DOI:
10.1039/C7FO01092C
(Paper)
Food Funct., 2018,
9, 253-262
Inhibition of advanced glycation endproducts during fish sausage preparation by transglutaminase and chitosan oligosaccharides induced enzymatic glycosylation
Received
20th July 2017
, Accepted 2nd November 2017
First published on 8th November 2017
Abstract
Advanced glycation endproducts (AGEs) are the harmful products of non-enzymatic reactions in foods formed during the heating process. In order to reduce the content of AGEs in foods, the inhibitory effect of different proportions of transglutaminase (TGase) and chitosan oligosaccharides (COS) on the AGEs of seabream fish meat sausage was studied. The results indicated that the TGase/COS ratio of 1
:
1 could inhibit the formation of AGEs, showing especially a decreased Nε-carboxymethyl-lysine (CML) content and a twice higher inhibition rate (36.4%) than that by aminoguanidine (17.4%), a commonly effective positive inhibitor of AGEs. The data of lysine, fructosamine, and glucosamine contents, combined with sodium dodecyl sulfate-polyacrylamide gel electrophoresis and matrix-assisted laser desorption/ionization time-of-flight mass spectrometry analyses, demonstrated that cross-linking of proteins and glycosylation of glutamine induced by TGase and COS resulted in steric hindrance that inhibited glycation. These findings revealed that TGase catalyzed glycosylation with COS differently from general antioxidants had potential as an effective treatment to inhibit the formation of AGEs in fishery products.
1. Introduction
Advanced glycation endproducts (AGEs) are the products of the Maillard reaction, which is a non-enzymatic reaction between reducing sugars and proteins, lipids, nucleic acids, or other components in foods during the heating process.1–3 AGEs can be divided into two categories according to their fluorescence: fluorescent AGEs, such as pentosidine and crossline, and non-fluorescent AGEs, including Nε-carboxymethyl-lysine (CML), Nε-carboxyethyl-lysine (CEL), and pyrraline. CML, a main AGEs compounds, is an indicator of heat damage in foods.4 AGEs contribute flavor and aroma compounds to heated foods, but they also cause adverse effects on human health.5,6 Recently, a large number of studies have shown that AGEs are linked to chronic diseases, including coronary heart disease, kidney disease, Alzheimer's disease, and diabetes.6–9 De Courten et al. reported that a diet low in AGEs might reduce the risk of diabetes.10 Wu et al. claimed that lotus seedpod oligomeric procyanidins could inhibit the formation of AGEs in high-fat-diet rats.11 Hence, the inhibition of AGEs in heated foods would be an effective strategy to decrease chronic disease risks in people, especially overweight individuals.
Owing to the severity of AGE related disorders, the investigation of AGE formation inhibitors has received a lot of attention in the past few years. The formation of AGEs is an outcome of oxidation reactions, and antioxidant substances, such as polyphenols, flavones, and proanthocyanidins, are regarded as effective inhibitors of fluorescent AGEs and CML in a model food system.12–14 Chen et al. reported that CML could be inhibited in cooked beef patties by phenolic compounds.15 However, the inhibitory activity of phenol compounds would be drastically lowered by the heating process.16 Additionally, synthetic antioxidants have a high risk of damaging the stomach, liver, and other visceral organs. Although extracted natural antioxidants could reduce the content of AGEs and CML, they may adversely affect the food flavor.17 Furthermore, their safety in foods is yet to be verified. Thus, in pursuit of healthy alternatives, it is urgent to find a safe and effective way to inhibit AGEs in foods.
Transglutaminase (TGase) is an enzyme belonging to the class of transferases: it catalyzes acyltransfer reactions, crosslinking, and deamidation between protein intra- or inter-chain glutamine (Gln, Q) and lysine (Lys, K) peptide residues (Fig. 1a). It is widely known to modify the functional properties of proteins in food systems, including the water holding capacity, emulsion stability, gelation properties, and thermal stability.18–22 Recently, many reports have confirmed that glycosylation could improve the functional properties and biological activity of a protein under the effect of TGase. Chitosan oligosaccharides (COS) have one amino group and two hydroxyl groups in the repeated residues of glycoside (Fig. 1b).23 It has been confirmed that COS could be crosslinked with Gln by TGase, and amino groups in COS were introduced by the formation of an amino bond (–CO–NH–) with the acylamino groups in Gln of the peptide chain.24 Glucosamine (GlcN) is the monomer of COS. Gottardi et al. reported that gluten hydrolysates with GlcN at mild temperatures enhance the antioxidant and antimicrobial properties under the effect of TGase.25 However, no studies have examined the effect of this approach on AGEs. Therefore, we investigated whether the non-enzymatic reaction (glycation) between the amino group and carbonyl group could be inhibited by the enzyme-mediated conjunction (glycosylation) between COS and Gln, thereby reducing the amount of AGEs in heated foods.
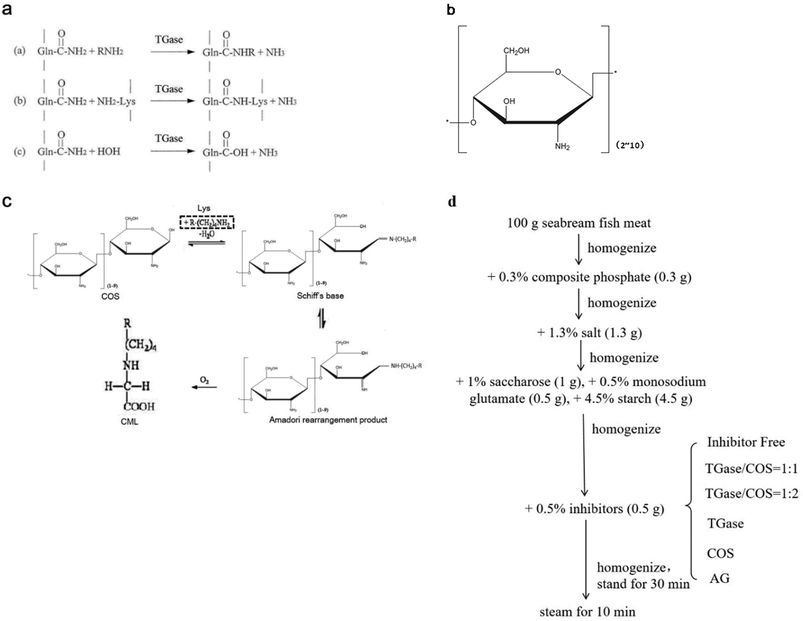 |
| Fig. 1 The introduction of TGase, COS, CML (COS reacts with Lys), and production process of SBFMS. a. The mechanism of action of TGase: (a) acyltransfer reaction, (b) crosslinking reaction, and (c) deamidation. b. Chemical structure of COS. c. Pathways to CML between COS and Lys in food systems (Lys is framed by a dotted line). d. The production process of SBFMS. | |
In the present study, we added different proportions of TGase and COS into the real food seabream fish meat sausage (SBFMS) to study the inhibition of AGEs. We measured the inhibitory effect of TGase and COS on the principal compounds of total fluorescent AGEs and CML, as TGase and COS have been recognized as safe food additives.18,23 This study can provide the fundamental basis for the food industry to produce safe and healthy heated fishery products with less harmful substances and better food properties.
2. Materials and methods
2.1. Materials and chemicals
AGEs (98%) and CML (98%) standard substances were obtained from Solarbio Technology Co., Ltd (Beijing, China) and Toronto Research Chemicals Inc. (Toronto, Canada), respectively. TGase (Ca2+-independent) produced from microorganisms was purchased from Pangbo Biological Engineering Co. (Nanning, China). COS was obtained from Bozhihuili Biological Technology Co., Ltd (Qingdao, China). D-(+)-GlcN hydrochloride, o-aminobenzoic acid, sodium cyanoborohydride, aminoguanidine (AG), phosphoric acid, and n-butylamine were obtained from Sigma Chemical Co. (Louis, USA). Methanol, acetonitrile, and tetrahydrofuran of HPLC grade were from Tedia Chemicals Inc. (Cincinnati, USA). Dichloromethane, trifluoroacetic anhydride (99%), and thionyl chloride (99.5%) of HPLC grade were purchased from ACS Chemicals Inc. (Houston, USA), J&K Scientific Inc. (Beijing, China), and Kemiou Chemicals Inc. (Tianjin, China), respectively. Seabream (Pagrosomus major) fish meat and condiments were obtained from Huifa Foods Co., Ltd (Zhucheng, China). Ultrapure water was produced from the Milli-Q system (Millipore, Bedford, USA). All other reagents were of analytical grade, unless mentioned otherwise.
2.2. Preparation of SBFMS
SBFMS was prepared as shown in Fig. 1d, in which AG was the positive control as a commonly effective inhibitor of AGEs.14 All samples were lyophilized and ground to fine powders using a high-speed blender (Joyoung Co., Ltd, Jinan, China). The lyophilized powders were stored at −18 °C until use.
2.3. Measurement of total fluorescent AGE content
The content of total fluorescent AGEs was measured by using a fluorescence spectrometer (Hitachi Corporation, Tokyo, Japan) according to our previous study.26
2.4. Measurement of CML content
The CML content of SBFMS was quantified by gas chromatography-mass spectrometry (Agilent Technologies Inc., Palo Alto, USA) according to our previous study.2
2.5. Determination of Lys content
The Lys content was measured according to the methods described by Hall et al. with slight modifications.27 The absorbance of the extracted solution was measured at 415 nm using a UV1101 ultraviolet spectrophotometer (Techcomp Ltd, Shanghai, China).
2.6. Measurement of fructosamine content
The content of fructosamine was determined using a nitroblue tetrazolium (NBT) assay, with slight modifications to the method described by Chompoo et al.28 The absorbance was measured at 530 nm by using a Multiscan MK3 microplate reader (Thermofisher Scientific Co., New York, USA).
2.7. Measurement of GlcN content
In order to estimate the amount of conjugated COS in the SBFMS, the content of GlcN, after removing non-crosslinked COS by dialysis, was measured using a high performance liquid chromatography system 1260 Series (HPLC) (Agilent Tech., Palo Alto, USA) according to the method described by Jiang and Zhao.29
2.8. Sodium dodecyl sulfate-polyacrylamide gel electrophoresis (SDS-PAGE) analysis
The SDS-PAGE analysis of glycoproteins was performed using slightly modified Lv et al.'s methods.30 The image of the gel was captured using a G4050 scanner (China Hewlett-Packard Co., Ltd, Beijing, China).
2.9. Matrix-assisted laser desorption/ionization time-of-flight mass spectrometry (MALDI-TOF/TOF-MS) analysis
It was difficult to determine whether COS was crosslinked with the proteins according to the result of SDS-PAGE analysis. In order to ascertain this, MALDI-TOF/TOF-MS analysis of glycoproteins was performed. After cutting the SDS-PAGE gel, the excised gel pieces (Table 1) containing glycoproteins were analyzed by MALDI-TOF/TOF-MS (Bruker Daltonics GmbH, Bremen, Germany), as described by Hrynets et al.31
Table 1 The names of the samples by gel cutting of SDS-PAGE. A, inhibitor free; B, TGase/COS = 1
:
1; C, TGase/COS = 1
:
2; D, TGase; E, COS; F, AG. An equal amount of glycoprotein (≈0.4 μg) was loaded in each lane
Names of samples |
Types of inhibitors |
Location of protein bands (kDa) |
Loaded protein content (μg) |
A |
Inhibitor free |
36.0 |
0.4025 |
B |
TGase/COS = 1 : 1 |
36.0 |
0.4450 |
C |
TGase/COS = 1 : 2 |
36.0 |
0.4025 |
D |
TGase |
36.0 |
0.4025 |
E |
COS |
36.0 |
0.4000 |
F |
AG |
36.0 |
0.4025 |
2.10. Statistical analysis
Our experiments were performed at least in duplicate with one-way analysis of variance (ANOVA) and statistical analyses were carried out using SPSS 19.0 software (SPSS Inc., Chicago, USA). The Duncan test was performed to test significant differences (p < 0.05) between test groups unless otherwise stated. All figures were prepared with Origin Pro8.5 software (OriginLab, Northampton, USA).
3. Results and discussion
3.1. Total fluorescent AGE content
The fluorescence intensity of total fluorescent AGEs could reflect their content, and the positive correlation between the AGE content and its fluorescence intensity was verified.32 As shown in Fig. 2a, the content of total fluorescent AGEs in SBFMS with TGase/COS = 1
:
1 was lower than that in the blank control (inhibitor free), although insignificant. The total fluorescent AGE contents in other groups, except in the positive control (SBFMS with AG), were higher than that in the blank control. We observed that using the TGase/COS ratio of 1
:
1 could reduce the content of total fluorescent AGEs in SBFMS, although the reduction effect was not as strong as the AG group. While considering the side effects of AG on human health, TGase/COS = 1
:
1 was preferred.
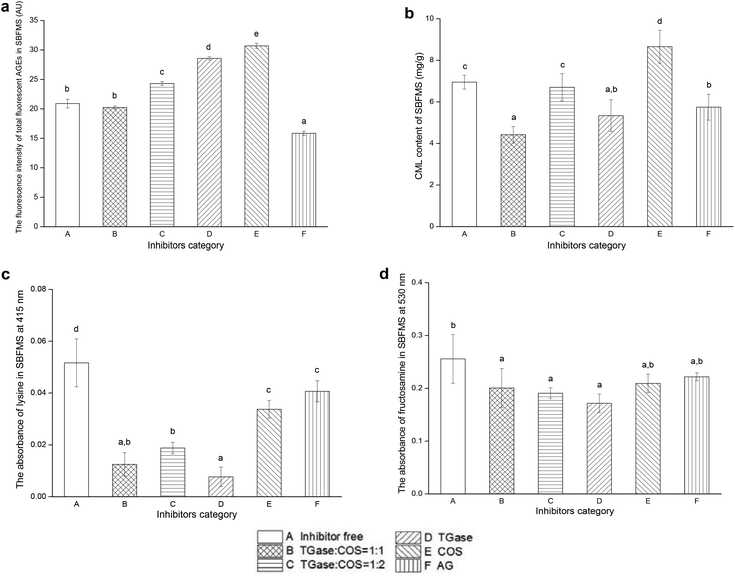 |
| Fig. 2 The fluorescence intensity of total fluorescent AGEs (a), content of CML (b), absorbance of lysine at 415 nm (c), and absorbance of fructosamine at 530 nm (d) in SBFMS with different inhibitors. A, inhibitor free; B, TGase/COS = 1 : 1; C, TGase/COS = 1 : 2; D, TGase; E, COS; F, AG. Fluorescence intensity was determined at 360 nm (excitation) and 475 nm (emission). Bars with different letters (a–e) differed significantly (p < 0.05). Analyses were conducted in duplicate. | |
3.2. CML content
Generally, CML is used as a marker of AGEs because of its largest number among AGE compounds.33 In our study, the content of CML decreased by 36.4% when the TGase/COS ratio was 1
:
1 (Fig. 2b), while the positive control (SBFMS with AG) showed a reduction by 17.4%. The CML content in SBFMS with TGase and COS was 23.3% lower and 24.5% higher than that in the blank control, respectively. The CML content of SBFMS with a TGase/COS ratio of 1
:
2 was reduced by 3.6%.
Of the six tested inhibitors, it was observed that TGase/COS = 1
:
1 inhibited both total fluorescent AGEs and CML, and drastically reduced the content of CML, compared to that of the positive control group. The inhibition rate of CML by TGase/COS = 1
:
1, the most abundant compound of AGEs, was two times higher than that of AG, which is a well-known anti-glycation drug owing to its property of scavenging dicarbonyl compounds.34 Although AG's inhibitory effect on total fluorescent AGEs was better than that when TGase/COS = 1
:
1, its adverse effects on human health cannot be ignored. Most of the studies on the inhibitors of AGEs had model food systems such as bovine serum albumin and one kind of reducing sugar. However, we chose real food, SBFMS, a complex food system showing more complex biochemical reactions as our experimental sample. This would be more convincing than model food systems. As mentioned previously, most of the substances that have been reported to inhibit the formation of AGEs are phenols, flavonoids, tannic acid, polyphenols, and other antioxidants.3,15,34 However, not all antioxidants could prevent glycation.35 Although the combined effect of TGase and COS was unrelated to antioxidation, it could effectively inhibit the formation of AGEs, especially CML. Furthermore, as common food additives, the TGase/COS ratio of 1
:
1 could not only reduce the harmful AGEs, but also improve food properties (such as emulsion stability, gelation properties, and thermal stability) and cause no negative effects on sensory properties.22,23 In order to figure out the inhibitory mechanism of the TGase/COS ratio of 1
:
1, we determined the principal chemical compounds including lysine, fructosamine, and GlcN.
3.3. Lys content
In order to detect the content of lysine in SBFMS and the effect of TGase in this inhibition, we analyzed lysine. As shown in Fig. 1c, Lys plays an important role in the formation of CML. The free amino group of the Lys side chain has been regarded as one of the major sites of glycation, inducing the formation of AGEs.36 The absorbance of the extracted solution at 415 nm is positively correlated with the content of Lys.27 As shown in Fig. 2c, the Lys contents of TGase added SBFMS were lower than those in SBFMS without TGase, and were negatively correlated with the concentration of TGase. The Lys contents of samples containing inhibitors (TGase/COS = 1
:
1, TGase/COS = 1
:
2, TGase, COS, AG) were significantly lower than that of the blank control (p < 0.05). These results indicated that TGase could effectively reduce the content of reactive Lys in food proteins, further inhibiting the formation of AGE compounds, particularly CML. On one hand, Gln could cross-link with Lys by the effect of TGase; on the other hand, Gln could cross-link with COS in the presence of TGase (the reason for inhibition will be discussed in section 3.7). The reduction of the Lys content in the presence of TGase/COS = 1
:
1 could be important to prevent the formation of CML. This mechanism has been verified by Ionescu et al. and Jiang and Zhao, who reported that TGase could catalyze the cross-linking of proteins in food by an acyl transfer reaction, and has been widely used to improve the functional properties of proteins in food.20,21 The content of lysine, however, does not correspond to the content of CML, because lysine could not only participate in the formation of CML, but it could also involve in the generation of CEL and other AGEs. Lysine, because of the existence of its amine in the side chain, is an active amino acid, and it can participate in many chemical reactions such as the crosslinking of proteins under the effect of TGase.37 Furthermore, there are many paths to generate CML, among which the Hodge path does not require the participation of lysine.38
3.4. Fructosamine content
Fructosamine, an unstable Amadori rearrangement product, is one of the products of the early stage of glycation products.33 Thus, the fructosamine content might reflect the inhibitory potential at the initiation stage of glycation. The absorbance of the extracted solution at 530 nm shows a positive correlation with the content of fructosamine.28 As shown in Fig. 2d, the absorbance values of these six groups of SBFMS were between 0.17 and 0.26, and the differences among groups B–F were not significant. Groups B–D showed significant differences compared with group A because of the addition of TGase in these groups. The ascending order of the content of fructosamine (TGase, TGase/COS = 1
:
2, TGase/COS = 1
:
1, COS, AG, inhibitor free) was similar to that of lysine (TGase, TGase/COS = 1
:
1, TGase/COS = 1
:
2, COS, AG, inhibitor free). It suggested that fructosamine was not the obvious inhibitory site of TGase and COS, because its levels did not differ significantly in TGase and COS added samples, but the addition of five types of inhibitors reduced the content of fructosamine. Similarly, Abdallah et al. reported that although catechins did not display any activity in the fructosamine assay, they showed inhibition of AGE formation, because their radical scavenging activity does not participate in the initiation of glycation.39 Consequently, we put forward that the inhibitory effect of the TGase/COS ratio of 1
:
1 on AGEs does not involve the production of fructosamine or the initiation of glycation.40
3.5. GlcN content
In order to determine the quantity of COS crosslinked with the peptide polymers of proteins in SBFMS, we performed the GlcN (the monomer of COS) analysis. As shown in Fig. 3, the peak of derivatized GlcN with a retention time in the range of 13 to 14 min was observed simultaneously in standard GlcN solution (Fig. 3a) and SBFMS (Fig. 3b). The content of GlcN in SBFMS was calculated according to the calibration curve of the derivatized GlcN peak area and concentration (Fig. 3c). The GlcN content in SBFMS containing the TGase/COS ratio of 1
:
1, TGase/COS ratio of 1
:
2, and COS was 0.081, 0.092, and 0.101 mg per 100 mg protein, respectively (Fig. 3d). GlcN was absent in other three groups, as no COS was added in them.
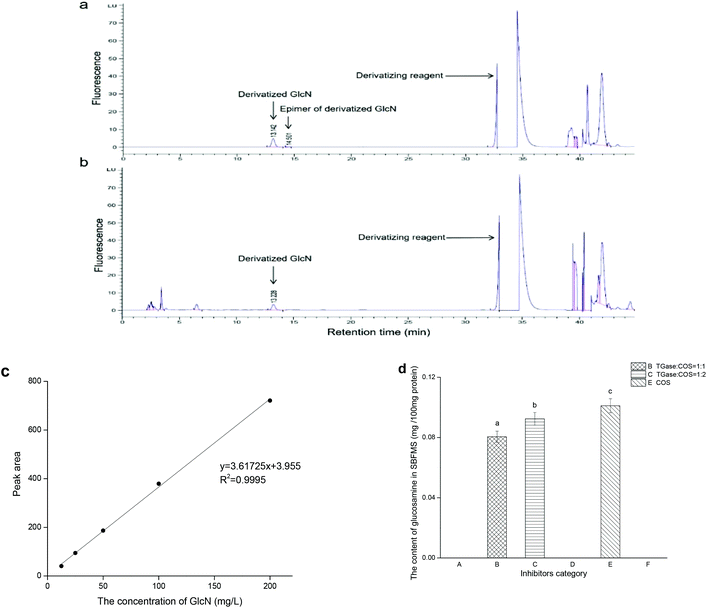 |
| Fig. 3 The detection results of GlcN. HPLC-fluorescence profiles of derivatized GlcN from standard GlcN solution (a) and SBFMS (b), excitation wavelength was 230 nm and emission wavelength was 425 nm, Eclipse Plus-C18 reversed phase column (250 mm × 4.6 mm, i.d. 5 μm; Agilent Tech., California, USA) with a flow rate of 1.0 mL min−1 at 25 °C. (c) Calibration curve of the derivatized GlcN peak area and concentration. (d) The content of GlcN in SBFMS with different inhibitors. A, inhibitor free; B, TGase/COS = 1 : 1; C, TGase/COS = 1 : 2; D, TGase; E, COS; F, AG. Bars with different letters (a–c) differed significantly (p < 0.05). Analyses were conducted in duplicate. | |
As shown in Fig. 3a, there were two peaks in the profile: one corresponding to derivatized GlcN and the other was its epimer peak. In the study of Jiang and Zhao, both peaks were included in GlcN measurement.29 However, we discarded the epimer peak because it could not be easily found in the profile of SBFMS (Fig. 3b). The correlation coefficient of the calibration curve of the derivatized GlcN peak area and concentration was higher than 0.999 (Fig. 3c), suggesting that this method could determine the content of GlcN accurately. As shown in Fig. 3d, the order of the GlcN content from low to high was TGase/COS of 1
:
1, TGase/COS of 1
:
2, and COS. No matter whether the SBFMS system contained TGase, the more COS was added, the more COS would be crosslinked with peptide polymers of proteins. The results indicated that a non-enzymatic reaction without TGase (glycation) and enzyme-mediated conjunction with TGase (glycosylation) occurred simultaneously, and there was a competitive relationship between them, in which the non-enzymatic reaction occupied the major position. Similarly, Hrynets et al. also claimed that glycation and glycosylation occurred concurrently in the system of GlcN, TGase, and natural actomyosin.31 The major position of glycation in our study was related to the indispensable steaming process, but the incubation pretreatment (room temperature, 30 min) before steaming contributed to glycosylation under the effect of TGase. Thus, glycation and glycosylation occurred simultaneously, while glycation showed an advantage in our research.
3.6. SDS-PAGE of glycoproteins in SBFMS
In order to detect the change of proteins in SBFMS with different inhibitors, glycoproteins extracted from SBFMS containing different inhibitors were analyzed by SDS-PAGE (Fig. 4). An equal amount of glycoproteins (≈0.4 μg) was loaded in each lane. In addition to the main band of the protein at ∼36 kDa, a new protein band at ∼70 kDa was visualized in the SBFMS containing TGase, and the intensities of the protein bands at about 36 kDa in lanes B, C, and D were lower than those in other samples.
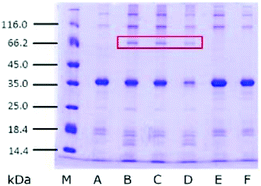 |
| Fig. 4 SDS-PAGE patterns of glycoprotein extracted from SBFMS with different inhibitors. M, molecular weight marker; A, inhibitor free; B, TGase/COS = 1 : 1; C, TGase/COS = 1 : 2; D, TGase; E, COS; F, AG. An equal amount of glycoprotein (≈0.4 μg) was loaded in each lane. | |
It could be seen from Fig. 4 that the main protein band was at about 36 kDa. We confirmed that this protein was tropomyosin, known as the major allergen in aquatic animals, based on previous reports.30 It is worth noting that the intensities of tropomyosin bands in the presence of TGase (lanes B, C, and D) were lower than those in the absence of TGase. Zhao et al. reported that the Maillard reaction could influence the structural and immunological properties of the major allergen in fish.41 Therefore, investigating the anti-allergenic property of TGase by reducing the tropomyosin content in aquatic products could be a new research direction.42,43 The new protein band at about 70 kDa was probably a consequence of the cross-linking among tropomyosin under the effect of TGase, which was supported by Song and Zhao.44 Based on the results of GlcN and SDS-PAGE, we believed that the modified protein was generated, which was conjugated to COS by glycosylation and glycation, and cross-linked among tropomyosin under the catalytic action of TGase. However, we could not ascertain the chemical bond between the protein and COS to distinguish glycosylation and glycation. Therefore, the cross-linking reaction type between the protein and COS (glycosylation or glycation), and the reason why the CML content was the lowest in SBFMS with the TGase/COS ratio of 1
:
1 are not clear through these results. So we performed MALDI-TOF/TOF-MS analysis.
3.7. MALDI-TOF/TOF-MS analysis of protein glycation/glycosylation modification in SBFMS
MALDI-TOF/TOF-MS was performed to check whether the glycosylation and glycation reactions occurred between COS and proteins under the effect of TGase. We set the molecular weight of COS cross-linked with proteins as n (2–10) times 161 (the molecular weight of GlcN), because we are not sure of its degree of polymerization. For example, if the degree of polymerization of COS is 5, its molecular weight is 823 Da (Mw = 161 × n + 18). COS could be cross-linked with γ-carboxyamide of Gln (Q) under the catalytic action (glycosylation) of TGase owing to its primary amine. In addition, the hydroxyl group of its end carbon could react with the amino groups of Lys residues (Lys, K) by glycation of the peptide. When the glycosylation between Gln and COS occurred, the mass of the peptide increased by 806 Da [(peptide + COS) − NH3]. When the glycation occurred between Lys and COS, the mass of the peptide increased by 805 Da [(peptide + COS) − H2O]. What needs to be specifically proposed is that ammonia liberation during glycation might affect the molecular weight.45 Hence, in order to distinguish the molecular weight difference between glycosylation and glycation, the molecular weight change of the glycation was assessed in two ways: +805 Da [(peptide + COS) − H2O] and +788 Da [(peptide + COS) − (H2O + NH3)].31
We analyzed the mass spectra using both the change in molecular mass and the sites of conjugation (K or Q), finding that glycation and glycosylation occurred at the same time although the signal was not so strong (Fig. 5a and b). As shown in Fig. 5, glycation occurred in SBFMS containing COS (Fig. 5a–c), while glycation and glycosylation occurred simultaneously in SBFMS containing COS and TGase (Fig. 5a and b). As shown in Fig. 6, the glycation of Lys (the fourth K) in the peptide sequence K.KKMQMLK.L was detected in SBFMS with COS, but there was only glycosylation of Gln (Q) instead of glycation of Lys (the fourth K) in SBFMS with TGase and COS. After a thorough literature search, we hypothesized that COS was cross-linked with Gln under the catalytic action of TGase, resulting in steric hindrance that blocks the access to glycation of nearby lysine residues.46 This was also the reason why the CML content of SBFMS was reduced by the addition of TGase/COS in a 1
:
1 ratio. While the CML content of SBFMS was not reduced by the addition of TGase/COS in a 1
:
2 ratio because there was more COS in the 1
:
2 system participating in the glycation and not in the glycosylation. In contrast, Hrynets et al. showed that the steric hindrance in glycation made it less accessible for TGase in their study of GlcN, natural actomyosin, and TGase.31 This may be because we used a different amino sugar, COS, which has much larger molecular weight than that of GlcN. Furthermore, the pretreatment step of incubation for 30 min at room temperature (about 25 °C) before steaming contributed to the catalytic action of TGase.
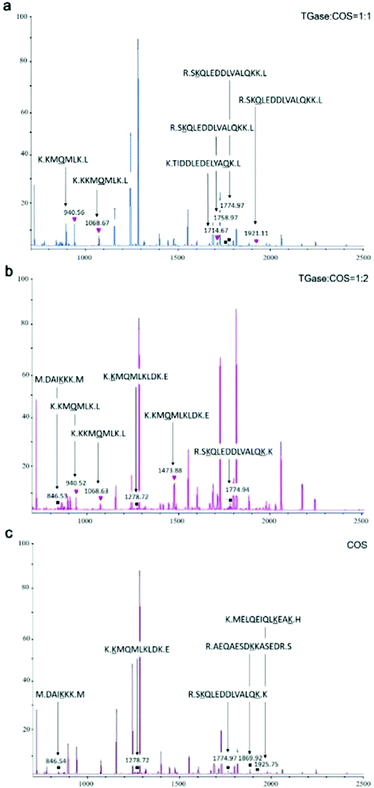 |
| Fig. 5 MALDI-TOF/TOF-MS analyses of protein glycation/glycosylation modifications: (a) TGase/COS = 1 : 1; (b) TGase/COS = 1 : 2; and (c) COS. The black squares indicate a non-enzymatic glycation reaction of COS and Lys (K), and the red triangles indicate an enzymatic glycosylation of COS and Gln (Q). The under horizontal amino acids (K/Q) on behalf of the non-enzymatic glycation reaction site (K) or the enzymatic glycosylation reaction site (Q). The small black dot in amino acid sequence represents the enzyme cutting site. A, alanine; D, aspartic acid; E, glutamic acid; H, histidine; I, isoleucine; K, lysine; L, leucine; M, methionine; Q, glutamine; R, arginine; S, serine; T, threonine; V, valine; Y, tyrosine. Analyses were conducted in duplicate. | |
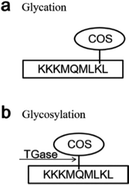 |
| Fig. 6 The glycation and glycosylation of a main short peptide (amino acid sequence KKKMQMLKL) in SBFMS. Glycation between lysine (K) with COS (a) and glycosylation between glutamine (Q) with COS under the effect of TGase (b). K, lysine; L, leucine; M, methionine; Q, glutamine. | |
It should be noted that this study examined the content of GlcN, the change of the molecular mass, and the reaction site (K or Q) to explain the reduction of the CML content of SBFMS on addition of TGase/COS in a ratio of 1
:
1. In terms of SBFMS, which is a complex food system, there is a need for a deeper and more thorough biochemical analysis to clarify the reasons for AGE reduction.
4. Conclusions
In summary, we have shown that the TGase/COS ratio of 1
:
1 could inhibit the formation of AGEs in SBFMS, especially showing a remarkable decrease in CML and the inhibition rate was two times higher than that of AG, used as a positive control. The addition of TGase could protect Lys from chemical modification by cross-linking of proteins. Additionally, enzymatic glycosylation of Gln, induced by TGase and COS, resulted in steric hindrance which inhibited glycation of nearby lysine residues. Enzyme-mediated glycosylation could inhibit the formation of AGEs through avoiding glycation. As food additives, TGase and COS are safer and potential glycation inhibitors compared with other antioxidants.
Conflicts of interest
There are no conflicts to declare.
Acknowledgements
We are grateful for the help from the National Institute of Metrology of China for MALDI-TOF/TOF-MS analysis and the help from Editage (Cactus Communications Inc.) to improve the language. This work was supported by the National Natural Science Foundation of China (Project no. 31571941).
References
- M. W. Poulsen, R. V. Hedegaard, J. M. Andersen, B. De Courten, S. Bügel, J. Nielsen, L. H. Skibsted and L. O. Dragsted, Advanced glycation endproducts in food and their effects on health, Food Chem. Toxicol., 2013, 60, 10–37 CrossRef CAS PubMed.
- J. Wang, S.-L. Tian, W.-H. Sun, H. Lin and Z.-X. Li, Determination of Nε-carboxymethyl-lysine content in muscle tissues of turbot by gas chromatography-mass spectrometry, Chin. J. Anal. Chem., 2015, 43, 1187–1192 CAS.
- C.-H. Wu, S.-M. Huang, J.-A. Lin and G.-C. Yen, Inhibition of advanced glycation endproduct formation by foodstuffs, Food Funct., 2011, 2, 224–234 CAS.
- H. T. Nguyen, H. van der Fels-Klerx and M. A. van Boekel, Kinetics of Nε-(carboxymethyl) lysine formation in aqueous model systems of sugars and casein, Food Chem., 2016, 192, 125–133 CrossRef CAS PubMed.
- T. Henle, Protein-bound advanced glycation endproducts (AGEs) as bioactive amino acid derivatives in foods, Amino Acids, 2005, 29, 313–322 CrossRef CAS PubMed.
- K. Hecht, Food Safety Chemistry: Toxicant Occurrence, Analysis and Mitigation, LWT–Food Sci. Technol., 2015, 63, 1351–1353 CrossRef CAS.
- J. R. Kizer, D. Benkeser, A. M. Arnold, J. H. Ix, K. J. Mukamal, L. Djousse, R. P. Tracy, D. S. Siscovick, B. M. Psaty and S. J. Zieman, Advanced glycation/glycoxidation endproduct carboxymethyl-lysine and incidence of coronary heart disease and stroke in older adults, Atherosclerosis, 2014, 235, 116–121 CrossRef CAS PubMed.
- C. M. Rebholz, B. C. Astor, M. E. Grams, M. K. Halushka, M. Lazo, R. C. Hoogeveen, C. M. Ballantyne, J. Coresh and E. Selvin, Association of plasma levels of soluble receptor for advanced glycation end products and risk of kidney disease: the atherosclerosis risk in communities study, Nephrol., Dial., Transplant., 2015, 30, 77–83 CrossRef CAS PubMed.
- W.-H. Hsu, S.-S. Lu, B.-H. Lee, Y.-W. Hus and T.-M. Pan, Monacolin K and monascin attenuated pancreas impairment and hyperglycemia induced by advanced glycation endproducts in BALB/c mice, Food Funct., 2013, 4, 1742–1750 CAS.
- B. de Courten, M. P. de Courten, G. Soldatos, S. L. Dougherty, N. Straznicky, M. Schlaich, K. C. Sourris, V. Chand, J. L. Scheijen, B. A. Kingwell, M. E. Cooper, C. G. Schalkwijk, K. Z. Walker and J. M. Forbes, Diet low in advanced glycation end products increases insulin sensitivity in healthy overweight individuals: a double-blind, randomized, crossover trial, Am. J. Clin. Nutr., 2016, 103, 1426–1433 CrossRef CAS PubMed.
- Q. Wu, S. Li, X. Li, Y. Sui, Y. Yang, L. Dong, B. Xie and Z. Sun, Inhibition of advanced glycation endproduct formation by lotus seedpod oligomeric procyanidins through RAGE-MAPK signaling and NF-κB activation in high-fat-diet rats, J. Agric. Food Chem., 2015, 63, 6989–6998 CrossRef CAS PubMed.
- H. Sugawa, R. Ohno, J. Shirakawa, A. Nakajima, A. Kanagawa, T. Hirata, T. Ikeda, N. Moroishi, M. Nagai and R. Nagai, Eucommia ulmoides extracts prevent the formation of advanced glycation end products, Food Funct., 2016, 7, 2566–2573 CAS.
- Z. Sun, X. Peng, J. Liu, K. Fan, M. Wang and F. Chen, Inhibitory effects of microalgal extracts on the formation of advanced glycation endproducts (AGEs), Food Chem., 2010, 120, 261–267 CrossRef CAS.
- W. Liu, Z. Wei, H. Ma, A. Cai, Y. Liu, J. Sun, N. A. DaSilva, S. L. Johnson, L. J. Kirschenbaum, B. P. Cho, J. A. Dain, D. C. Rowley, Z. A. Shaikh and N. P. Seeram, Anti-glycation and anti-oxidative effects of a phenolic-enriched maple syrup extract and its protective effects on normal human colon cells, Food Funct., 2017, 8, 757–766 CAS.
- G. Chen, R. L. Madl and J. S. Smith, Inhibition of advanced glycation endproducts in cooked beef patties by cereal bran addition, Food Control, 2017, 73, 847–853 CrossRef CAS.
- X. Zhang, F. Chen and M. Wang, Antioxidant and antiglycation activity of selected dietary polyphenols in a cookie model, J. Agric. Food Chem., 2014, 62, 1643–1648 CrossRef CAS PubMed.
- C. Srey, G. L. J. Hull, L. Connolly, C. T. Elliott, M. D. Del Castillo and J. M. Ames, Effect of inhibitor compounds on Nε-(carboxymethyl)lysine (CML) and Nε-(carboxyethyl)lysine (CEL) formation in model foods, J. Agric. Food Chem., 2010, 58, 12036–12041 CrossRef CAS PubMed.
- A. L. C. Gaspar and S. P. de Góes-Favoni, Action of microbial transglutaminase (MTGase) in the modification of food proteins: A review, Food Chem., 2015, 171, 315–322 CrossRef CAS PubMed.
- B. Zeeb, L. Fischer and J. Weiss, Stabilization of food dispersions by enzymes, Food Funct., 2014, 5, 198–213 CAS.
- A. Ionescu, I. Aprodu, A. Darabă and L. Porneală, The effects of transglutaminase on the functional properties of the myofibrillar protein concentrate obtained from beef heart, Meat Sci., 2008, 79, 278–284 CrossRef CAS PubMed.
- S. Jiang and X. Zhao, Transglutaminase-induced cross-linking and glucosamine conjugation in soybean protein isolates and its impacts on some functional properties of the products, Eur. Food Res. Technol., 2010, 231, 679–689 CrossRef CAS.
- Y. Zhang and X. Zhao, Study on the functional properties of soybean protein isolate cross-linked with gelatin by microbial transglutaminase, Int. J. Food Prop., 2013, 16, 1257–1270 CrossRef CAS.
- P. Zou, X. Yang, J. Wang, Y. Li, H. Yu, Y. Zhang and G. Liu, Advances in characterisation and biological activities of chitosan and chitosan oligosaccharides, Food Chem., 2016, 190, 1174–1181 CrossRef CAS PubMed.
- W. Jiang, Z. Zhou, D. Wang, X. Zhou, R. Tao, Y. Yang, Y. Shi, G. Zhang, D. Wang and Z. Zhou, Transglutaminase catalyzed hydrolyzed wheat gliadin grafted with chitosan oligosaccharide and its characterization, Carbohydr. Polym., 2016, 153, 105–114 CrossRef CAS PubMed.
- D. Gottardi, P. K. Hong, M. Ndagijimana and M. Betti, Conjugation of gluten hydrolysates with glucosamine at mild temperatures enhances antioxidant and antimicrobial properties, LWT–Food Sci. Technol., 2014, 57, 181–187 CrossRef CAS.
- J. Wang, Z. Li, R. T. Pavase, H. Lin, L. Zou, J. Wen and L. Lv, Advanced glycation endproducts in 35 types of seafood products consumed in eastern China, J. Ocean Univ. China, 2016, 15, 690–696 CrossRef CAS.
- R. J. Hall, N. Trinder and D. I. Givens, Observations on the use of 2,4,6-trinitrobenzenesulphonic acid for the determination of available lysine in animal protein concentrates, Analyst, 1973, 98, 673–686 RSC.
- J. Chompoo, A. Upadhyay, W. Kishimoto, T. Makise and S. Tawata, Advanced glycation end products inhibitors from alpinia zerumbet rhizomes, Food Chem., 2011, 129, 709–715 CrossRef CAS PubMed.
- S. Jiang and X. Zhao, Cross-linking and glucosamine conjugation of casein by transglutaminase and the emulsifying property and digestibility, Int. J. Food Prop., 2012, 15, 1286–1299 CrossRef CAS.
- L. Lv, H. Lin, Z. Li, Y. Song, H. Lin and A. Wang, Identification of oxidative modification of shrimp (Metapenaeus ensis) tropomyosin induced by malonaldehyde, Eur. Food Res. Technol., 2014, 239, 847–855 CrossRef CAS.
- Y. Hrynets, M. Ndagijimana and M. Betti, Transglutaminase-catalyzed glycosylation of natural actomyosin (NAM) using glucosamine as amine donor: Functionality
and gel microstructure, Food Hydrocolloids, 2014, 36, 26–36 CrossRef CAS.
- M. M. Joglekar, S. N. Panaskar and A. U. Arvindekar, Inhibition of advanced glycation end product formation by cymene - A common food constituent, J. Funct. Foods, 2014, 6, 107–115 CrossRef CAS.
- J. M. Ames, Determination of Nε-(carboxymethyl)lysine in foods and related systems, Ann. N. Y. Acad. Sci., 2008, 1126, 20–24 CrossRef CAS PubMed.
- S. Ho, P. Chang, H. Tong and P. Yu, Inhibition of fluorescent advanced glycation end-products and N-carboxymethyllysine formation by several floral herbal infusions, Int. J. Food Prop., 2014, 17, 617–628 CrossRef CAS.
- C. S. Harris, A. Cuerrier, E. Lamont, P. S. Haddad, J. T. Arnason, S. A. L. Bennett and T. Johns, Investigating wild berries as a dietary approach to reducing the formation of advanced glycation endproducts: chemical correlates of in vitro antiglycation activity, Plants Food Hum. Nutr., 2014, 69, 71–77 CrossRef CAS PubMed.
- S. Awasthi and N. T. Saraswathi, Sinigrin, a major glucosinolate from cruciferous vegetables restrains non-enzymatic glycation of albumin, Int. J. Biol. Macromol., 2016, 83, 410–415 CrossRef CAS PubMed.
- B. Li, F. Zhou, K. Huang, Y. Wang, S. Mei, Y. Zhou and T. Jing, Environmentally friendly chitosan/ PEI-grafted magnetic gelatin for the highly effective removal of heavy metals from drinking water, Sci. Rep., 2017, 7, 43082 CrossRef CAS PubMed.
- J. E. Hodge, Chemistry of browning reactions in model systems, J. Agric. Food Chem., 1953, 1, 928–943 CrossRef CAS.
- H. M. Abdallah, H. El-Bassossy, G. A. Mohamed, A. M. El-Halawany, K. Z. Alshali and Z. M. Banjar, Phenolics from garcinia mangostana inhibit advanced glycation endproducts formation: effect on amadori products, cross-linked structures and protein thiols, Molecules, 2016, 21, 251 CrossRef PubMed.
- S. Seo and S. Karboune, Investigation of the use of maillard reaction inhibitors for the production of patatin-carbohydrate conjugates, J. Agric. Food Chem., 2014, 62, 12235–12243 CrossRef CAS PubMed.
- Y.-J. Zhao, Q.-F. Cai, T.-C. Jin, L.-J. Zhang, D.-X. Fei, G.-M. Liu and M.-J. Cao, Effect of Maillard reaction on the structural and immunological properties of recombinant silver carp parvalbumin, LWT–Food Sci. Technol., 2017, 75, 25–33 CrossRef CAS.
- N. G. Heredia-Sandoval, A. R. Islas-Rubio, F. Cabrera-Chávez and A. M. Calderón de la Barca, Effect of Maillard reaction on the structural and immunological properties of recombinant silver carp parvalbumin, Food Funct., 2014, 5, 1813–1818 CAS.
- Z. Wu, J. Lian, R. Zhao, K. Li, X. Li, A. Yang, P. Tong and H. Chen, Ara h 2 cross-linking catalyzed by MTGase decreases its allergenicity, Food Funct., 2017, 8, 1195–1203 CAS.
- C. Song and X. Zhao, Structure and property modification of an oligochitosan-glycosylated and crosslinked soybean protein generated by microbial transglutaminase, Food Chem., 2014, 163, 114–119 CrossRef CAS PubMed.
- Y. Hrynets, M. Ndagijimana and M. Betti, Non-enzymatic glycation of natural actomyosin (NAM) with glucosamine in a liquid system at moderate temperatures, Food Chem., 2013, 139, 1062–1072 CrossRef CAS PubMed.
- W. Liu, H. Ma, L. Frost, T. Yuan, J. A. Dain and N. P. Seeram, Pomegranate phenolics inhibit formation of advanced glycation endproducts by scavenging reactive carbonyl species, Food Funct., 2014, 5, 2996–3004 CAS.
|
This journal is © The Royal Society of Chemistry 2018 |