Effects of sorafenib and cisplatin on preneoplastic foci of altered hepatocytes in fetal turkey liver†
Received
22nd August 2016
, Accepted 28th October 2016
First published on 2nd November 2016
Abstract
Foci of altered hepatocytes (FAH) were induced in fetal turkey liver (FTL) by diethyl nitrosamine. FAH in FTL were resistant to iron overload similar to FAH in humans and rodents. The mitotic index was significantly higher in FAH (6.2 mitosis in 1000 hepatocytes) than in extrafocal liver tissue (1.8 mitosis in 1000 hepatocytes). The calculation of the net growth rate based on both cell proliferation (mitosis) and cell death (TUNEL positive) revealed a threefold growth advantage of the FAH over the surrounding liver. Two well established anti-tumor substances from different chemical classes, different modes of action and with different clinical use in the treatment of hepatocellular carcinoma (HCC) were used to study their effect on FAH. Sorafenib is the only approved drug for systemic pharmacological treatment of HCC; cisplatin has been used for many years in hepatic arterial infusion. Cisplatin had no clear effect on number of size of FAH, cell proliferation (mitosis) or cell loss (TUNEL positive). Sorafenib enhanced the development of FAH. Morphometric quantification revealed a sorafenib-induced 2–3-fold increase in number (FAH per cm2 and FAH per cm3), size and volume fraction of FAH. This unexpected finding was confirmed in two experiments. The effect was driven by an increased cell proliferation in the FAH, resulting in an increased, 5.4-fold growth advantage of FAH versus the surrounding liver in sorafenib-treated FTL. In this model, sorafenib has a promoting effect on preneoplastic FAH. This might be of relevance for the treatment of patients with long term survival perspective, e.g. in an adjuvant setting.
Introduction
Sasaki and Yoshida were the first to notice that characteristic hepatocellular changes, namely early emerging “big light” and later appearing “smaller dark” cells, precede the occurrence of chemically induced liver cell tumors.1 Later on, different types of foci of altered hepatocytes (FAH) were discovered by cytomorphological and cytochemical approaches, progressing through an ordered sequence of distinct focal lesions to benign and malignant liver cell tumors.2 FAH have been known as pre-stages of hepatocellular carcinomas (HCC) in several species including humans.3 The chemical induction of FAH in rat liver has been repeatedly proposed as possible endpoint in carcinogenicity testing.4
FAH have also been used to study mechanistic aspects of carcinogenesis5,6 including initiation-promotion models. In the initiation arms of these studies investigators look for the de novo induction of FAH. Promotion may be observed as the effects of a test chemical or medicine on the development of preexisting, either spontaneously occurring or previously induced FAH. A promoting effect defined as a treatment-induced growth advantage of the hepatocytes in the FAH will result in an increase in number or size of FAH. An inhibition or delay of the development of FAH is regarded as indicative for an anticarcinogenic or anti-promoting effect. Based on their effects on FAH in rodent liver food constituents have been proposed as potential candidates for a pharmacological cancer prevention.7–9
As alternative to experiments in mammals, FAH can be induced in fetal turkey liver (FTL) by nitrosamines, and histologically defined FAH are easily perceivable in H&E stained sections. An interlaboratory study demonstrated that a single dose of diethylnitrosamine (DEN) will reliably result in FAH regardless of the turkey strain used and of minor modifications in the incubation conditions of the eggs.10
To test the hypothesis, that the development of FAH in FTL can be inhibited or delayed by pharmaceuticals, we administered antineoplastic substances subsequent to the induction of FAH in FTL by DEN. Two well established anti-tumor substances from different chemical classes, different mode of action and with different clinical use in the treatment of hepatocellular carcinoma were used. Sorafenib is a multi-protein kinase inhibitor with effects both on tumor cells and the vascularization of tumors, and until now the only systemic pharmacological treatment for HCC that has been approved by the US Food and drug administration11 and the European Medicines Agency.12 Cisplatin is a widely used cytotoxic anti-tumor drug. Cisplatin has been successfully used for the treatment of various tumors and may even be the backbone of the systemic therapy of several solid tumors. Whereas cisplatin has not been established for systemic therapy of hepatocellular cancer due to response rates of <10%,13 cisplatin has been used for many years in hepatic arterial infusion.14 The effect of both anti-tumor drugs on the number and size of and cell proliferation and cell death in FAH was quantified.
Materials and methods
Hatching eggs
Fertilized turkey eggs (FTE) were purchased from Thole Geflügelzüchterei GmbH in Bosel, Germany. Damaged eggs and eggs with less than 90% or more than 110% of the average weight were discarded. Eggs were incubated at 37.5 °C, 65% relative humidity and turned four times a day unless the egg shell had been opened and the CAM exposed.
Chemicals
Sorafenib (p-toluensulfonat) was from LC Laboratories, Woburn, MA, USA, Cisplatin “Cis-GRYR” (1 mg mL−1) was from TEVA GmbH, Ulm, Germany, diethylnitrosamine was from Sigma-Aldrich GmbH, Steinheim, Germany, iron(III)-hydroxide sucrose complex (IS) as Venofer®, containing 100 mg Fe3+ in a 5 ml ampoule was from Vifor France S.A., Neuilly-Sur-Seine, France. Dead cells in liver section were stained with the In situ cell death detection kit, POD from Roche Diagnostics Deutschland GmbH, Mannheim, Germany. Proteinase K (min. 3000 U mg−1) was from Qiagen GmbH, Hilden, Germany.
Administration procedures
DEN (2 mg at day 0, in water) and sorafenib (0.5 mg or 1.0 mg at day 8, in DMSO) were injected into the albumen. The eggs were positioned with the large end pointing upwards. After swabbing the injection site with 70% ethanol, the shell was pierced approximately 2 cm from the pointed end. The test substance was injected into the white of each egg using a 0.4 mm hypodermic needle, and the injection site was sealed with adhesive tape.
Cisplatin (0.25 mg or 0.5 mg or 1.0 mg at day 20, in 0.9% saline) and iron sucrose (400 μg Fe3+ at day 20, as Venofer®) were administered to the CAM: on the 7th day of incubation the apical pole of the egg was swabbed with alcohol. A small hole of less than a millimeter in diameter was drilled in the eggshell at the apical pole of the egg. The hole was sealed with adhesive tape (Leukosilk®). Eggs were returned to the incubator and incubation continued with the eggs in upright position (apical pole up) without rotation. On the 14th day of incubation, the hole was extended to approximately 2.5 centimeters in diameter. The hole in the shell was covered with a watch glass fixed with adhesive tape and incubation was continued with the eggs in upright position (apical pole up) without rotation. Cisplatin was applied on the CAM and iron sucrose was injected into a CAM blood vessel.
Tissue sampling
At day 24 the fetuses were removed and decapitated. The abdominal cavity was opened, the liver was removed, weighed and fixed in 10% phosphate buffered formalin (containing 4% formaldehyde) for histology. All experiments were performed in full compliance with national laws, following institutional rules and guidelines at the Federal Institute for Drugs and Medical Devices (Bundesinstitut für Arzneimittel und Medizinprodukte). The project was approved by the Research Council and authorized by the President of the Federal Institute for Drugs and Medical Devices.
Histochemical reactions
Tissue samples were embedded in paraffin. For each liver, slides were made for several (usually 5) section planes with the distance between different sections planes 0.5 mm, mounted and stained. Mitotic figures were quantified in haematoxylin and eosin stains (H&E). Cell death was demonstrated with terminal-deoxy-nucleotidyl-transferase mediated d-UTP nick-end-labeling (In situ cell death detection kit TUNEL) from Roche. The deparaffinized slides were pretreated with Proteinase K (20 μg mL−1) at 30 °C for 15 min, incubation with reaction mix was at 37 °C for 60 min.
For the histochemical demonstration of iron with the Perl's Prussian blue reaction according to ref. 15 paraffin sections were stained with potassium hexacyanoferrat(II) and a nuclear fast red counterstain.
Morphometry of FAH
H&E stained paraffin sections were used for the identification and quantification of FAH with increased cytoplasmic basophilia or eosinophilia. The areas of FAH and of the liver sections were measured in digital images taken with a 6.3× objective lens magnification for the area of the liver section and with a 40× objective lens magnification for FAH. For few unusually large FAH a 25× objective lens magnification was used. Surface fraction (resp. volume fraction) of FAH was calculated dividing the sum of all FAH areas in a liver section by the evaluated area of the section.
The numerical density of FAH (number of FAH per volume of liver tissue) was calculated from the morphometric data by three different methods: without lower limit of detection (method 1) as originally suggested by Fullman and later improved by Pugh and colleagues16 introducing a detection threshold (method 2), and with a size classes – based approach (method 3) specifically designed for liver foci.17 For the calculation of FAH per liver an estimation of the liver volume based on the liver weight assuming a specific mass density of 1 g cm−3 for FTL was used.
Quantification of cell proliferation
Mitotic figures (prometa-, meta- and anaphase) in hepatocytes were counted in high magnification (400-fold) photographs. For FAH photographs were taken of the whole area and mitotic figures were counted in all photographs (number of photographs depending on size of FAH). For the extrafocal tissue 40 photographs per liver were evaluated. Hepatocyte nuclei were counted in 5 photographs for each FAH (less for very small FAH) and for each extrafocal liver tissue sample. The total number of hepatocytes per area was calculated as the sum of mitotic figures and nuclei. The number of mitotic hepatocytes per 1000 hepatocytes was used as parameter for cell proliferation.
Quantification of cell loss
All TUNEL-positive hepatocytes in the FAH were counted, for the extrafocal tissue 10 views per section were evaluated, and the number of TUNEL-positive hepatocytes per section area was calculated. The total number of hepatocytes per area was quantified as described above. The number of TUNEL-positive hepatocytes per 1000 hepatocytes was used as parameter for cell loss.
Calculation of the net growth rate (NGR) of hepatocytes
The net growth rate was defined as the difference in the number of cells proliferating and the number of cells lost within 24 hours. The numbers for mitotic figures or TUNEL-positive cells per 1000 cells observed in histological sections were corrected for the vastly different time span a mitotic or apoptotic cell is histologically perceivable. The number of cells in mitosis or dying within 24 hours was calculated based on histological detection within a time window of 30 minutes for mitotic cells in prometaphase, metaphase or anaphase and of a time window of 3 hours for TUNEL-positive nuclei.
Statistical analysis
For statistical analysis the program SPSS (version 10.0.7) was used. Statistical significance of group differences was tested by Student's t-test or the Mann–Whitney-U-test, depending on the results of the Kolmogorov–Smirnov-test for normal distribution.
Results
Induction of FAH
For the initiation of hepatocarcinogenesis FTE were injected with 2 mg DEN prior to incubation. This dose was sufficiently high to reliably produce FAH without severe changes of the surrounding extrafocal liver tissue. Higher doses resulted in more FAH but excessively damaged the extrafocal liver tissue by the induction of necrosis and abundant karyomegaly and resulted in poor survival rates particularly after additional treatments with sorafenib or CP.
Most the FAH induced by 2 mg DEN were characterized by a strongly basophilic cytoplasm and frequently showed pseudoglandular structures. Few FAH were composed of hepatocytes with a more eosinophilic cytoplasm, only very rarely small clear cell foci were observed. The size of the FAH was variable from a few cells to macroscopically detectable lesions of more than one mm in diameter. The DEN-induced FAH were resistant to iron overload. The injection of 400 μg iron as iron sucrose into blood vessels of the CAM induced an excessive hepatic iron storage in extrafocal hepatic tissue but not or to a much lesser extent in basophilic FAH (Fig. 1).
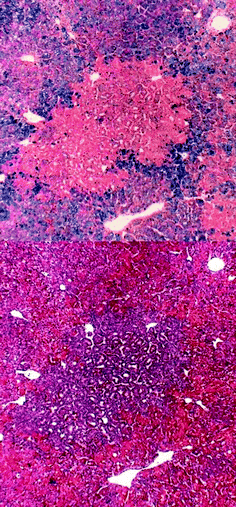 |
| Fig. 1 Top: DEN-induced iron resistant FAH in FTL. Bottom: Basophilic FAH with pseudoglandular structures; serial section to iron resistant FAH at top. | |
Cell growth in FAH and extrafocal liver
The FTL is a rapidly growing tissue with a high number of proliferating cells. Mitotic figures in H&E stained sections were used for the quantification of cell proliferation and the TUNEL assay for the quantification of cell loss. Mitotic figures and TUNEL-positive nuclei were several fold more frequent in FAH than in the surrounding extrafocal liver tissue (p < 0.001, Table 1). The similar numbers for mitotic figures and TUNEL-positive cells perceivable in the histological sections do not mean that cell proliferation and cell loss are balanced in FTL. The actual growth or net growth rate (NGR) of a cell population will depend on the difference between cell proliferation and cell loss, e.g. the difference between the number of cells entering mitosis and the number of cells entering apoptosis within 24 hours. The number of cells entering mitosis or apoptosis within a given time (24 hours) was calculated from the number of cells with mitotic figures or TUNEL-positive nuclei and the time span for which either the mitotic figures or the TUNEL-positive nuclei are detectable in histological sections (for details see material and methods section). After correction for the different time windows for the detection of cell proliferation by mitotic figures and of cell loss by TUNEL-positive hepatocytes the net growth rate of hepatocytes in FTL was 46.5 cells per 1000 hepatocytes in 24 h, which is a plausible value for this rapidly growing tissue.
Table 1 Mitotic figures and TUNEL-positive nuclei per 1000 hepatocytes in extrafocal liver and in FAH. AM = arithmetic mean, SD = standard deviation, Cl = 95% confidence interval
|
Mitotic figures |
TUNEL-positive nuclei |
|
Extrafocal |
FAH |
Extrafocal |
FAH |
AM
|
1.80
|
6.15
|
1.77
|
7.95
|
SD |
0.84 |
3.55 |
0.51 |
4.16 |
CI |
1.34–2.27 |
4.80–7.51 |
1.49–2.06 |
6.30–9.39 |
n
|
15 |
29 |
15 |
27 |
Whereas the increase in TUNEL-positive hepatocytes in FAH does indicate an increased cell turn over in FAH, the increase in cell proliferation clearly outweighs the increased cell loss, translating into a growth advantage of the FAH. The net growth rate in the FAH was 138.3 per 1000 hepatocytes in 24 hours, i.e. threefold higher than the growth rate in the surrounding extrafocal tissue.
Effect of sorafenib on survival and liver weight
The dose effect curve was rather steep for sorafenib in FTE. In untreated FTE, any dose of more than 2 mg sorafenib was lethal to all or almost all FTE with a steep increase in mortality from 1.1 to 1.8 mg. Lethality was not perceivably increased at 1.1 mg Sorafenib, slightly increased at 1.4 mg and doubled at 1.8 mg. The pretreatment of FTE with DEN for the induction of FAH increased their sensitivity to the toxic effect of sorafenib. After induction of FAH in FTL with DEN, treatment with 1 mg sorafenib at day 8 resulted in a decreased survival rate compared to control, a minor decrease in embryo weight and no effects on absolute liver weight (Table 2).
Table 2 Effect of sorafenib on survival, embryo and liver weight, FTE previously injected with 2 mg DEN
|
DEN |
Sorafenib [mg] |
Survival rate [%] |
Embryo weight [g] |
Abs. liver weight [g] |
Rel. liver weight [%] |
AM |
SD |
AM |
SD |
AM |
SD |
Exp. 1 |
2 mg |
— |
65 |
45.3 |
9.6 |
0.82 |
0.11 |
1.88 |
0.45 |
|
2 mg |
1 |
40 |
38.0 |
5.0 |
0.79 |
0.33 |
2.08 |
0.26 |
|
Exp. 2 |
2 mg |
— |
88 |
43.5 |
4.6 |
0.74 |
0.13 |
1.70 |
0.22 |
|
2 mg |
0.5 |
89 |
41.4 |
3.9 |
0.75 |
0.08 |
1.81 |
0.18 |
|
2 mg |
1 |
50 |
41.0 |
4.5 |
0.78 |
0.11 |
1.92 |
0.29 |
Effect of sorafenib on development of FAH
The development of FAH in FTL was enhanced by sorafenib: the original hypothesis was that sorafenib would have an inhibitory effect on the development of FAH in FTL. At variance with this we observed an about twofold (2.48 fold, not statistically significant) increase in the number of FAH per cm2 at a dose of 1 mg sorafenib per FTE. In a second experiment with more samples the dose of 1 mg sorafenib was repeated and a lower dose of 0.5 mg was added (Table 3). The second experiment confirmed the promoting effect of 1 mg sorafenib and the results achieved statistical significance. The size of the promoting effect of sorafenib was very similar although overall more FAH were induced in experiment 2 than in experiment 1. In the first experiment, the number of FAH per cm2 was increased 2.48-fold and in the second experiment, the number of FAH per cm2 was increased 2.31-fold. The effect was even clearer for the volume fraction of FAH (percentage of liver occupied by FAH) and the size of FAH (Fig. 2). The dose of 0.5 mg sorafenib included in the second experiment had no effect. Stereological calculations of the numerical density of FAH (number of FAH per cm3 or per liver) showed an about twofold increase regardless of the calculation method used (Table 4).
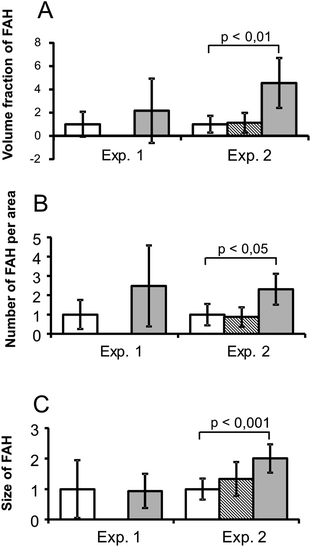 |
| Fig. 2 Morphometric measurements of the effect of sorafenib on FAH in FTL. A: volume fraction of FAH (percentage of liver volume); B: number of FAH per area (foci cm−2); C: size (area) of FAH. White columns: reference group, 2 mg DEN at day 0; hatched columns: sorafenib low dose: 2 mg DEN at day 0, 0.5 mg DEN sorafenib at day 8; grey columns: sorafenib high dose: 2 mg DEN at day 0, 1.0 mg sorafenib at day 8. Values shown as n-fold of the arithmetic mean of the reference group (DEN only). Arithmetic means with 95% confidence interval, Wilcoxon–Mann–Whitney test. | |
Table 3 Effect of Sorafenib on FAH cm−2 in FTL. SF = sorafenib, DMSO = dimethyl sulfoxide (vehicle), AM = arithmetic mean, SD = standard deviation, Cl = 95%-confidence interval, nf = number of fetuses, nS = number of histological sections
|
Experiment 1 |
Experiment 2 |
DEN DMSO |
DEN 1 mg SF |
DEN DMSO |
DEN 0.5 mg SF |
DEN 1 mg SF |
AM
|
0.27 |
0.67 |
1.26 |
1.10 |
2.92 |
SD |
0.37 |
0.79 |
1.64 |
1.54 |
2.23 |
CI |
0.07–0.47 |
0.11–1.24 |
0.56–1.97 |
0.45–1.75 |
1.90–3.93 |
n
f (nS) |
15 (74) |
10 (49) |
23 (107) |
24 (108) |
21 (98) |
Table 4 Numerical density of FAH calculated with different stereological methods (see Materials and methods). SF = sorafenib, DMSO = dimethyl sulfoxide (vehicle), consistent effect of 1 mg sorafenib regardless of calculation method
|
Number of FAH per cm3 |
Number of FAH or per liver |
DEN DMSO |
DEN 0.5 mg SF |
DEN 1 mg SF |
DEN DMSO |
DEN 0.5 mg SF |
DEN 1 mg SF |
Method 1 |
41.6 |
38.3 |
79.1 |
30.1 |
28.7 |
61.7 |
Method 2 |
56.3 |
43.3 |
86.3 |
41.7 |
32.5 |
67.3 |
Method 3 |
57.6 |
50.5 |
111.9 |
42.6 |
37.9 |
87.3 |
To understand what drives the sorafenib-induced increase in FAH, the cell growth in FAH was quantified and compared to the cell growth in the surrounding extrafocal liver tissue.
Effect of sorafenib on cell growth
Sorafenib did not alter the frequency of mitotic figures in the extrafocal liver tissue. The number of TUNEL-positive cells increased possibly indicating a toxic effect of sorafenib on FTL. This effect on the incidence of TUNEL-positive hepatocytes was not perceivable in FAH. In contrast, treatment with sorafenib increased the mitotic activity in FAH (Fig. 3).
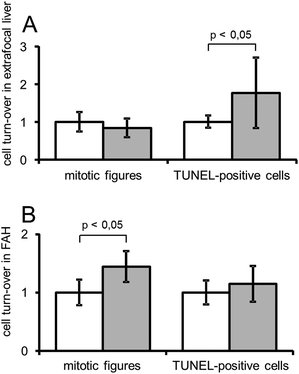 |
| Fig. 3 Effect of sorafenib on cell proliferation and cell death. A: Extrafocal hepatocytes B: hepatocytes in FAH. White columns: reference group, 2 mg DEN at day 0; grey columns: 2 mg DEN at day 0, 1.0 mg sorafenib at day 8. Values shown as n-fold of the arithmetic mean of the reference group (DEN only). Arithmetic means with 95% confidence interval, Wilcoxon–Mann–Whitney test. | |
The calculation of the NGR showed a sorafenib-induced decrease from 46/1000 to 37/1000 cells for extrafocal liver and an increase from 138/1000 to 199/1000 for FAH. Both effects combined result in a clearly increased growth advantage of FAH in sorafenib-treated FTL. In the control group (DEN only) the NGR of FAH was almost threefold higher (2.975) than the NGR of the surrounding extrafocal liver. In the sorafenib group (DEN + sorafenib) the NGR of FAH was more than five-fold higher (5.382) than the NGR of the surrounding extrafocal liver.
Effect of cisplatin
Cisplatin decreased the survival rate of untreated eggs at doses as low as 0.33 mg per egg with a clear increase in mortality at doses exceeding 1 mg (data not shown). In eggs pretreated with DEN, the dose of 1 mg cisplatin resulted in a 50% decrease of survival rates compared to vehicle injection after DEN treatment. The lower doses of 0.5 mg and 0.25 mg cisplatin had marginal (if any) effects on survival rates. Embryo weight was decreased at both doses of 1.0 and 0.5 mg cisplatin but not at the dose of 0.25 mg cisplatin (Table 5).
Table 5 Effect of cisplatin on survival, embryo and liver weight, FTE previously injected with 2 mg DEN
|
DEN |
Cisplatin [mg] |
Survival rate [%] |
Embryo weight [g] |
Abs. liver weight [g] |
Rel. liver weight [%] |
AM |
SD |
AM |
SD |
AM |
SD |
Exp. 1 |
2 mg |
— |
74 |
40.8 |
5.2 |
0.63 |
0.11 |
1.55 |
0.19 |
|
2 mg |
0.25 |
73 |
40.3 |
3.8 |
0.61 |
0.12 |
1.51 |
0.27 |
|
2 mg |
0.5 |
72 |
38.5 |
4.6 |
0.60 |
0.11 |
1.55 |
0.23 |
|
2 mg |
1 |
36 |
37.3 |
4.9 |
0.57 |
0.12 |
1.55 |
0.30 |
|
Exp. 2 |
2 mg |
— |
97 |
41.6 |
4.7 |
0.64 |
0.11 |
1.54 |
0.26 |
|
2 mg |
0.25 |
94 |
41.8 |
4.5 |
0.62 |
0.11 |
1.47 |
0.23 |
|
2 mg |
0.5 |
81 |
38.6 |
5.6 |
0.60 |
0.16 |
1.55 |
0.30 |
|
2 mg |
1 |
50 |
38.4 |
3.9 |
0.63 |
0.11 |
1.65 |
0.23 |
There was no clear effect of cisplatin on the development of FAH in FTL. The first experiment seemed to show a decrease in number and size of FAH. There was, however, no difference in the apparent effect of 0.25 mg, 0.5 mg and 1.0 mg cisplatin, i.e. no clear dose dependence. In a second experiment using the same doses no significant effects were observed. There was a numerical decrease in FAH at the higher doses of 1.0 mg and 0.5 mg cisplatin but no effect on the volume fraction or the size of the FAH (Fig. 4).
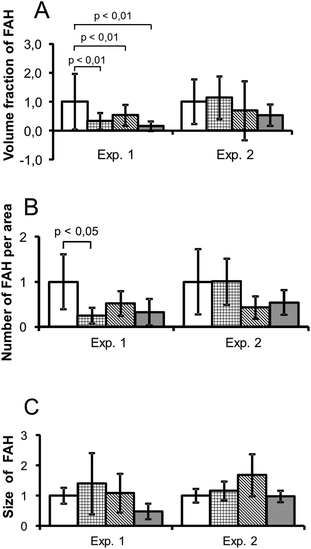 |
| Fig. 4 Morphometric measurements of the effect of cisplatin on FAH in FTL. A: Volume fraction of FAH (percentage of liver volume); B: number of FAH per area (foci cm−2); C: size (area) of FAH. White columns: reference group, 2 mg DEN at day 0; squared columns: cisplatin low dose: 2 mg DEN at day 0, 0.25 mg cisplatin at day 20; hatched columns: cisplatin mid dose: 2 mg DEN at day 0, 0.5 mg cisplatin at day 20; grey columns: cisplatin high dose: 2 mg DEN at day 0, 1.0 mg cisplatin at day 20. | |
In good agreement with morphometric data on numbers and size of FAH the quantification of cell proliferation and cell loss did not show a clear effect of cisplatin. In FAH but not in extrafocal tissue there may have been a tendency to a dose dependently increased cell turn over (Fig. 5).
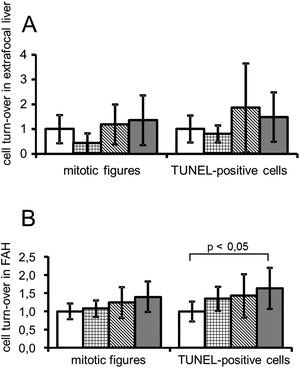 |
| Fig. 5 Effect of cisplatin on cell proliferation and cell death. A: Extrafocal hepatocytes B: hepatocytes in FAH. White columns: reference group, 2 mg DEN at day 0; squared columns: cisplatin low dose: 2 mg DEN at day 0, 0.25 mg cisplatin at day 20; hatched columns: cisplatin mid dose: 2 mg DEN at day 0, 0.5 mg cisplatin at day 20; grey columns: cisplatin high dose: 2 mg DEN at day 0, 1.0 mg cisplatin at day 20. Values shown as n-fold of the arithmetic mean of the reference group (DEN only). Arithmetic means with 95% confidence interval, Wilcoxon–Mann–Whitney test. | |
Discussion
In the experimental model of FTL, FAH can be reliably induced by DEN using turkey hatching eggs from different commercially available strains.10 Characteristic features of FAH in FTL are increased cytoplasmic basophilia, and they often develop pseudoglandular structures. Typical enzyme histochemical changes have been described in FAH in experimental animals and in humans many years ago18,19 and were later observed in FAH in FTL as well, e.g. decreased activities of glucose-6-phosphatase, adenosinetriphosphatase and glycogen phosphorylase,20 indicating the close similarity of FAH in FTL with preneoplastic FAH in mammals. Another landmark of hepatic preneoplasia, the resistance to iron accumulation was discovered in rat liver long ago,21,22 later observed in humans23,24 and has now been demonstrated in FAH in FTL for the first time. This finding further substantiates the likeness of FAH in mammals and FAH in FTL and the preneoplastic nature of FAH in this experimental model. Of note, excessive iron storage is per se carcinogenic. Hemochromatosis patients have a considerably increased risk for HCC and experimentally induced excessive iron storage in rats was associated with the occurrence of preneoplastic foci and nodules25 which might complicate the use of iron exclusion as marker reaction in studies on carcinogenesis or cancer prevention.
The quantification of cell proliferation and cell loss in FAH and in the extrafocal liver tissue showed that FAH in FTL have a significant growth advantage over the surrounding normal tissue. Very similar to findings in rodents26 this growth advantage is driven by a several fold increase in mitotic activity. An increased number of TUNEL-positive cells in the FAH is indicative of an enhanced cell-turn over in these lesions but the increase in cell proliferation clearly outweighs the increase in cell loss.
FAH in FTL are very similar to FAH in mammals with regard to their histomorphology, enzyme histochemical changes, increased cell proliferation and resistance to iron accumulation. Therefore, FAH in FTL can be considered as preneoplastic lesions and used to study the development of hepatic preneoplasia in situ in a non-mammalian system. The administration of test substances after previous induction of FAH by DEN provides an opportunity to study modulating effects on the preneoplastic lesions. Our original hypothesis that sorafenib or cisplatin may inhibit the development of these lesions was not confirmed; rather sorafenib treatment resulted in an increased number of FAH. This surprising finding of a twofold increase in FAH was reproducible and was consistently found with different morphometric parameters for the dose of 1 mg sorafenib but not for the lower dose of 0.5 mg sorafenib. This effect was driven by a sorafenib-induced increase in hepatocyte proliferation in the FAH. Proliferation of extrafocal hepatocytes was not changed by the treatment with sorafenib. A sorafenib-induced increase in the incidence of TUNEL-positive nuclei in the extrafocal liver tissue may be indicative of a moderately hepatotoxic effect of sorafenib on the fetal liver. Of note, this increase in TUNEL-positive nuclei was not observed in the FAH and this may be a sign that FAH are more resistant to the cytotoxicity of sorafenib than the surrounding parenchyma. Overall under sorafenib treatment the net growth rate of hepatocytes in FAH was 5.3 fold higher than the net growth rate of hepatocytes in the surrounding liver tissue. In comparison, without sorafenib treatment the net growth rate of hepatocytes in FAH was only 2.9 fold higher than in extrafocal liver tissue. Cisplatin had no effect on the FAH but with increasing doses a slight increase in cell proliferation and cell loss was observed in the extrafocal liver tissue. For Cisplatin these results seem to indicate a dose-dependent toxic effect resulting in an increased cell turn over with no perceivable effect on FAH. At variance sorafenib is mitogenic for hepatocytes in FAH and increases the number of preneoplastic FAH in FTL suggesting an enhancing or promoting effect on hepatocarcinogenesis in this experimental model.
In FTL sorafenib has a clear albeit moderate promoting effect on FAH. FAH have been identified as precursors of HCC in humans, and patients with HCC have an increased number of preneoplastic FAH.3 If sorafenib had a promoting effect on hepatocarcinogenesis in humans, this would explain why sorafenib was not effective in an adjuvant setting.27,28 Any effect on remaining hepatic micrometastases may have been offset by an unfavorable effect on the de novo development of HCC from preneoplastic focal lesions. The currently approved indications of sorafenib are advanced hepatocellular carcinoma and advanced renal cell carcinoma. In these patients a possible promoting effect of sorafenib on FAH is not regarded as relevant risk since the life expectancy of patients with advanced hepatocellular carcinoma or advanced renal cell carcinoma will depend on their primary cancer. This could be different in other indications, e.g. when sorafenib is developed for the use in adjuvant therapies27,29 with potentially curative effect in patients with longer life expectancy or even for the treatment of non-malignant disease such a liver fibrosis.30 In that case a more thorough assessment of any carcinogenic or promoting effect of sorafenib may be prudent. If the effect of sorafenib on FAH in FTL was directly extrapolated to mammals (rodents or humans), the twofold increase in FAH in FTL would translate into a twofold increase in FAH and later in tumors in mammals. This twofold increase in FAH would be considerably lower than the effect of phenobarbital which has often been used as a standard promoting agent in rat liver. Rather, the twofold increase in FAH in FTL seems similar in size to the mild effect of sucrose31 or fructose32,33 on hepatocarcinogenesis in rats. As the sensitivity of the FTL compared to e.g. rat liver for promoting effects is unknown it cannot be excluded that the promoting effect of sorafenib may be much stronger in rats (or other mammals) than in the FTL. It also might be that a twofold increase in FAH by 14 days exposure to sorafenib might result in a several fold increase in FAH after a several fold longer exposure.
It remains at present unclear why sorafenib has opposite effects on advanced hepatocellular carcinoma in humans and on FAH in FTL. This may be due to species differences or may indicate different effects of sorafenib on preneoplastic hepatocytes and fully transformed cancer cells in advanced tumors, possibly related to basically different metabolic states in its target cells in FAH and hepatocellular neoplasms. There is compelling evidence that hepatocarcinogenesis is characterized by a fundamental metabolic shift from gluconeogenesis and glycogenosis in early preneoplastic lesions towards the pentose-phosphate pathway and glycolysis in fully transformed malignant tumors.34 As sorafenib enhances glycolysis in liver cells35 it is conceivable that sorafenib may accelerate this shift. In that case the increased cell proliferation in FAH would reflect rather than drive the promoting effect of sorafenib. Studies on neoplasia and preneoplasia occurring simultaneously in the same experimental model or on FAH in humans might help to better understand the different effects of sorafenib.
Conclusions
Sorafenib has a promoting effect on hepatocarcinogenesis in FTL, driven by an increased proliferation of preneoplastic hepatocytes in FAH. It seems prudent to assess carcinogenicity of sorafenib prior to granting any indications in patients with considerably longer life expectancy particularly if there is reason to assume that these patients may have an increased number of FAH, i.e. patients at an increased risk for hepatocellular carcinoma.
Conflict of interest
There are no conflicts of interest to declare.
Acknowledgements
The authors greatly appreciate the assistance and contributions of Ms. Irene Bachmann.
References
- T. Sasaki and T. Yoshida, Experimentelle Erzeugung des Lebercarcinoms durch Fütterung mit o-Amidoazotoluol, Virchows Arch. Pathol. Anat. Physiol., 1935, 295(2), 175–200 CrossRef CAS.
- P. Bannasch, H. Enzmann, F. Klimek, E. Weber and H. Zerban, Significance of sequential cellular changes inside and outside foci of altered hepatocytes during hepatocarcinogenesis, Toxicol. Pathol., 1989, 17(4 Pt 1), 617–628 CAS ; discussion 629.
- Q. Su and P. Bannasch, Relevance of hepatic preneoplasia for human hepatocarcinogenesis, Toxicol. Pathol., 2003, 31(1), 126–133 CrossRef PubMed.
- H. Tsuda, M. Futakuchi, K. Fukamachi, T. Shirai, K. Imaida and S. Fukushima,
et al., A medium-term, rapid rat bioassay model for the detection of carcinogenic potential of chemicals, Toxicol. Pathol., 2010, 38(1), 182–187 CrossRef CAS PubMed.
- J. Groos, P. Bannasch, M. Schwarz and A. Kopp-Schneider, Comparison of mode of action of four hepatocarcinogens: A model-based approach, Toxicol. Sci., 2007, 99(2), 446–454 CrossRef CAS PubMed.
- H. C. Pitot, Adventures in hepatocarcinogenesis, Annu. Rev. Pathol.: Mech. Dis., 2007, 2, 1–29 CrossRef CAS PubMed.
- K. Nakagawa, K. Hosoe, T. Hidaka, K. Nabae, M. Kawabe and M. Kitano, Inhibition by licorice flavonoid oil of glutathione S-transferase–positive foci in the medium-term rat hepatocarcinogenesis bioassay, Nutr. Res., 2010, 30(1), 74–81 CrossRef CAS PubMed.
- J. R. Macías-Pérez, O. Beltrán-Ramírez, V. R. Vásquez-Garzón, M. E. Salcido-Neyoy, P. A. Martínez-Soriano and M. B. Ruiz-Sánchez,
et al., The effect of caffeic acid phenethyl ester analogues in a modified resistant hepatocyte model, Anticancer Drugs, 2013, 24(4), 394–405 CrossRef PubMed.
- F. Ferk, W. W. Huber, B. Grasl-Kraupp, K. Speer, S. Buchmann and R. Bohacek,
et al., Protective effects of coffee against induction of DNA damage and pre-neoplastic foci by aflatoxin B1, Mol. Nutr. Food Res., 2014, 58(2), 229–238 CAS.
- H. Enzmann, K. Brunnemann, M. Iatropoulos, S. Shpyleva, N. Lukyanova and I. Todor,
et al., Inter-laboratory comparison of turkey in ovo carcinogenicity assessment (IOCA) of hepatocarcinogens, Exp. Toxicol. Pathol., 2013, 65(6), 729–735 CrossRef CAS PubMed.
-
M. Reataza and D. K. Imagawa, Advances in managing hepatocellular carcinoma, Frontiers of Medicine in China, 2014, vol. 8, pp. 175–189 Search PubMed.
-
http://www.ema.europa.eu/docs/en_GB/document_library/EPAR_-_Product_Information/human/000690/WC500027704.pdf
.
- M. Stotz, A. Gerger, J. Haybaeck, T. Kiesslich, M. D. Bullock and M. Pichler, Molecular targeted therapies in hepatocellular carcinoma: Past, present and future, Anticancer Res., 2015, 35(11), 5737–5744 CAS.
- L. Marelli, R. Stigliano, C. Triantos, M. Senzolo, E. Cholongitas and N. Davies,
et al., Transarterial therapy for hepatocellular carcinoma: Which technique is more effective? A systematic review of cohort and randomized studies, Cardiovasc Intervent Radiol, 2007, 30, 6–25 CrossRef PubMed.
-
Romeis Mikroskopische Technik, ed. M. Mulisch and U. Welsch, Spektrum Akademischer Verlag, Heidelberg, 2010 Search PubMed.
- T. D. Pugh, J. H. King, H. Koen, D. Nychka, J. Chover and G. Wahba,
et al., Reliable stereological method for estimating the number of microscopic hepatocellular foci from their transections, Cancer Res., 1983, 43(3), 1261–1268 CAS.
- H. Enzmann, L. Edler and P. Bannasch, Simple elementary method for the quantification of focal liver lesions induced by carcinogens, Carcinogenesis, 1987, 8(2), 231–235 CrossRef CAS PubMed.
- P. Bannasch, Pathobiology of chemical hepatocarcinogenesis: Recent progress and perspectives. Part II. Metabolic and molecular changes, J. Gastroenterol. Hepatol., 1990, 5(3), 310–320 CrossRef CAS PubMed.
- P. Bannasch, U. Jahn, H. Hacker, Q. Su, W. Hoffmann and R. Pichlmayr,
et al., Focal hepatic glycogenosis, Int. J. Oncol., 1997, 10, 261–268 CAS.
- H. G. Enzmann, K. D. Brunnemann, B. Kaestner, M. J. Iatropoulos and G. M. Williams, Dose-dependent induction of preneoplastic lesions by the tobacco-specific nitrosamine carcinogen NNK in the in ovo carcinogenicity assessment (IOCA) assay, Exp. Toxicol. Pathol., 2014, 66(1), 35–40 CrossRef CAS PubMed.
- G. M. Williams and R. S. Yamamoto, Absence of stainable iron from preneoplastic and neoplastic lesions in rat liver with 8-hydroxyquinoline-induced siderosis, J. Natl. Cancer Inst., 1972, 49(3), 685–692 CAS.
- G. M. Williams, M. Klaiber, S. E. Parker and E. Farber, Nature of early appearing, carcinogen-induced liver lesions to iron accumulation, J. Natl. Cancer Inst., 1976, 57(1), 157–165 CAS.
- T. Terada and Y. Nakanuma, Iron-negative foci in siderotic macroregenerative nodules in human cirrhotic liver. A marker of incipient neoplastic lesions, Arch. Pathol. Lab. Med., 1989, 113(8), 916–920 CAS.
- Y. M. Deugnier, P. Charalambous, D. Le Quilleuc, B. Turlin, J. Searle and P. Brissot,
et al., Preneoplastic significance of hepatic iron–free foci in genetic hemochromatosis: A study of 185 patients, Hepatology, 1993, 18(6), 1363–1369 CAS.
- G. A. Asare, A. C. Paterson, M. C. Kew, S. Khan and K. S. Mossanda, Iron-free neoplastic nodules and hepatocellular carcinoma without cirrhosis in Wistar rats fed a diet high in iron, J. Pathol., 2006, 208(1), 82–90 CrossRef CAS PubMed.
- H. Zerban, S. Radig, A. Kopp-Schneider and P. Bannasch, Cell proliferation and cell death (apoptosis) in hepatic preneoplasia and neoplasia are closely related to phenotypic cellular diversity and instability, Carcinogenesis, 1994, 15(11), 2467–2473 CrossRef CAS PubMed.
- J.-H. Zhong, L. Ma and L.-Q. Li, Postoperative therapy options for hepatocellular carcinoma, Scand. J. Gastroenterol, 2014, 49(6), 649–661 CrossRef CAS PubMed.
- J. Bruix, T. Takayama, V. Mazzaferro, G.-Y. Chau, J. Yang and M. Kudo,
et al., Adjuvant sorafenib for hepatocellular carcinoma after resection or ablation (STORM): a phase 3, randomised, double-blind, placebo-controlled trial, Lancet Oncol., 2015, 16(13), 1344–1354 CrossRef CAS PubMed.
- W.-J. Jeng, C.-C. Lin, W.-T. Chen, I.-S. Sheen, C.-Y. Lin and S.-M. Lin, Adjuvant therapy for hepatocellular carcinoma after curative treatment, Dig. Dis. Sci., 2014, 32(6), 747–754 CrossRef PubMed.
- J. T. Stefano, I. V. A. Pereira, M. M. Torres, P. M. Bida, A. M. M. Coelho and M. P. Xerfan,
et al., Sorafenib prevents liver fibrosis in a non-alcoholic steatohepatitis (NASH) rodent model, Braz. J. Med. Biol. Res., 2015, 48(5), 408–414 CrossRef CAS PubMed.
- T. K. Hei and O. Sudilovsky, Effects of a high-sucrose diet on the development of enzyme-altered foci in chemical hepatocarcinogenesis in rats, Cancer Res., 1985, 45(6), 2700–2705 CAS.
- H. Enzmann, D. Ohlhauser, T. Dettler and P. Bannasch, Enhancement of hepatocarcinogenesis in rats by dietary fructose, Carcinogenesis, 1989, 10(7), 1247–1252 CrossRef CAS PubMed.
- R. Kumamoto, H. Uto, K. Oda, R. Ibusuki, S. Tanoue and S. Arima,
et al., Dietary fructose enhances the incidence of precancerous hepatocytes induced by administration of diethylnitrosamine in rat, Eur. J. Med. Res., 2013, 18(1), 54 CrossRef PubMed.
- P. Bannasch, Glycogenotic hepatocellular carcinoma with glycogen-ground-glass hepatocytes: A heuristically highly relevant phenotype, World J. Gastroenterol., 2012, 18(46), 6701–6708 CrossRef PubMed.
- V. Tesori, A. C. Piscaglia, D. Samengo, M. Barba, C. Bernardini and R. Scatena,
et al., The multikinase inhibitor Sorafenib enhances glycolysis and synergizes with glycolysis blockade for cancer cell killing, Sci. Rep., 2015, 5, 9149 CrossRef PubMed.
Footnote |
† This research did not receive any specific grant from funding agencies in the public, commercial, or not-for-profit sectors. |
|
This journal is © The Royal Society of Chemistry 2017 |