DOI:
10.1039/C7SC01093A
(Edge Article)
Chem. Sci., 2017,
8, 4511-4516
Enantioselective γ-borylation of unsaturated amides and stereoretentive Suzuki–Miyaura cross-coupling†
Received
9th March 2017
, Accepted 19th April 2017
First published on 24th April 2017
Abstract
The rhodium-catalyzed, directed catalytic asymmetric hydroboration of γ,δ-unsaturated amides affords a direct route to chiral acyclic secondary γ-borylated carbonyl derivatives in high enantiomeric purity. In contrast to a similar β-borylated amide derivative, the γ-borylated amide undergoes Suzuki–Miyaura cross-coupling with stereoretention. The utility of the boronic ester products is further illustrated by other stereospecific C–B bond transformations leading to γ-amino acid derivatives, 1,4-amino alcohols, and 5-substituted-γ-lactone and γ-lactam ring systems.
Introduction
Chiral boronic esters are valuable synthetic intermediates for a variety of stereospecific transformations.1–3 Consequently, an assortment of enantioselective routes for their preparation are under active development.4–19 We have focused on the rhodium-catalyzed catalytic asymmetric hydroboration (CAHB) of β,γ-unsaturated amides,20 esters,20a and oxime ethers21 for the preparation of functionalized chiral boronic esters. For example, disubstituted alkenes such as (E)-1 undergo highly enantioselective β-borylation by pinacolborane (pinBH) when catalyzed by the combination of (R)-L1 with [Rh(nbd)2BF4] (i.e., 2
:
1 L1
:
Rh). CAHB followed by oxidation of the C–B bond affords β-hydroxy amide (S)-2 in an enantiomeric ratio (er) greater than 99
:
1 (Fig. 1).20e
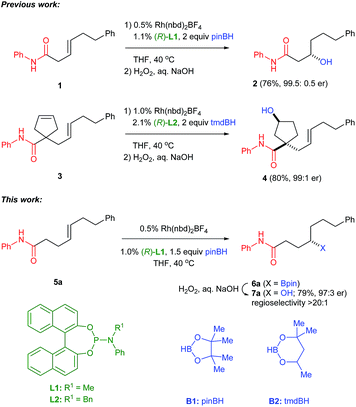 |
| Fig. 1 CAHB/oxidation of β,γ- versus γ,δ-unsaturated amides. | |
In an effort to expand the scope of CAHB, γ,δ-unsaturated amides such as 3 were explored.22 Such substrates differ from 1 in a number of important ways including, (i) the alkene is more remote than in the β,γ-unsaturated amides, (ii) substrate 3 contains two distinctly different alkene moieties, although each is ostensibly positioned γ,δ- with respect to the carbonyl directing group, and (iii) enantioselective borylation of the endocyclic alkene requires controlling the stereochemical course by desymmetrization rather than π-facial discrimination, a fundamentally different set of requirements.23 In the event, substrate 3 undergoes CAHB/oxidation to give the monounsaturated γ-hydroxy amide (1S,3S)-4 in high yield (80%), regioselectivity (>20
:
1) and enantioselectivity (99
:
1 er). Only the endocyclic double bond in 3 undergoes reaction; the pendant acyclic alkene is untouched. We now report the efficient, regioselective γ-borylation of a similarly disposed alkene in γ,δ-unsaturated amide 5a (Fig. 1). CAHB by pinBH (0.5% [Rh(nbd)2BF4/2 (R)-L1]) followed by oxidation affords chiral γ-hydroxy amide (S)-7a in 79% overall yield (97
:
3 er). While conjugate addition11 and C–H activation10a methodologies provide efficient alternatives to CAHB for enantioselective β-borylation of carbonyl compounds, direct γ-borylation is unique to CAHB.
Results and discussion
The γ,δ-unsaturated amides (E)-5b–n shown in Fig. 2, along with several related structures, were treated with the catalyst system for γ-borylation (i.e., 2
:
1 combinations of L1 or L2 with [Rh(nbd)2BF4], 0.5 mol% unless otherwise noted). In addition to phenyl amide 5a described above, the corresponding Weinreb amide 5b, the morpholine amide245c and benzyl amide 5d undergo CAHB to afford the intermediate γ-borylated amides 6b–d with high levels of enantioselectivity. Within this series of amides, the secondary amides (i.e., N-phenyl and N-benzyl) give the highest γ-selectivity (>20
:
1). Oxidations of 6b–d afford the corresponding chiral γ-hydroxy amides 7b–d (≥94
:
6 er). CAHBs of 5c and 5d were carried out on gram scale giving chiral boronic esters 6c (82%, 94.5
:
5.5) and 6d (81%, 96.5
:
3.5 er) in good yield and without loss of enantioselectivity. CAHB/oxidation of an isomeric (Z)-alkene, benzyl amide (Z)-5d, affords results similar to those obtained with the (E)-isomer.
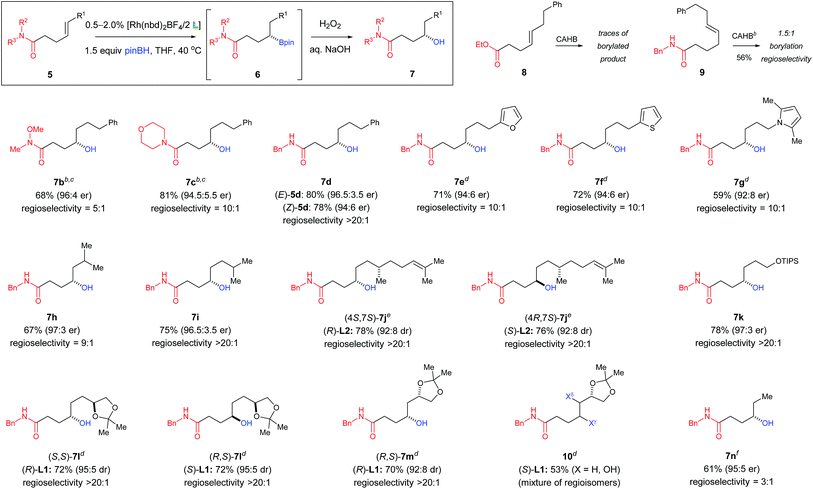 |
| Fig. 2 Substrate scope for CAHB of γ,δ-unsaturated amides. aUnless otherwise noted all reaction use 0.5% [Rh(nbd)2BF4/2 (R)-L1], 1.5 equiv. pinBH, THF, 40 °C (12 h) followed by oxidation using H2O2/aq. NaOH. Unless otherwise noted, the isolated yield is that of the major regioisomer and reflects the average of three experiments generally exhibiting a spread of ±2%; regioselectivity is determined from the crude 1H NMR of 7. Enantiomer ratios (er) are determined by chiral HPLC analysis; diastereomer ratios (dr) are determined for the purified mixture of diastereomers by integrating major and minor 13C NMR resonances. b2.0% [Rh(nbd)2BF4/2 (R)-L1]. cOxidation conditions: NaBO3/H2O. d1.0% [Rh(nbd)2BF4/2 L1]; (R)-L1 is used unless noted otherwise in the figure. e1.0% [Rh(nbd)2BF4/2 L2]. fer is determined by 19F NMR of the corresponding Mosher ester (see ESI for details†). | |
In contrast to amides 5a–d, the analogous ester 8 is largely recovered unchanged upon attempted CAHB; only trace amounts of borylated products are identified along with some evidence for alkene isomerization. δ,ε-Unsaturated amide 9, a one-carbon homologue of benzyl amide (E)-5d, is found to be considerably less reactive and less selective. Complete consumption of starting material requires 2% catalyst loading to afford 56% yield of a mixture of borylated products.25
Benzyl amides 5e–g containing heteroaromatic ring systems are nonetheless good substrates under the standard CAHB/oxidation conditions yielding 7e–g, respectively. Certain branched alkyl substituents (i.e., 5h–j) are also well tolerated. In particular, the chiral substrate (E)-5j demonstrates that (i) the proximal disubstituted alkene with respect to the amide directing group undergoes CAHB while the more distal trisubstituted alkene is untouched and (ii) the stereochemical course of γ-borylation is efficiently catalyst controlled. CAHB/oxidation with (R)-L2 affords (4S,7S)-7j; (S)-L2 affords (4R,7S)-7j. The silyl ether moiety in 5k is tolerated and affords 7k (78%, 97
:
3 er). Chiral acetal 5l again undergoes catalyst controlled γ-borylation with high diastereoselectivity; (R)-L1 affords (S,S)-7l (72%, 95
:
5 dr); (S)-L1 affords (R,S)-7l in the same yield and diastereomer ratio. However, substrate 5m, in which the chiral acetal moiety is in closer proximity to the site of hydroboration, shows a strong matched/mismatched effect. While (R)-L1 affords (R,S)-7m (70%, 92
:
8 dr), the catalyst employing (S)-L1 gives rise to a complex mixture of regioisomers 10. Substrate 5n (R1 = Me) also exhibits only modest regioselectivity (3
:
1), perhaps due to the size of the vinyl substituent compared to other derivatives described above; however, CAHB proceeds in good yield and high enantioselectivity (61%, 95
:
5 er).
Having developed an efficient method for the γ-borylation of γ,δ-unsaturated amides, Suzuki–Miyaura cross-coupling of 11 was examined (Fig. 3). Stereochemical aspects of the palladium-catalyzed cross-coupling of chiral secondary organoboron derivatives have recently attracted a great deal attention. Molander,26 Suginome,27 and Hall28 reported that β-borylated carbonyl derivatives 12–14, whether as the boronic ester or the trifluoroborate salt, undergo cross-coupling with stereoinversion. The stereochemical course is rationalized by intramolecular coordination between the carbonyl oxygen and the boron atom of the boronic ester or the partially hydrolyzed trifluoroborate. The intramolecular coordination promotes invertive transmetallation resulting in overall stereoinversion for cross-coupling. Biscoe19a,29 also found stereoinversion for simple substrates lacking functionality needed for coordination to boron during the course of transmetallation (e.g., 15). On the other hand, Suginome30 reported that boracyclic intermediate 16 undergoes cross-coupling with stereoretention. Similarly, Morken31 reported that 17 undergoes hydroxyl-directed, inner-sphere, retentive transmetallation and overall cross-coupling with stereoretention. However, when the hydroxyl is one-carbon further removed, 18 fails to undergo cross-coupling under the otherwise same conditions. We have previously shown that 19 undergoes cross-coupling with overall stereoretention.22
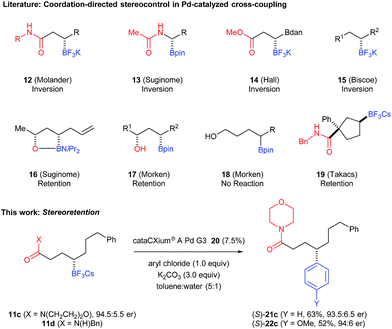 |
| Fig. 3 Stereochemical course of Suzuki–Miyaura cross-coupling with chiral secondary boronic esters or trifluoroborate salts. | |
Chiral boronic ester 6c (i.e., the morpholine amide) was converted to its corresponding trifluoroborate salt 11c32 and subjected to palladium-catalyzed cross-coupling using the Buchwald cataCXium® A Pd G3 (20) precatalyst.22,33 Cross-coupling with chlorobenzene yields amide (S)-21c (63%); 4-chloroanisole yields (S)-22c (52%). The products are obtained with essentially complete overall stereoretention.34 We find that the nature of the amide is important to the success of the cross-coupling. In contrast to the tertiary morpholine amide, the analogous secondary amide 11d does not undergo cross-coupling under the conditions employed for 11c. Hall et al.28a reported that β-boronic esters of secondary amides failed to cross-couple in cases where the corresponding tertiary amide coupled smoothly.
Chiral organoboronates are useful for a variety of other stereospecific transformations. Fig. 4 illustrates several examples starting from chiral boronic esters 6b–d; the latter are isolated in 69–82% yield from the corresponding alkenes. Treating 6b with H2O2/aq. NaOH affords the known chiral 5-substituted-γ-lactone 23 (95%).35 As an alternative to palladium-catalyzed cross-coupling, the morpholine amide derivative 6c undergoes stereoretentive cross-coupling with 2-lithiothiofuran under the conditions developed by Aggarwal2e to give 24c (84%). Compound 6c also undergoes BCl3-assisted amination with benzyl azide under the conditions reported by Knochel36 to form the γ-amino acid derivative 25c (65%). Phenyl azide also serves as a good nucleophile in such amination reactions, and 6c is converted to the corresponding N-phenyl γ-amino acid en route to the 5-substituted-γ-lactam 26 (68%) by acid catalyzed cyclization. Benzyl amide derivative 6d is efficiently converted to 1,4-aminoalcohol 27d after oxidation of the C–B bond followed by amide reduction with LAH (94%). While the secondary N-benzyl amide 11d failed in the attempted palladium-catalyzed cross-coupling described above, 6d undergoes efficient vinylation in a sequence initiated the by treatment with excess vinyl Grignard;2h amide 28d is formed in high yield (93%).
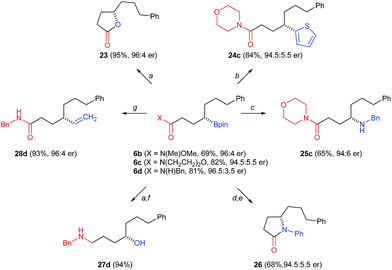 |
| Fig. 4 Stereospecific transformations of organoboranes 6b–d. Conditions: (a) NaOH/H2O2; (b) (i) n-BuLi, thiophene, (ii) NBS; (c) BCl3, BnN3; (d) BCl3, PhN3; (e) 6 M HCl; (f) LiAlH4; (g) (i) vinylMgBr, (ii) I2/NaOMe. | |
Conclusions
γ,δ-Unsaturated secondary (i.e., N-phenyl and N-benzyl) and tertiary (i.e., Weinreb and morpholine) amides undergo efficient rhodium-catalyzed CAHB to afford γ-borylated derivatives in good yield and with high levels of asymmetric induction; enantioselectivity as high as 97
:
3 er is observed. While two good alternative methods are available to prepare chiral secondary β-borylated carbonyl compounds, the present method of directed-CAHB provides to our knowledge the first direct route to chiral acyclic secondary γ-borylated carbonyl compounds with high regio- and enantioselectivity.
A previous study found β- and γ-borylation of related substrates differ in the sense of stereoinduction, i.e., π-facial discrimination.20a However, it is not the case in the present study; β-borylation of β,γ-unsaturated amide 1 and γ-borylation of the one-carbon homologue γ,δ-unsaturated amide 5 add to the same face of the alkene. In the present study, CAHB of a substrate bearing both di- and trisubstituted alkene moieties (i.e., 5j) occurs only on the disubstituted double bond proximal to the carbonyl group. Chiral substrates 5j and 5l undergo highly diastereoselective CAHB with catalyst control; however, substrate 5m, in which the resident oxygen-bearing stereocenter resides adjacent to the alkene, exhibits a strong matched and mismatched effect with enantiomeric catalysts.
The γ-borylated products are found to undergo stereoretentive palladium-catalyzed Suzuki–Miyaura cross-coupling, presumably via amide-directed inner-sphere stereoretentive transmetallation, as well as stereoretentive C–B to C–C transformations using main group organometallic reagents (e.g., lithium and magnesium). In addition, a variety of other stereospecific transformations are highlighted by the conversions of chiral, secondary γ-boronic esters 6b–d to 1,4-amino alcohols, γ-amino acid derivatives, and 5-substituted-γ-lactone and γ-lactam ring systems. Further studies are in progress.
Acknowledgements
Funding from the NIH National Institutes of General Medical Sciences (R01-GM100101) is gratefully acknowledged. We thank S. Zhang (UNL) for some preliminary experiments.
Notes and references
- For selected recent reviews, see:
(a) C.-Y. Wang, J. Derosa and M. R. Biscoe, Chem. Sci., 2015, 6, 5105 RSC;
(b) L. Xu, S. Zhang and P. Li, Chem. Soc. Rev., 2015, 44, 8848 RSC;
(c) A. H. Cherney, N. T. Kadunce and S. E. Reisman, Chem. Rev., 2015, 115, 9587 CrossRef CAS PubMed;
(d) D. Leonori and V. K. Aggarwal, Angew. Chem., Int. Ed., 2015, 54, 1082 CrossRef CAS PubMed.
-
(a) Y. Wang, A. Noble, E. L. Myers and V. K. Aggarwal, Angew. Chem., Int. Ed., 2016, 55, 4270 CrossRef CAS PubMed;
(b) A. Noble, S. Roesner and V. K. Aggarwal, Angew. Chem., Int. Ed., 2016, 55, 15920 CrossRef CAS PubMed;
(c) A. Fawcett, D. Nitsch, M. Ali, J. M. Bateman, E. L. Myers and V. K. Aggarwal, Angew. Chem., Int. Ed., 2016, 55, 14663 CrossRef CAS PubMed;
(d) A. Millán, J. R. Smith, J. L.-Y. Chen and V. K. Aggarwal, Angew. Chem., Int. Ed., 2016, 55, 2498 CrossRef PubMed;
(e) M. Odachowski, A. Bonet, S. Essafi, P. Conti-Ramsden, J. N. Harvey, D. Leonori and V. K. Aggarwal, J. Am. Chem. Soc., 2016, 138, 9521 CrossRef CAS PubMed;
(f) J. Llaveria, D. Leonori and V. K. Aggarwal, J. Am. Chem. Soc., 2015, 137, 10958 CrossRef CAS PubMed;
(g) C. Sandford, R. Rasappan and V. K. Aggarwal, J. Am. Chem. Soc., 2015, 137, 10100 CrossRef CAS PubMed;
(h) R. P. Sonawane, V. Jheengut, C. Rabalakos, R. Larouche-Gauthier, H. K. Scott and V. K. Aggarwal, Angew. Chem., Int. Ed., 2011, 50, 3760 CrossRef CAS PubMed.
-
(a) J. Schmidt, J. Choi, A. T. Liu, M. Slusarczyk and G. C. Fu, Science, 2016, 354, 1265 CrossRef CAS PubMed;
(b) B. Potter, E. K. Edelstein and J. P. Morken, Org. Lett., 2016, 18, 3286 CrossRef CAS PubMed;
(c) T. Rybak and D. G. Hall, Org. Lett., 2015, 17, 4156 CrossRef CAS PubMed;
(d) S. C. Matthew, B. W. Glasspoole, P. Eisenberger and C. M. Crudden, J. Am. Chem. Soc., 2014, 136, 5828 CrossRef CAS PubMed;
(e) A. W. Buesking and J. A. Ellman, Chem. Sci., 2014, 5, 1983 RSC;
(f) D. Imao, B. W. Glasspoole, V. S. Laberge and C. M. Crudden, J. Am. Chem. Soc., 2009, 131, 5024 CrossRef CAS PubMed.
- Stoichiometric reagents: J. M. Brown and B. N. Nguyen, Stereoselective hydroboration and diboration of carbon–carbon double bonds, Georg Thieme Verlag, 2011, vol. 1, p. 295 Search PubMed.
- For a review on recent advances in the preparation and application of allylboron species, see: C. Diner and K. J. Szabó, J. Am. Chem. Soc., 2017, 139, 2 CrossRef CAS PubMed.
- Enantioselective allyl- and arylborylation:
(a) H. M. Nelson, B. D. Williams, J. Miró and F. D. Toste, J. Am. Chem. Soc., 2015, 137, 3213 CrossRef CAS PubMed;
(b) T. Jia, P. Cao, B. Wang, Y. Lou, X. Yin, M. Wang and J. Liao, J. Am. Chem. Soc., 2015, 137, 13760 CrossRef CAS PubMed.
- Enantioselective allylic substitution with diborylmethane:
(a) Y. Shi and A. H. Hoveyda, Angew. Chem., Int. Ed., 2016, 55, 3455 CrossRef CAS PubMed;
(b) M. Zhan, R.-Z. Li, Z.-D. Mou, C.-G. Cao, J. Liu, Y.-W. Chen and D. Niu, ACS Catal., 2016, 6, 3381 CrossRef CAS.
- Enantioselective aminoborylation:
(a) D. Nishikawa, K. Hirano and M. Miura, Org. Lett., 2016, 18, 4856 CrossRef CAS PubMed;
(b) N. Matsuda, K. Hirano, T. Satoh and M. Miura, J. Am. Chem. Soc., 2013, 135, 4934 CrossRef CAS PubMed;
(c) K. Hong and J. P. Morken, J. Am. Chem. Soc., 2013, 135, 9252 CrossRef CAS PubMed.
- Enantioselective B–H bond insertion:
(a) D. Chen, X. Zhang, W.-Y. Qi, B. Xu and M.-H. Xu, J. Am. Chem. Soc., 2015, 137, 5268 CrossRef CAS PubMed;
(b) Q.-Q. Cheng, S.-F. Zhu, Y.-Z. Zhang, X.-L. Xie and Q.-L. Zhou, J. Am. Chem. Soc., 2013, 135, 14094 CrossRef CAS PubMed.
- Enantioselective C–H borylation:
(a) J. He, Q. Shao, Q. Wu and J.-Q. Yu, J. Am. Chem. Soc., 2017, 139, 3344 CrossRef CAS PubMed;
(b) L.-L. Li, Z.-L. Tao, Z.-Y. Han and L.-Z. Gong, Org. Lett., 2017, 19, 102 CrossRef CAS PubMed.
- Enantioselective conjugate borylation:
(a) Q. Jiang, T. Guo and Z. Yu, J. Org. Chem., 2017, 82, 1951 CrossRef CAS PubMed;
(b) C. Jarava-Barrera, A. Parra, A. López, F. Cruz-Acosta, D. Collado-Sanz, D. J. Cárdenas and M. Tortosa, ACS Catal., 2016, 6, 442 CrossRef CAS PubMed;
(c) J.-B. Xie, S. Lin, S. Qiao and G. Li, Org. Lett., 2016, 18, 3926 CrossRef CAS PubMed;
(d) Z. Niu, J. Chen, Z. Chen, M. Ma, C. Song and Y. Ma, J. Org. Chem., 2015, 80, 602 CrossRef CAS PubMed;
(e) H. Wu, J. M. Garcia, F. Haeffner, S. Radomkit, A. R. Zhugralin and A. H. Hoveyda, J. Am. Chem. Soc., 2015, 137, 10585 CrossRef CAS PubMed;
(f) S. Radomkit and A. H. Hoveyda, Angew. Chem., Int. Ed., 2014, 53, 3387 CrossRef CAS PubMed;
(g) Y. Luo, I. D. Roy, A. G. E. Madec and H. W. Lam, Angew. Chem., Int. Ed., 2014, 53, 4186 CrossRef CAS PubMed.
- Enantioselective conjunctive cross-coupling:
(a) G. J. Lovinger, M. D. Aparece and J. P. Morken, J. Am. Chem. Soc., 2017, 139, 3153 CrossRef CAS PubMed;
(b) L. Zhang, G. J. Lovinger, E. K. Edelstein, A. A. Szymaniak, M. P. Chierchia and J. P. Morken, Science, 2016, 351, 70 CrossRef CAS PubMed.
- Enantioselective diboration:
(a) L. Fang, L. Yan, F. Haeffner and J. P. Morken, J. Am. Chem. Soc., 2016, 138, 2508 CrossRef CAS PubMed;
(b) Z. Yu, R. J. Ely and J. P. Morken, Angew. Chem., Int. Ed., 2014, 53, 9632 CrossRef CAS PubMed;
(c) J. R. Coombs, F. Haeffner, L. T. Kliman and J. P. Morken, J. Am. Chem. Soc., 2013, 135, 11222 CrossRef CAS PubMed;
(d) G. E. Ferris, K. Hong, I. A. Roundtree and J. P. Morken, J. Am. Chem. Soc., 2013, 135, 2501 CrossRef CAS PubMed.
- Stereospecific catalytic Miyaura borylation:
(a) Q. Zhou, H. D. Srinivas, S. Zhang and M. P. Watson, J. Am. Chem. Soc., 2016, 138, 11989 CrossRef CAS PubMed;
(b) C. H. Basch, K. M. Cobb and M. P. Watson, Org. Lett., 2016, 18, 136 CrossRef CAS PubMed.
- Enantioselective nucleophilic addition to aldehyde:
(a) M. V. Joannou, B. S. Moyer and S. J. Meek, J. Am. Chem. Soc., 2015, 137, 6176 CrossRef CAS PubMed;
(b) K. Kubota, E. Yamamoto and H. Ito, J. Am. Chem. Soc., 2015, 137, 420 CrossRef CAS PubMed.
- Group-selective cross-coupling of diboranes:
(a) S. N. Mlynarski, C. H. Schuster and J. P. Morken, Nature, 2014, 505, 386 CrossRef CAS PubMed;
(b) C. Sun, B. Potter and J. P. Morken, J. Am. Chem. Soc., 2014, 136, 6534 CrossRef CAS PubMed;
(c) R. D. Dewhurst and T. B. Marder, Nat. Chem., 2014, 6, 279 CrossRef CAS PubMed.
- Enantioselective homologation of organoboron via lithiation–borylation:
(a) A. Varela, L. K. B. Garve, D. Leonori and V. K. Aggarwal, Angew. Chem., Int. Ed., 2017, 56, 2127 CrossRef CAS PubMed;
(b) D. J. Blair, D. Tanini, J. M. Bateman, H. K. Scott, E. L. Myers and V. K. Aggarwal, Chem. Sci., 2017, 8, 2898 RSC;
(c) For a review on lithiation–borylation methodology and its application in synthesis, see: D. Leonori and V. K. Aggarwal, Acc. Chem. Res., 2014, 47, 3174 CrossRef CAS PubMed;
(d) For related computational studies, see: S. Essafi, S. Tomasi, V. K. Aggarwal and J. N. Harvey, J. Org. Chem., 2014, 79, 12148 CrossRef CAS PubMed.
- From vinylboronates:
(a) J. T. Han, W. J. Jang, N. Kim and J. Yun, J. Am. Chem. Soc., 2016, 138, 15146 CrossRef CAS PubMed;
(b) D. Nishikawa, K. Hirano and M. Miura, J. Am. Chem. Soc., 2015, 137, 15620 CrossRef CAS PubMed;
(c) J. J. Verendel, O. Pamies, M. Dieguez and P. G. Andersson, Chem. Rev., 2014, 114, 2130 CrossRef CAS PubMed.
- Examples of CAHB by copper catalysts (a–j), cobalt catalysts (k–m), iron catalysts (n), iridium catalysts (o–p), and rhodium catalysts (q–r):
(a) M. G. Ceinos, A. Parra, V. M. Heras and M. Tortosa, Angew. Chem., Int. Ed., 2016, 55, 6969 CrossRef PubMed;
(b) Y. Xi and J. F. Hartwig, J. Am. Chem. Soc., 2016, 138, 6703 CrossRef CAS PubMed;
(c) D. Wang, P. Cao, B. Wang, T. Jia, Y. Lou, M. Wang and J. Liao, Org. Lett., 2015, 17, 2420 CrossRef CAS PubMed;
(d) A. Parra, L. Amenós, M. Guisán-Ceinos, A. López, J. L. G. Ruano and M. Tortosa, J. Am. Chem. Soc., 2014, 136, 15833 CrossRef CAS PubMed;
(e) Z.-T. He, Y.-S. Zhao, P. Tian, C.-C. Wang, H.-Q. Dong and G.-Q. Lin, Org. Lett., 2014, 16, 1426 CrossRef CAS PubMed;
(f) X. Feng, H. Jeon and J. Yun, Angew. Chem., Int. Ed., 2013, 52, 3989 CrossRef CAS PubMed;
(g) D. Noh, S. K. Yoon, J. Won, J. Y. Lee and J. Yun, Chem.–Asian J., 2011, 6, 1967 CrossRef CAS PubMed;
(h) Y. Sasaki, C. Zhong, M. Sawamura and H. Ito, J. Am. Chem. Soc., 2010, 132, 1226 CrossRef CAS PubMed;
(i) D. Noh, H. Chea, J. Ju and J. Yun, Angew. Chem., Int. Ed., 2009, 48, 6062 CrossRef CAS PubMed;
(j) R. Corberán, N. W. Mszar and A. H. Hoveyda, Angew. Chem., Int. Ed., 2011, 50, 7079 CrossRef PubMed;
(k) H. Zhang and Z. Lu, ACS Catal., 2016, 6, 6596 CrossRef CAS;
(l) L. Zhang, Z. Zuo, X. Wan and Z. Huang, J. Am. Chem. Soc., 2014, 136, 15501 CrossRef CAS PubMed;
(m) J. Chen, T. Xi, X. Ren, B. Cheng, J. Guo and Z. Lu, Org. Chem. Front., 2014, 1, 1306 RSC;
(n) J. Chen, T. Xi and Z. Lu, Org. Lett., 2014, 16, 6452 CrossRef CAS PubMed;
(o) M. Magre, M. Biosca, O. Pàmies and M. Diéguez, ChemCatChem, 2015, 7, 114 CrossRef CAS;
(p) C. Mazet and D. Gérard, Chem. Commun., 2011, 47, 298 RSC;
(q) N. Hu, G. Zhao, Y. Zhang, X. Liu, G. Li and W. Tang, J. Am. Chem. Soc., 2015, 137, 6746 CrossRef CAS PubMed;
(r) S. A. Moteki, K. Toyama, Z. Liu, J. Ma, A. E. Holmes and J. M. Takacs, Chem. Commun., 2012, 48, 263 RSC.
-
(a) S. M. Smith, G. L. Hoang, R. Pal, M. O. B. Khaled, L. S. W. Pelter, X. C. Zeng and J. M. Takacs, Chem. Commun., 2012, 48, 12180 RSC;
(b) S. M. Smith, M. Uteuliyev and J. M. Takacs, Chem. Commun., 2011, 47, 7812 RSC;
(c) S. M. Smith and J. M. Takacs, Org. Lett., 2010, 12, 4612 CrossRef CAS PubMed;
(d) S. M. Smith and J. M. Takacs, J. Am. Chem. Soc., 2010, 132, 1740 CrossRef CAS PubMed;
(e) S. M. Smith, N. C. Thacker and J. M. Takacs, J. Am. Chem. Soc., 2008, 130, 3734 CrossRef CAS PubMed.
- V. M. Shoba, N. C. Thacker, A. J. Bochat and J. M. Takacs, Angew. Chem., Int. Ed., 2016, 55, 1465 CrossRef CAS PubMed.
- G. L. Hoang, Z.-D. Yang, S. M. Smith, R. Pal, J. L. Miska, D. E. Pérez, L. S. W. Pelter, X. C. Zeng and J. M. Takacs, Org. Lett., 2015, 17, 940 CrossRef CAS PubMed.
- The symmetry in substrate 3 was also exploited in a DFT study of potential catalytic cycle for the reaction; see: Z.-D. Yang, R. Pal, G. L. Hoang, X. C. Zeng and J. M. Takacs, ACS Catal., 2014, 4, 763 CrossRef CAS PubMed.
- R. Peters, P. Waldmeier and A. Joncour, Org. Process Res. Dev., 2005, 9, 508 CrossRef CAS.
- When reacted separately substrate 9 requires higher catalyst loading for complete consumption as stated above (i.e., 2.0% Rh for 9versus 0.5% for 5d). However, the direct competition of 9 and 5d for limiting pinBH (equivalent amounts of each) affords only a modest excess of recovered 9 (74% recovered) relative to recovered 5d (61% recovered). The results suggest that 5d is consumed only slightly faster than 9 by active catalyst. A relatively slow conversion of the rhodium precatalyst to active catalyst by 9 in the absence of 5d could account of the conflicting observations; see ref. 22 for a similar example.
- D. L. Sandrock, L. Jean-Gerard, C. Chen, S. D. Dreher and G. A. Molander, J. Am. Chem. Soc., 2010, 132, 17108 CrossRef CAS PubMed.
-
(a) T. Awano, T. Ohmura and M. Suginome, J. Am. Chem. Soc., 2011, 133, 20738 CrossRef CAS PubMed;
(b) T. Ohmura, T. Awano and M. Suginome, J. Am. Chem. Soc., 2010, 132, 13191 CrossRef CAS PubMed.
-
(a) J. C. H. Lee, H.-Y. Sun and D. G. Hall, J. Org. Chem., 2015, 80, 7134 CrossRef CAS PubMed;
(b) J. C. Lee, R. McDonald and D. G. Hall, Nat. Chem., 2011, 3, 894 CrossRef CAS PubMed.
- L. Li, S. Zhao, A. Joshi-Pangu, M. Diane and M. R. Biscoe, J. Am. Chem. Soc., 2014, 136, 14027 CrossRef CAS PubMed.
- M. Daini and M. Suginome, J. Am. Chem. Soc., 2011, 133, 4758 CrossRef CAS PubMed.
- T. P. Blaisdell and J. P. Morken, J. Am. Chem. Soc., 2015, 137, 8712 CrossRef CAS PubMed.
- A. J. J. Lennox and G. Lloyd-Jones, Angew. Chem., Int. Ed., 2012, 51, 9385 CrossRef CAS PubMed.
- N. C. Bruno, M. T. Tudge and S. L. Buchwald, Chem. Sci., 2013, 4, 916 RSC.
- Authentic (R)-21c was prepared via enolate allylation using the Evans chiral auxiliary: in situ half reduction to aldehyde followed by Wadsworth–Horner–Emmons olefination, Pd/C hydrogenation and transamidation with morpholine is described in the accompanying ESI.†.
-
(a) C. H. Larsen, B. H. Ridway, J. T. Shaw, D. M. Smith and K. A. Woerpel, J. Am. Chem. Soc., 2005, 127, 10879 CrossRef CAS PubMed;
(b) M. Aquino, S. Cardani, G. Fronza, C. Fuganti, R. P. Fernandez and A. Tagliani, Tetrahedron, 1991, 47, 7887 CrossRef CAS.
-
(a) E. Hupe, I. Marek and P. Knochel, Org. Lett., 2002, 4, 2681 CrossRef , see also;
(b) P. Y. Chavant, F. Lhermitte and M. Vaultier, Synlett, 1993, 519 CrossRef CAS;
(c) D. S. Matteson and G. Y. Kim, Org. Lett., 2002, 4, 2153 CrossRef CAS PubMed;
(d) V. Bagutski, T. G. Elford and V. K. Aggarwal, Angew. Chem., Int. Ed., 2011, 50, 1080 CrossRef CAS PubMed.
Footnote |
† Electronic supplementary information (ESI) available: Catalyst optimization data; experimental procedures; compound characterization data; spectra. See DOI: 10.1039/c7sc01093a |
|
This journal is © The Royal Society of Chemistry 2017 |