expression of the ZEB family was slightly affected by 100 μM of capsaicin treatment but with no significant difference. It should be noted that capsaicin treatment resulted in a sharp decrease in the protein levels of Snail1 and Twist1 (Fig. 3B). Considering that the mRNA level of Twist1 was even slightly up-regulated, capsaicin may decrease the protein level of Twist1 by affecting its stability. As the direct target of Snail and Twist, the protein level of E-cadherin was also up-regulated (Fig. 3B).
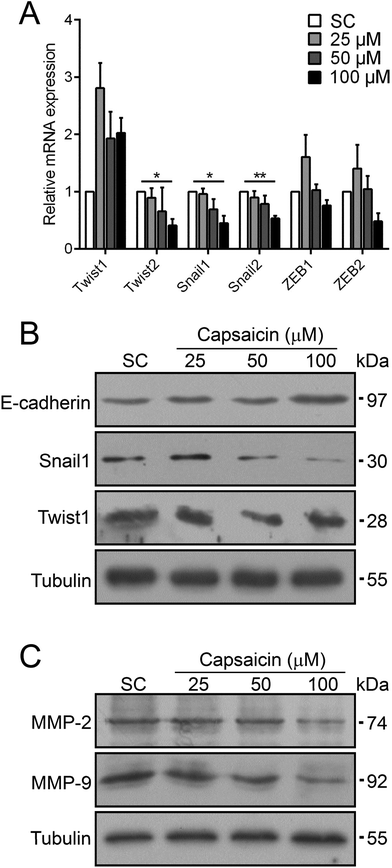 |
| Fig. 3 Capsaicin regulates the expression of EMT-related genes. (A) Capsaicin treatment decreased the mRNA expression of Twist2, Snail1 and Snail2 of BCPAP cells. Cells were treated with different concentrations of capsaicin, and the mRNA expression levels of EMT-TFs were analyzed by Q-PCR. Data are represented as mean ± SEM (n = 3). Statistical analysis was performed using an unpaired Student's t test. *P < 0.05 and **P < 0.01 vs. solvent control. (B and C) Capsaicin downregulates the protein levels of Snail1, Twist1, MMP-2 and MMP-9. Cells were treated with different dosages of capsaicin for 24 h and then subjected to western blot analysis with indicated antibodies. Representative bands are shown. Tubulin was used as a loading control. | |
Matrix metalloproteinases (MMPs) are well-recognized enzymes that digest the main proteins involved in cell–cell or cell–ECM interactions to facilitate cancer cell diffusion.27 The inhibition efficacy of capsaicin on the migration and invasion of thyroid cancer cells prompted us to detect the change of MMP expression. As shown in Fig. 3C, the protein levels of MMP-2 and MMP-9 were also drastically reduced after capsaicin treatment. Taken together, these data implied that capsaicin abolished the phenotype of EMT in BCPAP cells by means of decreasing the expressions of EMT-TFs including Twist2, Snail1 and Snail2. Besides this, capsaicin inhibited the expression of MMPs to suppress the degradation of ECMs of thyroid cancer cells.
3.4 Capsaicin exerts its inhibitory effects on the metastasis of BCPAP cells via the TRPV1 channel
It is well-known that capsaicin is a specific ligand to TRPV1.17 The activation of TRPV1 by capsaicin was closely associated with its anti-proliferative and anti-metastatic effects on various types of cancer cells.6 Hence, we detected the expression profiles of human TRPV channels in various thyroid cell lines by RT-PCR. As depicted in Fig. 4A, compared with other members of the TRPV family, TRPV1 mRNA was intensively and generally expressed in both normal and thyroid cancer cell lines. In addition, the protein levels of TRPV1 of normal thyroid cell lines and three other thyroid carcinoma cell lines were assessed by western blotting. As shown in Fig. 4B, the endogenous protein levels of TRPV1 in thyroid cancer cell lines were universally much higher than that of the normal thyroid cell line. The BCPAP cells robustly expressed TRPV1. The expressions of TRPV1 in FTC-133 and 8505C cells were moderate. In order to find out whether the anti-metastatic effect of capsaicin in our study relied on its affinity to TRPV1, short hairpin RNAs (shRNAs) were constructed to silence the endogenous TRPV1 expression of BCPAP cells. Compared with the scramble shRNA, the transfection of three TRPV1 shRNA plasmids targeting different regions of human TRPV1 mRNA into BCPAP cells profoundly downregulated the levels of TRPV1 proteins as determined by western blotting (Fig. 4C). As depicted in Fig. 4D and E, capsaicin showed a weak inhibitory effect on TRPV1-downregulated BCPAP cells, indicating that capsaicin might exert its migration inhibitory effect on thyroid cancer cells in a TRPV1-dependent manner.
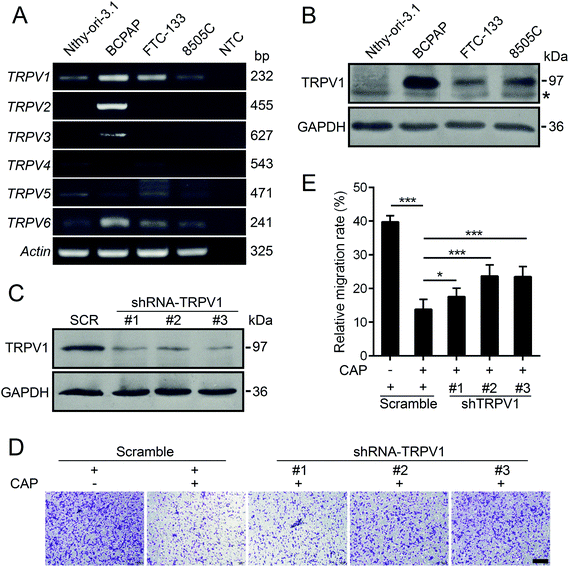 |
| Fig. 4 The profiles of TRPV expression in normal or different thyroid cancer cell lines. Total RNAs or proteins were extracted from Nthy-ori-3.1, BCPAP, FTC-133 and 8505C cells, and the mRNA levels of different TRPVs were determined by RT-PCR in (A). Protein levels of TRPV1 were determined by western blotting in (B). The asterisk indicated a non-specific band. (C) TRPV1 knockdown was confirmed by western blotting. BCPAP cells were transfected with siRNAs targeting the TRPV1 protein (shTRPV1) or negative control (Scramble), and the lysates were collected 48 h later. (D) The anti-migratory effect of capsaicin on BCPAP cells was attenuated by TRPV1 knockdown. Cells were individually transfected with scramble shRNA, shTRPV1-#1, #2 and #3 for 48 h, and subsequently the cells were treated with 100 μM capsaicin or DMSO for another 24 h. The migration capacity was analyzed by using the transwell assay. Scale bar, 60 μm. (E) Quantitative analysis of cell migration in (D). Data represent the mean ± SEM. *P < 0.05, ***P < 0.001. | |
Capsazepine is a synthetic analogue of capsaicin, which serves as a competitive antagonist against the activation of TRPV1 by capsaicin.28 We thus determined whether capsazepine could attenuate the inhibitory effects of capsaicin on BCPAP metastasis. Using transwell assays, we found that pre-treatment with 10 μM of capsazepine for 2 h dramatically abolished the inhibition effect of capsaicin on cell migration and invasion (Fig. 5A and B). What is worth pointing out is that the cell mobility was not affected by capsazepine alone, moreover, neither individual nor combined treatment of capsazepine and capsaicin influenced the cell viability (Fig. 5C). In addition, the western blot assay showed that capsazepine treatment partially attenuated the capsaicin induced decrease of Twist1. The decreased expression of MMP-9 was substantially reversed by capsazepine as well (Fig. 5D). Taken together, our results indicated that capsaicin mediated inhibition of papillary thyroid cancer metastasis was at least in part through TRPV1 activation.
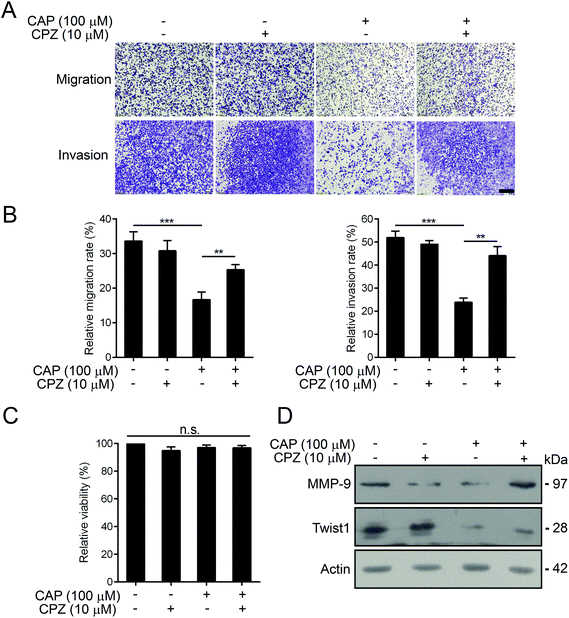 |
| Fig. 5 Capsaicin exerts its inhibitory effects on the metastasis of BCPAP cells in a TRPV1-dependent manner. (A) The anti-migratory and anti-invasive effects of capsaicin on BCPAP cells were abolished by capsazepine. Cells were exposed to 100 μM of capsaicin for 24 h or 10 μM of capsazepine for 2 h alone. In the case of combination, cells were pre-treated with 10 μM of capsazepine for 2 h and then cultured with 100 μM of capsaicin for another 24 h. Cell migration and invasion assays were performed by using the transwell assay. Scale bar, 60 μm. (B) Quantitative analysis of cell migration and invasion rates in (A). Data are represented as the mean ± SEM of three independent experiments. Statistical analysis was performed using an unpaired Student's t test. **P < 0.01 and ***P < 0.001. (C) Effects of capsazepine on the cell viability of BCPAP cells. Cells were treated as in (A), and the cell viability was detected using the MTT assay. n. s., no significance, P > 0.05. (D) Capsaicin-induced decrease in the expression of MMP-9 and Twist1 was restored by capsazepine. Cells were treated as in (A), and then the protein levels of MMP-9 and Twist1 were determined by western blotting. Actin was used as a loading control. | |
4. Discussion
There is persuasive epidemiological and experimental evidence that dietary phytochemicals possess anticancer activity. Capsaicin is one of the homovanillic acid derivatives and is primarily responsible for the spicy flavor in chili peppers, which has also been consumed as a herbal medicine for centuries. However, capsaicin has a hot and convoluted history of controversy about whether its consumption or clinical application is entirely safe. Conflicting epidemiological data and results obtained from basic research suggest that capsaicin can act as a carcinogen or as a cancer preventive agent.29,30 Moreover, whether capsaicin plays a crucial role in papillary thyroid carcinogenesis or progression and which possible molecular mechanisms may be involved in remain poorly understood.
First of all, we investigated the cytotoxicity of capsaicin with papillary thyroid cancer BCPAP cells. Unexpectedly, capsaicin at a concentration ranged from 25 to 100 μM failed to inhibit the cell viability of BCPAP cells. Even when the exposure time was extended to 48 h, the decreased cell viability induced by capsaicin was lower than 10% (Fig. 1).
In addition to proliferation, possessing high invasiveness and metastasis potency is another evil face of tumors. During clinical practice, papillary thyroid cancer with low risk often has a good prognosis after a complete surgical resection and subsequent administration of radioiodine.31 However, metastatic thyroid cancer often resists to conventional therapy and causes 80% of cancer-related death.32 Hence, metastasis has become a major challenge in cancer therapy. The versatility of capsaicin prompted us to determine whether capsaicin could block the metastatic steps of thyroid cancer cells. Metastasis occurs when cancer cells acquire the ability to invade the vasculature and migrate to distant organs. Besides this, metastasis is a complicated and multistep process, including cell migration, invasion and attachment.33 Our study provides solid evidence that capsaicin treatment results in a decrease in cell migration and invasion of BCPAP cells, which were proven by both wound healing and transwell assays in vitro. Besides that, we used Matrigel, poly-lysine and concanavalin A as the matrix of cell adhesion and found that capsaicin significantly decreased the cell attachment rate to Matrigel or poly-lysine (Fig. 2). It can be concluded that capsaicin suppressed the metastasis potential of BCPAP cells by regulating the adhesion related pathways.
Consistent with our observations, capsaicin suppressed the progression of many other types of cancer by its anti-migratory and anti-invasive activity. For example, capsaicin significantly reduced the cell migration and invasion of cholangiocarcinoma HuCCT1 cells via the inactivation of the NF-κB/p65 signaling pathway and subsequently suppressed the expression of MMP-9.34 In breast cancer MDA-MB-231 cells, capsaicin was found to suppress the cell migration and invasion by inhibiting the phosphorylation of c-Src, FAK and paxillin, and by down-regulating the expressions of MMP-2 and MMP-9.35 In a transgenic adenocarcinoma of the mouse prostate (TRAMP) model, capsaicin treatment demonstrated a significant reduction in the metastatic burden without leading to obvious pathological toxicities in the liver and gastrointestinal tract, which correlated to a reduction in p27.36 However, a few research studies hold a view that capsaicin is not a tumor-suppression but a tumor-promotion chemical due to the conflicting results from epidemiological and basic research studies. For instance, using both in vitro and in vivo colorectal cancer models, researchers confirmed that capsaicin at 100 μM enhanced the migratory and invasive capability of diverse colorectal cancer cell lines via the activation of Akt/mTOR and STAT-3 pathways.37 Another group stated that the cancer-promoting effect of capsaicin was due to the increased EGFR expression and subsequent reduced levels of NF-κB and cyclin D1, which enhanced lung epithelial cell proliferation and EMT during lung carcinogenesis.38 These controversial findings indicated that capsaicin could exert opposing two sides to the cancer cell metastasis, and the exact role of capsaicin in cancer progression is much more likely dependent on cancer types and different responses to stress stimulation.
Tumor metastasis is a complex and multistage process, among which, EMT has been considered as the most important mechanism. During embryonic development, cells transit between the epithelial and mesenchymal states in a highly plastic and dynamic manner. By means of modifying the adhesion molecules expressed by the cell, EMT enables it to adopt a migratory and invasive behavior. Recently, EMT has been believed to trigger the dissociation of cancer cells from primary carcinomas, which subsequently migrate and disseminate to distant sites.13 The EMT is executed in response to pleiotropic signaling factors that induce the expression of specific transcription factors called EMT-TFs. Many EMT-TFs are involved in embryonic development, wound healing, fibrosis, and cancer metastasis. Snail, a family belongs to zinc finger transcription factors, includes Snail1 and Snail2. The Twist family was identified as basic helix-loop-helix transcription factors, whose members are Twist1 and Twist2. The zinc finger E-box binding homeobox proteins, which are named Zeb family, consist of Zeb1 and Zeb2. The activation of these EMT-TFs leads to the downregulation of genes encoding epithelial junction proteins, and upregulation of proto-oncogenes facilitating tumor progression.39 Our study showed that capsaicin decreased the mRNA levels of Snail1, Snail2 and Twist2 but not Zeb. Snail1 is capable of binding to two E2-BOXES that are located proximal to the transcriptional start site of the E-cadherin gene to repress its transcription. Besides this, Twist family members are considered to repress E-cadherin expression by directly binding to its promoter.40 We found that the protein level of the epithelial marker, E-cadherin, was increased in BCPAP cells by capsaicin treatment as a consequence of the downregulation of Twist1 and Snail1. Intriguingly, at the protein level, capsaicin was able to downregulate the expression of Twist1 albeit its increased tendency in mRNA transcription. We speculated that the increased degradation of Twist1 or other mechanisms that affect the protein stability of Twist1 may account for this result. In fact, previous research revealed that post-translation regulation is a crucial regulatory mechanism for Twist. It has been reported that K63-mediated ubiquitination is involved in the regulation of protein stability of Twist. Stabilized Twist could translocate to the nucleus and trigger a subsequent EMT, thus facilitating cancer stem cell functions and leading to chemoresistance.41 These results suggest that capsaicin inhibits thyroid cancer cell metastasis at least in part by EMT suppression. The proteolytic degradation of ECM components by tumor cell-secreted proteases, such as matrix metalloproteinases, is a decisive step for the migration and invasion of tumor cells.42 Our finding also verified that capsaicin significantly restrained the expression of MMP-2 and MMP-9 in a dose-dependent manner (Fig. 3).
Previous studies revealed that the pro-apoptotic activities of capsaicin in various types of cancers were mostly mediated by TRPV143,44 and TRPV6.45,46 However, whether the anti-metastatic effect of capsaicin on BCPAP cells is mediated by TRPV channels remains unknown. The TRP vanilloid subfamily consists of six homologous members with diverse functions. Among these homologous members, TRPV1 and TRPV6 are universally expressed in different kinds of thyroid cell lines. Compared to TRPV6, the mRNA expression of TRPV1 is higher. Besides this, the protein levels of TRPV1 in thyroid cancer cell lines were generally higher than those of the normal thyroid cell line, indicating that TRPV1 could be a potential therapeutic drug target for thyroid cancer (Fig. 4). Notably, TRPV1, the most-studied member of the TRPV family, is reported to be the most specific receptor of capsaicin. Hence, we set about determining the potential role of TRPV1 participating in the anti-metastatic effects of capsaicin. As expected, the shRNA-mediated knockdown of TRPV1 resulted in an impaired suppression effect of capsaicin on BCPAP cell migration, which indicated that capsaicin might exert its migration inhibitory effect through TRPV1. In order to further cement this hypothesis, capsazepine, the competitive antagonist of capsaicin,28 was used. The decreased migration and invasion rates induced by capsaicin were significantly reduced by capsazepine without affecting the cell viability. Besides this, the downregulated protein expressions of Twist1 and MMP-9 were also restored by capsazepine treatment (Fig. 5). These results indicated that capsaicin inhibited the metastasis of BCPAP cells in a TRPV1-dependent manner.
5. Conclusions
In summary, our study provided evidence for the first time that capsaicin inhibits multiple steps of metastasis of papillary thyroid cancer BCPAP cells, including cell migration, invasion and adhesion. In addition, we concluded that capsaicin downregulates the expression of MMPs and partially inverses the EMT. Importantly, TRPV1 is involved in these effects induced by capsaicin, which may serve as a novel target for developing a new strategy for treating papillary thyroid cancer, especially for metastasis.
Conflicts of interest
There are no conflicts of interest to declare.
Acknowledgements
This study was funded by the grants from the National Natural Science Foundation of China (No. 81402214, 81602352 and 81602353), Wuxi Municipal Commission of Health and Family Planning (Q201608) and the Natural Science Foundation of Jiangsu Province (BK20171145).
References
- A. Chapa-Oliver and L. Mejía-Teniente, Capsaicin: From Plants to a Cancer-Suppressing Agent, Molecules, 2016, 21, 931–945 CrossRef PubMed.
- A. Galano and A. Martinez, Capsaicin, a tasty free radical scavenger: mechanism of action and kinetics, J. Phys. Chem. B, 2012, 116, 1200–1208 CrossRef CAS PubMed.
- A. C. Reddy and B. R. Lokesh, Studies on anti-inflammatory activity of spice principles and dietary n-3 polyunsaturated fatty acids on carrageenan-induced inflammation in rats, Ann. Nutr. Metab., 1994, 38, 349–358 CrossRef CAS PubMed.
- K. Sambaiah and M. N. Satyanarayana, Hypocholesterolemic effect of red pepper & capsaicin, Indian J. Exp. Biol., 1980, 18, 898–899 CAS.
- B. Frias and A. Merighi, Capsaicin, Nociception and Pain, Molecules, 2016, 21, 797–830 CrossRef PubMed.
- R. Clark and S. H. Lee, Anticancer Properties of Capsaicin Against Human Cancer, Anticancer Res., 2016, 36, 837–843 CAS.
- R. L. Siegel, K. D. Miller and A. Jemal, Cancer Statistics, CA-Cancer J. Clin., 2017, 67, 7–30 CrossRef PubMed.
- Y. E. Nikiforov and M. N. Nikiforova, Molecular genetics and diagnosis of thyroid cancer, Nat. Rev. Endocrinol., 2011, 7, 569–580 CrossRef CAS PubMed.
- E. L. Mazzaferri and S. M. Jhiang, Long-term impact of initial surgical and medical therapy on papillary and follicular thyroid cancer, Am. J. Med., 1994, 97, 418–428 CrossRef CAS PubMed.
- V. V. Vasko and M. Saji, Molecular mechanisms involved in differentiated thyroid cancer invasion and metastasis, Curr. Opin. Oncol., 2007, 19, 11–17 CrossRef CAS PubMed.
- S. I. Sherman, J. D. Brierley, M. Sperling, K. B. Ain, S. T. Bigos, D. S. Cooper, B. R. Haugen, M. Ho, I. Klein, P. W. Ladenson, J. Robbins, D. S. Ross, B. Specker, T. Taylor and H. R. Maxon 3rd, Prospective multicenter study of thyroiscarcinoma treatment: initial analysis of staging and outcome. National Thyroid Cancer Treatment Cooperative Study Registry Group, Cancer, 1998, 83, 1012–1021 CrossRef CAS PubMed.
- I. J. Nixon, M. M. Whitcher, F. L. Palmer, R. M. Tuttle, A. R. Shaha, J. P. Shah, S. G. Patel and I. Ganly, The impact of distant metastases at presentation on prognosis in patients with differentiated carcinoma of the thyroid gland, Thyroid, 2012, 22, 884–889 CrossRef PubMed.
- M. A. Nieto, R. Y. Huang, R. A. Jackson and J. P. Thiery, Emt: 2016, Cell, 2016, 166, 21–45 CrossRef CAS PubMed.
- J. P. Thiery, H. Acloque, R. Y. Huang and M. A. Nieto, Epithelial-mesenchymal transitions in development and disease, Cell, 2009, 139, 871–890 CrossRef CAS PubMed.
- G. P. Gupta and J. Massague, Cancer metastasis: building a framework, Cell, 2006, 127, 679–695 CrossRef CAS PubMed.
- P. Friedl and K. Wolf, Tumour-cell invasion and migration: diversity and escape mechanisms, Nat. Rev. Cancer, 2003, 3, 362–374 CrossRef CAS PubMed.
- M. J. Caterina, M. A. Schumacher, M. Tominaga, T. A. Rosen, J. D. Levine and D. Julius, The capsaicin receptor: a heat-activated ion channel in the pain pathway, Nature, 1997, 389, 816–824 CrossRef CAS PubMed.
- B. Nilius and V. Flockerzi, Mammalian transient receptor potential (TRP) cation channels. Preface, Handb. Exp. Pharmacol., 2014, 223, 5–6 Search PubMed.
- A. Litan and S. A. Langhans, Cancer as a channelopathy: ion channels and pumps in tumor development and progression, Front. Cell. Neurosci., 2015, 9, 86–97 Search PubMed.
- L. Leanza, A. Manago, M. Zoratti, E. Gulbins and I. Szabo, Pharmacological targeting of ion channels for cancer therapy: In vivo evidences, Biochim. Biophys. Acta, 2016, 1863, 1385–1397 CrossRef CAS PubMed.
- K. Kunzelmann, Ion channels
and cancer, J. Membr. Biol., 2005, 205, 159–173 CrossRef CAS PubMed.
- C. Y. Zhang, L. Zhang, H. X. Yu, J. D. Bao, Z. Sun and R. R. Lu, Curcumin inhibits invasion and metastasis in K1 papillary thyroid cancer cells, Food Chem., 2013, 139, 1021–1028 CrossRef CAS PubMed.
- C. Tan, L. Zhang, X. Cheng, X. F. Lin, R. R. Lu, J. D. Bao and H. X. Yu, Curcumin inhibits hypoxia-induced migration in K1 papillary thyroid cancer cells, Exp. Biol. Med., 2015, 240, 925–935 CrossRef CAS PubMed.
- L. Zhang, Y. Sun, M. Fei, C. Tan, J. Wu, J. Zheng, J. Tang, W. Sun, Z. Lv, J. Bao, Q. Xu and H. Yu, Disruption of chaperone-mediated autophagy-dependent degradation of MEF2A by oxidative stress-induced lysosome destabilization, Autophagy, 2014, 10, 1015–1035 CrossRef CAS PubMed.
- A. L. Berrier and K. M. Yamada, Cell-matrix adhesion, J. Cell. Physiol., 2007, 213, 565–573 CrossRef CAS PubMed.
- S. Lamouille, J. Xu and R. Derynck, Molecular mechanisms of epithelial-mesenchymal transition, Nat. Rev. Mol. Cell Biol., 2014, 15, 178–196 CrossRef CAS PubMed.
- K. Kessenbrock, C. Y. Wang and Z. Werb, Matrix metalloproteinases in stem cell regulation and cancer, Matrix Biol., 2015, 44–46, 184–190 CrossRef CAS PubMed.
- S. Bevan, S. Hothi, G. Hughes, I. F. James, H. P. Rang, K. Shah, C. S. Walpole and J. C. Yeats, Capsazepine: a competitive antagonist of the sensory neurone excitant capsaicin, Br. J. Pharmacol., 1992, 107, 544–552 CrossRef CAS PubMed.
- K. Bley, G. Boorman, B. Mohammad, D. McKenzie and S. Babbar, A comprehensive review of the carcinogenic and anticarcinogenic potential of capsaicin, Toxicol. Pathol., 2012, 40, 847–873 CrossRef CAS PubMed.
- A. M. Bode and Z. Dong, The two faces of capsaicin, Cancer Res., 2011, 71, 2809–2814 CrossRef CAS PubMed.
- J. A. Sipos and E. L. Mazzaferri, The therapeutic management of differentiated thyroid cancer, Expert Opin. Pharmacother., 2008, 9, 2627–2637 CrossRef CAS PubMed.
- J. E. Talmadge and I. J. Fidler, AACR centennial series: the biology of cancer metastasis: historical perspective, Cancer Res., 2010, 70, 5649–5669 CrossRef CAS PubMed.
- S. Turajlic and C. Swanton, Metastasis as an evolutionary process, Science, 2016, 352, 169–175 CrossRef CAS PubMed.
- G. R. Lee, S. H. Jang, C. J. Kim, A. R. Kim, D. J. Yoon, N. H. Park and I. S. Han, Capsaicin suppresses the migration of cholangiocarcinoma cells by down-regulating matrix metalloproteinase-9 expression via the AMPK-NF-kappaB signaling pathway, Clin. Exp. Metastasis, 2014, 31, 897–907 CrossRef CAS PubMed.
- B. H. Li and L. Yuan, Inhibitory effects of capsaicin on migration and invasion of breast cancer MDA-MB-231 cells and its mechanism, Shengli Xuebao, 2017, 69, 183–188 Search PubMed.
- N. A. Venier, T. Yamamoto, L. M. Sugar, H. Adomat, N. E. Fleshner, L. H. Klotz and V. Venkateswaran, Capsaicin reduces the metastatic burden in the transgenic adenocarcinoma of the mouse prostate model, Prostate, 2015, 75, 1300–1311 CrossRef CAS PubMed.
- J. Yang, T. Z. Li, G. H. Xu, B. B. Luo, Y. X. Chen and T. Zhang, Low-concentration capsaicin promotes colorectal cancer metastasis by triggering ROS production and modulating Akt/mTOR and STAT-3 pathways, Neoplasma, 2013, 60, 364–372 CrossRef CAS PubMed.
- S. Geng, Y. Zheng, M. Meng, Z. Guo, N. Cao, X. Ma, Z. Du, J. Li, Y. Duan and G. Du, Gingerol Reverses the Cancer-Promoting Effect of Capsaicin by Increased
TRPV1 Level in a Urethane-Induced Lung Carcinogenic Model, J. Agric. Food Chem., 2016, 64, 6203–6211 CrossRef CAS PubMed.
- K. T. Yeung and J. Yang, Epithelial-mesenchymal transition in tumor metastasis, Mol. Oncol., 2017, 11, 28–39 CrossRef PubMed.
- J. Yang, S. A. Mani, J. L. Donaher, S. Ramaswamy, R. A. Itzykson, C. Come, P. Savagner, I. Gitelman, A. Richardson and R. A. Weinberg, Twist, a master regulator of morphogenesis, plays an essential role in tumor metastasis, Cell, 2004, 117, 927–939 CrossRef CAS PubMed.
- H. J. Lee, C. F. Li, D. Ruan, S. Powers, P. A. Thompson, M. A. Frohman and C. H. Chan, The DNA Damage Transducer RNF8 Facilitates Cancer Chemoresistance and Progression through Twist Activation, Mol. Cell, 2016, 63, 1021–1033 CrossRef CAS PubMed.
- S. E. King, Matrix metalloproteinases: new directions toward inhibition in the fight against cancers, Future Med. Chem., 2016, 8, 297–309 CrossRef CAS PubMed.
- C. Amantini, M. Mosca, M. Nabissi, R. Lucciarini, S. Caprodossi, A. Arcella, F. Giangaspero and G. Santoni, Capsaicin-induced apoptosis of glioma cells is mediated by TRPV1 vanilloid receptor and requires p38 MAPK activation, J. Neurochem., 2007, 102, 977–990 CrossRef CAS PubMed.
- L. Zheng, J. Chen, Z. Ma, W. Liu, F. Yang, Z. Yang, K. Wang, X. Wang, D. He, L. Li and J. Zeng, Capsaicin enhances anti-proliferation efficacy of pirarubicin via activating TRPV1 and inhibiting PCNA nuclear translocation in 5637 cells, Mol. Med. Rep., 2016, 13, 881–887 CrossRef CAS PubMed.
- J. Chow, M. Norng, J. Zhang and J. Chai, TRPV6 mediates capsaicin-induced apoptosis in gastric cancer cells–Mechanisms behind a possible new “hot” cancer treatment, Biochim. Biophys. Acta, 2007, 1773, 565–576 CrossRef CAS PubMed.
- J. K. Lau, K. C. Brown, A. M. Dom, T. R. Witte, B. A. Thornhill, C. M. Crabtree, H. E. Perry, J. M. Brown, J. G. Ball, R. G. Creel, C. L. Damron, W. D. Rollyson, C. D. Stevenson, W. E. Hardman, M. A. Valentovic, A. B. Carpenter and P. Dasgupta, Capsaicin induces apoptosis in human small cell lung cancer via the TRPV6 receptor and the calpain pathway, Apoptosis, 2014, 19, 1190–1201 CrossRef CAS PubMed.
Footnote |
† These authors equally contributed to this work. |
|
This journal is © The Royal Society of Chemistry 2018 |