DOI:
10.1039/C7TX00083A
(Paper)
Toxicol. Res., 2017,
6, 554-560
A comparative study of hydrophilic phosphine hexanuclear rhenium cluster complexes’ toxicity
Received
24th March 2017
, Accepted 16th May 2017
First published on 17th May 2017
Abstract
The octahedral rhenium cluster compound Na2H8[{Re6Se8}(P(C2H4CONH2)(C2H4COO)2)6] has recently emerged as a very promising X-ray contrast agent for biomedical applications. However, the synthesis of this compound is rather challenging due to the difficulty in controlling the hydrolysis of the initial P(C2H4CN)3 ligand during the reaction process. Therefore, in this report we compare the in vitro and in vivo toxicity of Na2H8[{Re6Se8}(P(C2H4CONH2)(C2H4COO)2)6] with those of related compounds featuring the fully hydrolysed form of the phosphine ligand, namely Na2H14[{Re6Q8}(P(C2H4COO)3)6] (Q = S or Se). Our results demonstrate that the cytotoxicity and acute in vivo toxicity of the complex Na2H8[{Re6Se8}(P(C2H4CONH2)(C2H4COO)2)6] solutions were considerably lower than those of compounds with the fully hydrolysed ligand P(C2H4COOH)3. Such behavior can be explained by the higher osmolality of Na2H14[{Re6Q8}(P(C2H4COO)3)6] versus Na2H8[{Re6Se8}(P(C2H4CONH2)(C2H4COO)2)6].
Introduction
Recent studies indicate that hexanuclear rhenium cluster complexes with the general formula [{Re6Q8}L6] (where Q is S, Se, or Te and Ls are some organic or inorganic apical ligands) have high potential for therapeutic and diagnostic applications in different fields of biology and medicine due to their luminescence, photosensitising and antitumor properties as well as due to highly efficient absorption of X-rays.1–13 This set of outstanding physical properties led to intensive studies of the biological properties of rhenium octahedral clusters. As an example, we have recently developed the cluster complex Na2H8[{Re6Se8}(P(C2H4CONH2)(C2H4COO)2)6] that demonstrated high radiodensity and high water solubility.9 Importantly, our in vitro studies have shown that this compound has low cytotoxicity as it does not internalise into cells. In vivo studies have further confirmed low acute toxicity and the rapid elimination of the compound from the body through the urinary tract after intravenous injection.9,11 This compound has therefore excellent potential for application as an X-ray contrast agent.
This compound is synthesised under hydrothermal conditions from tris-(2-cyanoethyl)phosphine (P(C2H4CN)3) and Na4[{Re6Se8}(OH)6], upon which the organic ligand precursor undergoes hydrolysis. Unfortunately, under these operating conditions, it is difficult to control the hydrolysis of P(C2H4CN)3 and reproducibly obtain only two of three nitrile groups for each ligand being hydrolysed to form a carboxylate and one to form an amide. However, we have noticed that a similar hydrothermal reaction between [{Re6Q8}(OH)6]4− (Q = S or Se) and P(C2H4COOH)3 carried out in aqueous solutions led to the formation of the corresponding rhenium cluster complexes having tris-(2-carboxyethyl)phosphines as ligands – Na2H14[{Re6Q8}(P(C2H4COO)3)6]. Moreover, these compounds can also be obtained directly from P(C2H4COOH)·HCl and Na4[{Re6Q8}(OH)6] with high purity and good yield.14 Therefore, in this report we compare the biological properties of compounds Na2H14[{Re6Q8}(P(C2H4COO)3)6] (Q = S (1) or Se (2)) in vitro and in vivo with those of closely related Na2H8[{Re6Se8}(P(C2H4CONH2)(C2H4COO)2)6] (3) in order to evaluate what effect (if any) the level of hydrolysis and the cluster core composition (i.e. {Re6S8}2+vs. {Re6Se8}2+) has on cellular internalisation and toxicity, and acute toxicity.
Results
The critical step in the evaluation of the biological properties of compounds (1), (2) and (3) is the determination of their cytotoxicity. The effect of the cluster complexes on the metabolic activity of the cells was determined using the MTT colorimetric assay. We used three different cell lines as models to evaluate the toxic effect of these compounds: two cancer cell lines – human larynx carcinoma (Hep-2) and human epithelioid cervix carcinoma cell line (HeLa) – and a normal cell line – peritoneal macrophages (MPh). The Hep-2 and HeLa cells were chosen since these cell lines have already been used for the evaluation of the cytotoxicity of several hexanuclear metal clusters and cluster containing materials8–11,15–18 and therefore the results obtained allow for the comparison of cytotoxicity between different cluster based materials. Macrophages are cells of the mononuclear phagocytic system responsible for the clearance of pathogens and chemical compounds by phagocytosis and pinocytosis and they are also involved in inflammatory reactions.19,20 Finding a non-toxic in vivo bioimaging agent for macrophages could provide researchers with new tools to advance the evaluation of disease (e.g. cardiovascular disease, in particular ischemia, arthritis and other inflammation processes) and therapies.21,22
The results obtained by the MTT test in the concentration range 0.0056–5.76 mM are presented in Fig. 1, while values of the half maximal inhibitory concentration (IC50) are summarised in Table 1. The data show that compound (2) has the highest cytotoxic effect, while compound (3) has the lowest cytotoxic effect on all three model cell lines. The MTT assay also demonstrates that the peritoneal macrophages are noticeably more sensitive to (1) and (2) than HeLa and Hep-2 cells. Finally, all three studied cluster complexes showed no suppressive effect on the cell viability at concentrations from 0.0056 to 0.18 mM.
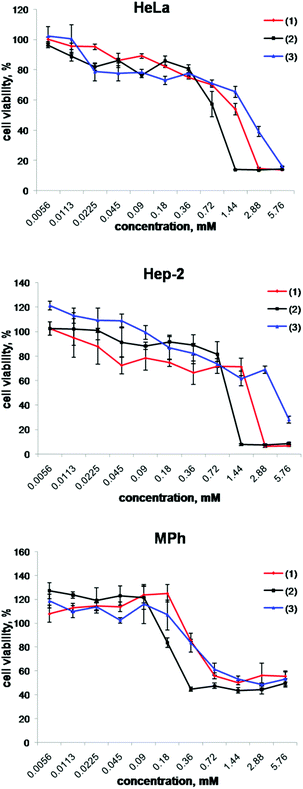 |
| Fig. 1 Cell viability response of HeLa, Hep-2 and MPh cells using the MTT assay for (1), (2) and (3). | |
Table 1 The IC50 of (1), (2) and (3) on the Hep-2, HeLa cells and peritoneal macrophages determined by the MTT assay
Compound |
IC50 values, mM |
MPh |
HeLa |
Hep-2 |
(1) |
1.27 ± 0.03 |
1.42 ± 0.07 |
1.80 ± 0.64 |
(2) |
0.36 ± 0.06 |
0.82 ± 0.03 |
1.07 ± 0.08 |
(3) |
1.72 ± 0.58 |
1.66 ± 0.14 |
5.60 ± 0.17 |
Since octahedral rhenium cluster complexes demonstrate luminescence in the red region, it is easy to monitor the cellular internalisation of these compounds using confocal microscopy. Indeed, it has already been shown before that compound (3) does not enter Hep-2 cells.11 Here we have undertaken similar studies on Hep-2, HeLa and MPh cell lines to evaluate whether there is any difference in both their internalisation in healthy and cancerous cells and between cluster complexes with different cores and apical ligands. Fig. 2 shows the representative confocal microscopy images of the cells incubated with 0.18 mM solutions of (1)–(3) for 24 hours. These images show that for both cancerous cell lines, there was no uptake of any of the studied compounds (Fig. 2G–R), while there is clear evidence of intense red photoluminescence located in the cytoplasm of the macrophages (Fig. 2A–F).
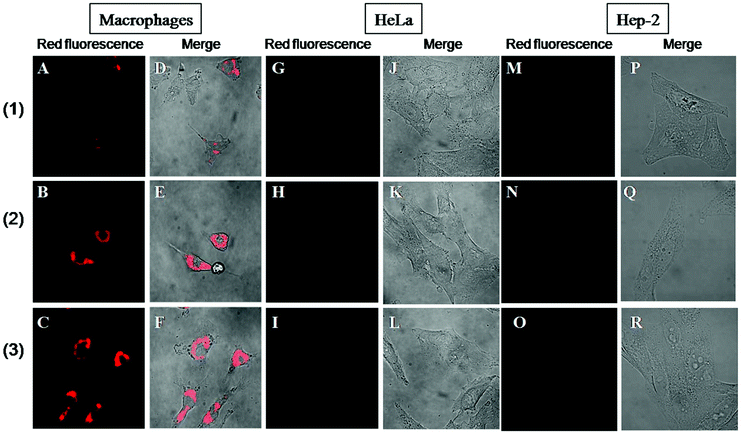 |
| Fig. 2 Confocal fluorescence microscopy of peritoneal macrophages (A–F), HeLa (G–L) and Hep-2 (M–R) after incubation with (1), (2) and (3) for 24 hours. Red fluorescence and merged (phase contrast and fluorescence) images. | |
Acute toxicity studies were undertaken on Balb/C mice, i.e. the same mice strain that was used for the acute toxicity studies of compound (3) before. The animals were randomly split into 9 groups with 10 mice in each group and treated intravenously using clusters (1) and (2) at doses 50, 150, 250 and 350 mg (Re) per kg or sham-injected with physiological saline. The survival rate of the mice after the treatment (Fig. 3) shows no evidence of toxicity for doses up to 150 mg (Re) per kg of the complexes (1) or (2). Within the groups that were treated with 250 mg (Re) per kg of (1) and (2), 6 and 4 mice, respectively, died within the first day. 350 mg (Re) per kg of both clusters was the lethal dose for all ten mice in the group. Finally, we have not observed any changes in the behavior of any of the animals that survived the treatment in any of the groups.
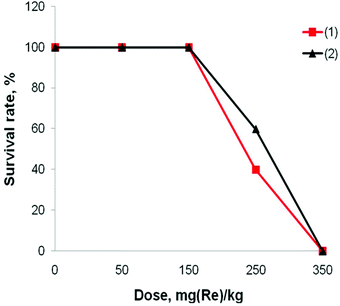 |
| Fig. 3 Survival rate of mice injected intravenously with 0.1 mL of the cluster complexes (1) or (2) at different doses (n = 10). | |
The median lethal dose (LD50) was determined to be 256 ± 25 mg (Re) per kg and 232 ± 24 mg (Re) per kg for the complexes (1) and (2), respectively. The LD50 for complex (3) was earlier found to be 4.67 ± 0.69 g kg−1,11 which corresponds to 1.59 ± 0.23 g (Re) per kg. Thus, from all three compounds, complex (3) is the least toxic for both in vitro and in vivo.
One of the factors that can significantly affect the acute toxicity of such closely related compounds may be the osmolality of their aqueous solutions. Therefore, we measured the osmolality of the solutions of (1) and (2) in the same concentrations that were used for treatment of mice (10, 30, 50, 70 mg (Re) per mL) and compared the results with those of complex (3) (Table 2).
Table 2 The osmolality of aqueous solutions of the cluster complexes (1), (2) and (3) at different concentrations
Compound |
C, mg (Re) per mL |
C, mM |
Osmolality, mOsm kg−1 |
(1) |
70 |
62.7 |
580 ± 1 |
50 |
44.8 |
398 ± 2 |
30 |
26.9 |
257 ± 4 |
10 |
9.0 |
96 ± 1 |
(2) |
70 |
62.7 |
613 ± 5 |
50 |
44.8 |
425 ± 2 |
30 |
26.9 |
255 ± 1 |
10 |
9.0 |
99 ± 1 |
(3) |
70 |
62.7 |
279 ± 2 |
50 |
44.8 |
198 ± 1 |
30 |
26.9 |
122 ± 1 |
10 |
9.0 |
51 ± 1 |
The solutions of the cluster complex (3) at all measured concentrations showed osmolality lower than that of serum (285 ± 10 mOsm kg−1). However, the solutions of the cluster complexes (1) and (2) possessed an acceptable osmolality only at the concentrations of 10 and 30 mg (Re) per mL. At concentrations of 50 and 70 mg (Re) per mL the osmolality of the solutions was higher than the osmolality of serum.
Discussion
Compounds Na2H14[{Re6Q8}(P(C2H4COO)3)6], where Q = S (1) and Se (2) are chemical derivatives of the compound Na2H8[{Re6Se8}(P(C2H4CONH2)(C2H4COO)2)6] (3) that was earlier proposed as a potentially good X-ray contrast agent. Specifically, there are two major differences between compounds (1), (2) and (3): (i) the overall charge of the cluster anions of (1) and (2) is significantly higher than that of (3) due to the chemical composition and charge of the organic ligand, i.e. three anion P(C2H4COO)33− rather than two anion P(C2H4CONH2)(C2H4COO)22− and (ii) the content of the cluster core in (1) is different from (2) and (3). To evaluate the effect of these differences in chemical composition on the biological properties of the cluster complexes, we have studied cellular toxicity and cellular internalisation using three different cell lines and acute toxicity in mice.
The results of the MTT assay have shown that compound (3) is the least cytotoxic for both the cancerous cell lines HeLa and Hep-2 cells and the healthy cell line MPh, while confocal microscopy has revealed that HeLa and Hep-2 cells do not take up any of the studied cluster complexes, while intense red luminescence located in the cytoplasm was observed from macrophages treated with the clusters. Thus, confocal microscopy imaging confirms the uptake of the clusters by MPh. The lack of uptake of the metal cluster by cancer cells is easily explained by the high negative charge of the cluster anion that prohibits cellular internalisation due to repulsive force with the cellular membrane. On the other hand it was already shown that macrophages are efficient in the uptake of both positively and negatively charged particles.23,24 Macrophages are cells of the mononuclear phagocytic system, which are distributed extensively in the tissue and play an important anti-inflammatory role. Cellular inflammation is one of the major symptoms of many developing pathologies including atherosclerosis, type 2 diabetes, Alzheimer's disease, cancer and other illnesses.25–28 One of the main approaches currently used for the detection and imaging of inflammation is based on the CT visualisation of hard tissue. X-ray imaging based on the use of radiopaque agents accumulated selectively in macrophages was recently offered as an alternative method for the detection of cellular inflammation in soft tissues.29–33 The ability of (1), (2) and (3) to easily accumulate in macrophages makes these moieties potentially good agents for the visualisation of cellular inflammation.
According to our in vivo toxicity studies on Balb/C mice, complex (3) is considerably less toxic than (1) and (2). Despite this, all these compounds demonstrate similar cellular internalisation patterns and the difference in their cellular toxicity is not significant. One of the possible explanations of the noticeably increased acute toxicity of (1) and (2) is the difference in the osmolality of the cluster solutions caused by a significant increase of the cluster anion charge, which consequently leads to a higher number of ions in a solution. Indeed, variations of plasma osmolality may interfere with the cell membrane polarisation or function and induce cell shape changes that may also modify the behavior of blood cells.34,35 Moreover, an intravenous injection of a hyperosmolar solution may produce significant changes in the acid–base equilibrium and the electrolyte concentrations,36 as well as increase the viscosity of the blood, disrupt the hemodynamics and induce thrombosis. The measured osmolality of the solutions (1) and (2) in the concentrations used for the treatment of mice was found to be about two-fold higher than that of the equivalent solutions of (3) and, apparently such an increase in osmolality was enough to increase the acute intravenous toxicity (i.e. LD50 values) of the cluster complexes (1) and (2) by the factor of ∼6 in comparison with complex (3).
Experimental
Materials and methods
Neutral aquahydroxo complexes [{Re6Q8}(H2O)4(OH)2] (Q = S or Se) were prepared according to a literature procedure.37 Na4[{Re6Q8}(OH)6] was obtained by the reaction of the corresponding complex [{Re6Q8}(H2O)4(OH)2] with an aqueous solution of NaOH followed by precipitation of Na4[{Re6Q8}(OH)6] with an excess of ethanol.9 Na2H8[{Re6Se8}(P(C2H4CONH2)(C2H4COO)2)6] (3) was synthesised by the hydrothermal reaction between Na4[{Re6Se8}(OH)6] and P(C2H4CN)3 (130 °C, 48 h) and purified by the method described before.9,11 Na2H14[{Re6Q8}(P(C2H4COO)3)6] (Q = S (1) or Se (2)) were synthesised by the hydrothermal reaction between Na4[{Re6Se8}(OH)6] and P(C2H4COOH)3·HCl (130 °C, 48 h) and purified by the methods described before.14
Osmolality of complexes (1), (2) and (3)
The osmolalities of aqueous solutions (1)–(3) were measured with a freezing point depression osmometer (OCKP-1, KIVI Osmometry, Saint Petersburg, Russian Federation). Each point was measured three times and the average value was determined.
Cell culture
Mouse peritoneal macrophages (MPh) were obtained from Balb/C mice weighing 20–22 g. Animals were euthanized by cervical dislocation after a one week acclimation period (temperature 23 ± 2 °C and humidity 60%, free access to water and standard food). Mice were injected intraperitoneally with 3 mL of phosphate buffer saline (PBS), then the peritoneal lavage fluid was washed and the peritoneal macrophages were collected and seeded in appropriate plates ready for in vitro experiments.
Human larynx carcinoma cell line (Hep-2) and human epithelioid cervix carcinoma cell line (HeLa) were purchased from the State Research Center of Virology and Biotechnology VECTOR (Russian Federation).
All cells were cultured in Eagle's Minimum Essential Medium (MEM, pH = 7.4) supplemented with a 10% fetal bovine serum (FBS) under a humidified atmosphere (5% CO2 and 95% air) at 37 °C.
MTT-assay
MPh, Hep-2 and HeLa cells were seeded into 96-well plates at the concentration of 5–7 × 103 cells per well and incubated for 24 h under a 5% CO2 atmosphere at 37 °C. The cells were then treated with solutions of (1), (2) and (3) taken in a broad range of concentrations (from 0.0056 to 5.76 mM) and incubated for 72 h under the same conditions. MTT reagent was added to each well to achieve a final concentration of 250 μg mL−1, and the plates were incubated for 4 h. The formazan formed was then dissolved in DMSO (100 μL). The optical density was measured with a plate reader Multiskan FC (Thermo Scientific, USA) at a wavelength of 570 nm. The experiment was repeated three times on separate days.
Quantitative data obtained were analysed using Stastistica 10.0 (StatSoft). Analysis between groups for the MTT assay was assessed by the Mann–Whitney U test. The p values <0.05 were considered statistically significant.
Confocal fluorescence imaging
The cells were seeded on microscope slides at the concentration of 1 × 105 cells per slide and incubated at 37 °C under a 5% CO2 atmosphere for 24 h. The culture medium was then replaced for the culture medium containing 0.18 mmol of (1), (2) and (3) and the cells were incubated for 24 h. The cells were then washed with PBS and fixed in 4% paraformaldehyde. The cells were visualised using a Zeiss LSM 510 confocal microscope (Carl Zeiss Inc., Jena, Germany) equipped with a laser diode (405 nm) for fluorescence and with a 100× oil immersion objective.
Animals and housing conditions
All animal procedures were carried out in accordance with the protocols approved by the bio-ethics committee of Siberian Branch of the Russian Academy of Sciences, recommendations for proper use and care of laboratory animals (European Communities Council Directive 86/609/CEE) and principles of the Declaration of Helsinki. Mice were housed in stainless steel cages containing sterile paddy husk as bedding in ventilated animal rooms with free access to water and a commercial laboratory complete food. Animals were acclimatised for 1 week prior to the experimental use.
Median lethal dose assessment
Ninety 8-week-old Balb/C mice, each having a body weight of 20 ± 2 g, were randomised into 9 groups of 10 animals per group (5 males and 5 females in each group) and received intravenous injection in the tail vein of 0.1 mL of an aqueous solution of the cluster complex (1) or (2) at doses 50, 150, 250 or 350 mg (Re) per kg, or sham-injected with the same volume of physiological saline. The solutions for intravenous infusion were used at the physiological pH = 7.4 that was achieved by the addition of NaOH. The animals were regularly observed for clinical signs and mortality for two weeks. After this period the mice were sacrificed.
Conclusions
In conclusion, in vitro studies have shown that the cytotoxicity and cellular internalisation behavior of complexes (1) and (2) are comparable to those of the complex (3). However, due to the increased osmolality of the complexes (1) and (2), their intravenous acute toxicity in vivo is significantly higher. Thus our study demonstrates that even a minor chemical change of the ligand environment of the cluster that alters the overall charge of the cluster anion can have a drastic effect with regard to the toxicity of the compound. Our study has also demonstrated that in terms of toxicity and internalisation, there is no significant difference between {Re6S8}2+ and {Re6Se8}2+. Moving forward, one way to produce highly effective X-ray contrast rhenium octahedral species of similar chemical structures, but reduced acute toxicity would be the esterification of all or some of the carboxylate groups of the organic ligands to reduce the overall charge of the complexes.
Acknowledgements
This work was supported by the Russian Science Foundation under Grant 15-15-10006.
References
- K. A. Brylev, M. A. Shestopalov, O. P. Khripko, V. A. Trunova, V. V. Zvereva, C. C. Wang, Y. V. Mironov and V. E. Fedorov, Biodistribution of rhenium cluster complex K4[Re6S8(CN)6] in the body of laboratory rats, Bull. Exp. Biol. Med., 2013, 155, 741 CrossRef CAS PubMed
.
- S. Cordier, Y. Molard, K. A. Brylev, Y. V. Mironov, F. Grasset, B. Fabre and N. G. Naumov, Advances in the engineering of near infrared emitting liquid crystals and copolymers, extended porous frameworks, theranostic tools and molecular junctions using tailored Re6 cluster building blocks, J. Cluster Sci., 2015, 26, 53 CrossRef CAS
.
- J. G. Elistratova, A. R. Mustafina, K. A. Brylev, K. A. Petrov, M. A. Shestopalov, Y. V. Mironov, V. M. Babaev, I. K. Rizvanov, P. Masson and O. G. Sinyashin, Sensing activity of cholinesterases through a luminescence response of the hexarhenium cluster complex [{Re6S8}(OH)6]4−, Analyst, 2016, 141, 4204 RSC
.
- L. Gao, M. A. Peay and T. G. Gray, Encapsulation of phosphine-terminated rhenium(III) chalcogenide clusters in silica nanoparticles, Chem. Mater., 2010, 22, 6240 CrossRef CAS
.
- M. Kubeil, H. Stephan, H.-J. Pietzsch, G. Geipel, D. Appelhans, B. Voit, J. Hoffmann, B. Brutschy, Y. V. Mironov, K. A. Brylev and V. E. Fedorov, Sugar-decorated dendritic nanocarriers: encapsulation and release of the octahedral rhenium cluster complex [Re6S8(OH)6]4−, Chem. – Asian J., 2010, 5, 2507 CrossRef CAS PubMed
.
- S.-Y. Lee, A. Fiene, W. Li, T. Hanck, K. A. Brylev, V. E. Fedorov, J. Lecka, A. Haider, H.-J. Pietzsch, H. Zimmermann, J. Sévigny, U. Kortz, H. Stephan and C. E. Müller, Polyoxometalates—potent and selective ecto-nucleotidase inhibitors, Biochem. Pharmacol., 2015, 93, 171 CrossRef CAS PubMed
.
- M. A. Shestopalov, K. E. Zubareva, O. P. Khripko, Y. I. Khripko, A. O. Solovieva, N. V. Kuratieva, Y. V. Mironov, N. Kitamura, V. E. Fedorov and K. A. Brylev, The first water-soluble hexarhenium cluster complexes with a heterocyclic ligand environment: synthesis, luminescence, and biological properties, Inorg. Chem., 2014, 53, 9006 CrossRef CAS PubMed
.
- S.-J. Choi, K. A. Brylev, J.-Z. Xu, Y. V. Mironov, V. E. Fedorov, Y. S. Sohn, S.-J. Kim and J.-H. Choy, Cellular uptake and cytotoxicity of octahedral rhenium cluster complexes, J. Inorg. Biochem., 2008, 102, 1991 CrossRef CAS PubMed
.
- A. A. Krasilnikova, M. A. Shestopalov, K. A. Brylev, I. A. Kirilova, O. P. Khripko, K. E. Zubareva, Y. I. Khripko, V. T. Podorognaya, L. V. Shestopalova, V. E. Fedorov and Y. V. Mironov, Prospects of molybdenum and rhenium octahedral cluster complexes as X-ray contrast agents, J. Inorg. Biochem., 2015, 144, 13 CrossRef CAS PubMed
.
- A. A. Krasilnikova, A. O. Solovieva, A. A. Ivanov, K. E. Trifonova, T. N. Pozmogova, A. R. Tsygankova, A. I. Smolentsev, E. I. Kretov, D. S. Sergeevichev, M. A. Shestopalov, Y. V. Mironov, A. M. Shestopalov, A. F. Poveshchenko and L. V. Shestopalova, Comprehensive study of hexarhenium cluster complex Na4[{Re6Te8}(CN)6] – in terms of a new promising luminescent and X-ray contrast agent, Nanomedicine, 2017, 13, 755 CrossRef CAS PubMed
.
- A. A. Krasilnikova, A. O. Solovieva, K. E. Trifonova, K. A. Brylev, A. A. Ivanov, S.-J. Kim, M. A. Shestopalov, M. S. Fufaeva, A. M. Shestopalov, Y. V. Mironov, A. F. Poveshchenko and L. V. Shestopalova, Cellular internalization and morphological analysis after intravenous injection of a highly hydrophilic octahedral rhenium cluster complex – a new promising X-ray contrast agent, Contrast Media Mol. Imaging, 2016, 11, 459 CrossRef CAS PubMed
.
- J. G. Elistratova, K. A. Brylev, A. O. Solovieva, T. N. Pozmogova, A. R. Mustafina, L. V. Shestopalova, M. A. Shestopalov, V. V. Syakayev, A. A. Karasik and O. G. Sinyashin, Supporting effect of polyethylenimine on hexarhenium hydroxo cluster complex for cellular imaging applications, J. Photochem. Photobiol., A, 2017, 340, 46 CrossRef CAS
.
- T. N. Pozmogova, A. A. Krasil'nikova, A. A. Ivanov, M. A. Shestopalov, S. N. Gyrylova, L. V. Shestopalova, A. M. Shestopaloiv and V. A. Shkurupy, Studying the effect of a composition of the cluster core in high-radiopacity cluster complexes of rhenium on their acute toxicity in vivo, Bull. Exp. Biol. Med., 2016, 161, 64 CrossRef CAS PubMed
.
- E. V. Svezhentseva, A. A. Ivanov, Y. A. Vorotnikov, S. N. Gyrylova, O. G. Kurskaya, M. A. Gulyaeva, A. Y. Alekseev, Y. V. Mironov, M. A. Shestopalov and A. M. Shestopalov, Materials based on X-ray contrast octahedral metal cluster complexes and hydrophilic polymers, Mater. Today Proc., 2017 Search PubMed
, accepted manuscript.
- A. M. Cheplakova, A. O. Solovieva, T. N. Pozmogova, Y. A. Vorotnikov, K. A. Brylev, N. A. Vorotnikova, E. V. Vorontsova, Y. V. Mironov, A. F. Poveshchenko, K. A. Kovalenko and M. A. Shestopalov, Nanosized mesoporous metal–organic framework MIL-101 as a nanocarrier for photoactive hexamolybdenum cluster compounds, J. Inorg. Biochem., 2017, 166, 100 CrossRef CAS PubMed
.
- K. Kirakci, P. Kubát, M. Kučeráková, V. Šícha, H. Gbelcová, P. Lovecká, P. Grznárová, T. Ruml and K. Lang, Water-soluble octahedral molybdenum cluster compounds Na2[Mo6I8(N3)6] and Na2[Mo6I8(NCS)6]: Syntheses, luminescence, and in vitro studies, Inorg. Chim. Acta, 2016, 441, 42 CrossRef CAS
.
- A. O. Solovieva, Y. A. Vorotnikov, K. E. Trifonova, O. A. Efremova, A. A. Krasilnikova, K. A. Brylev, E. V. Vorontsova, P. A. Avrorov, L. V. Shestopalova, A. F. Poveshchenko, Y. V. Mironov and M. A. Shestopalov, Cellular internalisation, bioimaging and dark and photodynamic cytotoxicity of silica nanoparticles doped by {Mo6I8}4+ metal clusters, J. Mater. Chem. B, 2016, 4, 4839 RSC
.
- N. A. Vorotnikova, O. A. Efremova, A. R. Tsygankova, K. A. Brylev, M. V. Edeleva, O. G. Kurskaya, A. J. Sutherland, A. M. Shestopalov, Y. V. Mironov and M. A. Shestopalov, Characterization and cytotoxicity studies of thiol-modified polystyrene microbeads doped with [{Mo6X8}(NO3)6]2– (X = Cl, Br, I), Polym. Adv. Technol., 2016, 27, 922 CrossRef CAS
.
- R. van Furth, Z. A. Cohn, J. G. Hirsch, J. H. Humphrey, W. G. Spector and H. L. Langevoort, The mononuclear phagocyte system: a new classification of macrophages, monocytes, and their precursor cells, Bull. W. H. O., 1972, 46, 845 CAS
.
- M. Zhang, M. Yang, C. Bussy, S. Iijima, K. Kostarelos and M. Yudasaka, Biodegradation of carbon nanohorns in macrophage cells, Nanoscale, 2015, 7, 2834 RSC
.
- J. S. Yoo, R. K. Das, Z. Y. Jow and Y. T. Chang, In vivo detection of macrophage recruitment in hind-limb ischemia using a targeted near-infrared fluorophore, PLoS One, 2014, 9, e103721 Search PubMed
.
- S. B. Lee, H. W. Lee, T. D. Singh, Y. Li, S. K. Kim, S. J. Cho, S. W. Lee, S. Y. Jeong, B. C. Ahn, S. Choi, I. K. Lee, D. K. Lim, J. Lee and Y. H. Jeon, Visualization of macrophage recruitment to inflammation lesions using highly sensitive and stable radionuclide-embedded gold nanoparticles as a nuclear bio-imaging platform, Theranostics, 2017, 7, 926 CrossRef PubMed
.
- J.-H. Park and N. Oh, Endocytosis and exocytosis of nanoparticles in mammalian cells, Int. J. Nanomed., 2014, 9, 51 CrossRef PubMed
.
- K. Xiao, Y. Li, J. Luo, J. S. Lee, W. Xiao, A. M. Gonik, R. Agarwal and K. S. Lam, The effect of surface charge on in vivo biodistribution of PEG-oligocholic acid based micellar nanoparticles, Biomaterials, 2011, 32, 3435 CrossRef CAS PubMed
.
- M. Fillon, Details linking chronic inflammation and cancer continue to emerge, J. Natl. Cancer Inst., 2013, 105, 509 CrossRef PubMed
.
- C. Franceschi and J. Campisi, Chronic inflammation (inflammaging) and its potential contribution to age-associated diseases, J. Gerontol., Ser. A, 2014, 69, S4 CrossRef PubMed
.
- P. Hunter, The inflammation theory of disease, EMBO Rep., 2012, 13, 968 CrossRef CAS PubMed
.
- I. Manabe, Chronic inflammation links cardiovascular, metabolic and renal diseases, Circ. J., 2011, 75, 2739 CrossRef CAS PubMed
.
- J. Domey, U. Teichgräber and I. Hilger, Gold nanoparticles allow detection of early-stage edema in mice via computed tomography imaging, Int. J. Nanomed., 2015, 10, 3803 CrossRef CAS PubMed
.
- X.-W. Hua, T.-F. Lu, D.-W. Li, W.-G. Wang, J. Li, Z.-Z. Liu, W.-W. Lin, J.-J. Zhang and Q. Xia, Contrast-enhanced micro-computed tomography using ExiTron nano6000 for assessment of liver injury, World J. Gastroenterol., 2015, 21, 8043 Search PubMed
.
- M. Krenkel, A. Markus, M. Bartels, C. Dullin, F. Alves and T. Salditt, Phase-contrast zoom tomography reveals precise locations of macrophages in mouse lungs, Sci. Rep., 2015, 5, 9973 CrossRef CAS PubMed
.
- M. Nahrendorf, E. Keliher, B. Marinelli, F. Leuschner, C. S. Robbins, R. E. Gerszten, M. J. Pittet, F. K. Swirski and R. Weissleder, Detection of macrophages in aortic aneurysms by nanoparticle positron emission tomography–computed tomography, Arterioscler., Thromb., Vasc. Biol., 2011, 31, 750 CrossRef CAS PubMed
.
- W. Wang, J. Li, R. Liu, A. Zhang and Z. Yuan, Size effect of Au/PAMAM contrast agent on CT imaging of reticuloendothelial system and tumor tissue, Nanoscale Res. Lett., 2016, 11, 429 CrossRef PubMed
.
- J.-J. Lahet, F. Lenfant, J. Lecordier, A. Bureau, L. Duvillard, B. Chaillot and M. Freysz, Effects of various osmolarity on human red blood cells in terms of potassium efflux and hemolysis induced by free radicals, Biomed. Pharmacother., 2008, 62, 697 CrossRef CAS PubMed
.
- K. S. Lang, P. A. Lang, C. Bauer, C. Duranton, T. Wieder, S. M. Huber and F. Lang, Mechanisms of suicidal erythrocyte death, Cell. Physiol. Biochem., 2005, 15, 195 CrossRef CAS PubMed
.
- N. Dennhardt, S. Schoof, W. A. Osthaus, L. Witt, H. Bertram and R. Sümpelmann, Alterations of acid–base balance, electrolyte concentrations, and osmolality caused by nonionic hyperosmolar contrast medium during pediatric cardiac catheterization, Pediatr. Anesth., 2011, 21, 1119 CrossRef PubMed
.
- K. A. Brylev, Y. V. Mironov, S. S. Yarovoi, N. G. Naumov, V. E. Fedorov, S.-J. Kim, N. Kitamura, Y. Kuwahara, K. Yamada, S. Ishizaka and Y. Sasaki, A family of octahedral rhenium cluster complexes [Re6Q8(H2O)n(OH)6-n]n–4 (Q = S, Se; n = 0-6): structural and pH-dependent spectroscopic studies, Inorg. Chem., 2007, 46, 7414 CrossRef CAS PubMed
.
|
This journal is © The Royal Society of Chemistry 2017 |