DOI:
10.1039/C7SC00831G
(Edge Article)
Chem. Sci., 2017,
8, 4431-4436
3-Cyanoallyl boronates are versatile building blocks in the synthesis of polysubstituted thiophenes†
Received
22nd February 2017
, Accepted 11th April 2017
First published on 18th April 2017
Abstract
We report the preparation of hitherto unprecedented 3-cyanoallyl boronates using condensation of the parent α-boryl aldehyde and nitriles. The resulting allyl boronates have been used to generate a wide range of borylated thiophenes, which represent a valuable class of heterocycles in modern drug discovery. Subsequent Suzuki–Miyaura cross-coupling enabled the synthesis of pharmaceutically important 3,5-disubstituted aminothiophenes. Moreover, late stage functionalization gave access to borylated bromothiophene and thieno[2,3-b]pyridines.
Allylboronates1 are among the most widely used building blocks in organic synthesis and are commonly employed in drug discovery. Despite the widespread application of allylboron reagents in chemical synthesis, their use has been largely limited to the corresponding allylation reactions, which typically utilize electron-rich allylboranes or boronates. In contrast, the preparation and application of electron-poor allylboronates has not received enough attention in organic synthesis to date. Most of the electron-withdrawing groups have been limited to the halogens2 or their position was restricted at C-2.3 We have come across a single example4 wherein a C-3 amide-containing allylboronate was isolated as a byproduct in 35% yield. Attempts were made to access the C-3 ester-containing electron-poor allylboronates, but both5 failed to give desired products. We wondered whether the C-3 substituted electron-poor allylboronates could be generally obtained from amphoteric α-(MIDA)boryl aldehydes,6 as MIDA-protected boron species showed enhanced stability7 that allow quick and facile access to previously inaccessible boron compounds.6f If such allylboronates were accessible, the four-carbon unit arising from these allylboronate species could serve as the foundation in the synthesis of heterocycles (Scheme 1). To the best of our knowledge, heterocycle annulation from allylboronates has not received attention in synthesis. The boron motif found in the borylated heterocycle derivatives could enable late-stage cross-coupling with suitable partners or provide a handle for site-selective appendage of a boron-containing heterocycle using recently described protocols.6d
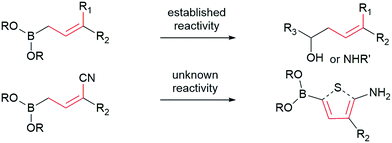 |
| Scheme 1 Contrasting typical reactivity of allyl boronates in allylation reactions with synthesis of heterocycles. | |
Results and discussion
We commenced our study with the preparation of substituted 3-cyanoallyl boronates by reacting the α-boryl aldehyde6c with nitrile derivatives. Malononitrile (3a) and ethyl cyanoacetate (3b) were first examined. We have determined that the highest yields were obtained when the Knoevenagel condensation was carried out in acetonitrile with diethylamine as the base (Table 1). Other organic bases, such as imidazole, triethylamine, morpholine, and piperidine gave low yields or no products. Under the optimized reaction conditions, the 3-cyanoallyl boronates were isolated in excellent yields (91% for 3a and 93% for 3b). Benzyl amide group was also tested and the reaction was clean, giving compound 3c in 84% yield after isolation. Cyanoacetamide was well tolerated (3d), showing that the presence of the –NH2 group does not negatively affect the reaction outcome. For compounds 3b, 3c and 3d, only one isomer was obtained from the reaction mixture and the geometry of the double bond was determined by NOESY experiments. However, the condensation reaction with cyanoacetic acid (R = COOH) resulted in complex mixture. The resulting allylboronates, bearing two electron-withdrawing groups at the terminus, have not been reported in the literature and would be difficult to access by other methods. With the successful preparation of these 3-cyanoallyl boronates, we pursued their application in the synthesis of polysubstituted borylated thiophenes.
Table 1 Synthesis of substituted 3-cyanoallyl boronates
Polysubstituted thiophenes received attention as valuable building blocks in organic synthesis.8 They have been widely used in the pharmaceutical industry,9 dye chemistry,10 and as functional materials.11 In modern drug discovery, polysubstituted thiophenes are important because they constitute a bioisosteric replacement12 for the phenyl ring. Thiophene-containing derivatives are often characterized by reduced toxicity and better pharmacokinetic properties.13 Substituted 2-aminothiophenes are one of the most important thiophene categories14 as they are common structures in the FDA approved drugs (Fig. 1).
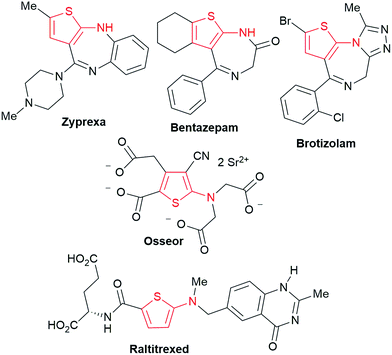 |
| Fig. 1 Examples of 2-aminothiophene-containing pharmaceutical drugs. | |
Depending on the functional groups tolerance and the stability of the products, borylated thiophenes are commonly synthesized through halogen–metal exchange15 on the preformed halothiophenes. Recent C–H activation16 with iridium catalysts showed considerable advantages, but is still restricted by the presence of directing groups and functional group tolerance. Borylation of aminothiophenes, on the other hand, is an appealing alternative to these methods that has received limited attention. This can partially be attributed to the protodeborylation reaction of the resulting products16a,17 as well as to the detrimental effect of the amino group. To the best of our knowledge, the reported examples only describe tertiary amine or amide-containing thiophenes.18 Therefore, a general method for making borylated aminothiophenes is highly desirable.
Our 3-cyanoallyl boron species could be readily converted to borylated thiophenes in the presence of elemental sulfur (the Gewald reaction). When treated with sulfur and Et2NH in THF, compounds 3a and 3b could be smoothly transformed to borylated thiophenes. We have also developed a more convenient one-pot process (Table 2). The reaction was best done at a relatively low concentration, no more than 0.05 M, to suppress the dimerization of the 3-cyanoallyl boronates intermediates.19 A variety of nitrile compounds were tested and the reactions were generally good with isolated yields varying from 33% to 91%. The electron density of the aromatic ring has a small effect on the reaction, as electron-rich phenyl (4l) gave lower yield (80%) while electron-poor (4e, 4f) phenyl examples gave better yields (91% and 89%). Heteroaryl nitriles such as thiophene (4g), furane (4i), pyrrole (4k) were also suitable and some susceptible groups were well tolerated (4d, 4h). It is noteworthy that all the products (4a to 4l) are new and many of them cannot be easily made using alternative methods. Larger scale synthesis was also desirable. With that in mind, compound 4c was obtained in 87% on a 0.5 g scale. The reaction was fast and TLC showed the complete consumption of the α-boryl aldehyde component in 3 hours. However, the sulfonyl example (4j) turned out to be more difficult to synthesize, requiring longer reaction time (24 h) and excess amount of (phenylsulfonyl)acetonitrile. The low yield of 4j was attributed to the dimerization of the allylboronate intermediate. Most of the borylated aminothiophenes are partially water-soluble, thus aqueous work-up should be avoided to achieve highest yields. Additionally, we were pleased that no C–B bond scission was observed under the standard reaction conditions and the products are stable under ambient conditions.
Table 2 Synthesis of borylated thiophenes
We sought to demonstrate our borylated aminothiophenes as intermediates to access other synthetically challenging borylated thiophene derivatives. A recent study showed that borylated bromothiophenes are promising monomers in material science due to their ability to polymerize under suitable Suzuki–Miyaura conditions.20 Our method offers a convenient route to previously inaccessible bromothiophene derivatives. Compound 4d was reacted with t-BuONO and CuBr2 in acetonitrile to generate borylated bromothiophenes 5a (Scheme 2), which was difficult to synthesize by alternative methods,21 in 63% yield.
 |
| Scheme 2 Synthesis of borylated bromothiophenes. | |
It is known that 2-heteroaryl boronates are unfavorable substrates for Suzuki–Miyaura coupling due to the tendency of protodeborylation,16a and the cross-coupling of borylated aminothiophenes could be even more challenging because of the interfering adjacent –NH2 group. To achieve reasonable yields of the cross-coupling reaction, an extensive screen of reaction conditions was carried out. For most of the screened conditions, mainly protodeborylation product was observed. Fortunately, it was found that with 0.1 equiv. RuPhos Pd G3 as the catalyst and 3.0 equiv. Na2CO3 as the base, the desired products 6a and 6b were obtained in 78% and 71% yields (Table 3). Though 3,5-disubstituted aminothiophenes can be generally prepared by Gewald reaction from suitable substituted acetaldehydes, our Suzuki approach gave access to 3,5-disubstituted variants, for which the corresponding acetaldehyde derivatives are not readily available (6c, 6d and 6e).22
Table 3 Suzuki–Miyaura-cross-coupling of aminothiophenes
Our borylated thiophenes also proved to be useful building blocks in the synthesis of borylated bisheterocycle. Recent studies23 showed that substituted thieno[2,3-b]pyridine is an important motif in the medicinal chemistry. Borylation of thieno[2,3-b]pyridines has been underexplored and to the best of our knowledge, there are no previous publications covering this topic. We wondered if the borylated thieno[2,3-b]pyridines could be made by condensing borylated aminothiophene with ketones. We initiated our study by treating 4c with cyclohexanone, using trimethylchlorosilane24 as the Lewis acid (Table 4). The cyclization reaction was finished in 1 hour and 8a was isolated in excellent yield (95%). Several ketones were examined and it was found that the reaction was influenced by the steric effect. Hindered ketones (8c, 8e) require extended reaction time (12 hours) and yields are relatively lower while cyclic ketones (7a, 7b) gave excellent yields, regardless of the ring size. No protodeborylation was observed during the reaction progress for all borylated starting material and products, even though high temperature (100 °C) was required for the transformation.
Table 4 Synthesis of borylated thieno[2,3-b]pyridines
Conclusions
In summary, we have successfully developed a series of stable, previously inaccessible 3-cyanoallyl boronates. These compounds have allowed us to generate a series of borylated thiophenes in good to excellent yields. The utility of the resulting thiophene products as key intermediates toward synthetically challenging borylated bromothiophene and thieno[2,3-b]pyridines has been demonstrated. The successful cross-coupling of borylated aminothiophenes gave access to 3,5-disubstituted aminothiophenes, which are of interest in medicinal chemistry. Further applications of electron-poor allylboronates in synthesis are now enabled and are under intense investigation in our laboratory.
Acknowledgements
We thank the Natural Science and Engineering Research Council (NSERC) for funding. S. J. K. thanks Ontario Graduate Scholarship for funding. Dr Milan Bergeron-Brlek is also thanked for discussion.
Notes and references
-
(a) Y. Yamamoto and N. Asao, Chem. Rev., 1993, 93, 2207–2293 CrossRef CAS;
(b) H. Hugo and D. G. Hall, Org. React., 2008, 73, 1 Search PubMed;
(c)
Asymmetric Synthesis, ed. M. Christmann and S. Braese, Wiley-VCH, Weinheim, 2008 Search PubMed;
(d)
Boronic Acids: Preparation, Applications in Organic Synthesis and Medicine, ed. D. G. Hall, Wiley-VCH, Weinheim, 2005 Search PubMed;
(e) M. Yus, J. C. Gonzalez-Gomez and F. Foubelo, Chem. Rev., 2013, 113, 5595–5698 CrossRef CAS PubMed;
(f) I. Marek and G. Sklute, Chem. Commun., 2007, 48, 1683–1691 RSC;
(g) T. R. Ramadhar and R. A. Batey, Synthesis, 2011, 9, 1321–1346 Search PubMed;
(h) J. W. Kennedy and D. G. Hall, Angew. Chem., Int. Ed., 2003, 42, 4732–4739 CrossRef CAS PubMed.
-
(a) C. Hertweck and W. Boland, J. Org. Chem., 2000, 65, 2458–2463 CrossRef CAS PubMed;
(b) P. V. Ramachandran and A. Chatterjee, Org. Lett., 2008, 10, 1195–1198 CrossRef CAS PubMed;
(c) V. Rauniyar and D. G. Hall, J. Org. Chem., 2009, 74, 4236–4241 CrossRef CAS PubMed;
(d) R. Corberan, N. W. Mszar and A. H. Hoveyda, Angew. Chem., Int. Ed., 2011, 50, 7079–7082 CrossRef CAS PubMed;
(e) M. J. Koh, T. T. Nguyen, H. Zhang, R. R. Schrock and A. H. Hoveyda, Nature, 2016, 531, 459–465 CrossRef CAS PubMed.
-
(a) J. W. J. Kennedy and D. G. Hall, J. Am. Chem. Soc., 2002, 124, 898–899 CrossRef CAS PubMed;
(b) F.-Y. Yang, M. Shanmugasundaram, S.-Y. Chuang, P.-J. Ku, M.-Y. Wu and C.-H. Cheng, J. Am. Chem. Soc., 2003, 125, 12576–12583 CrossRef CAS PubMed.
- A. Hercouet, F. Berre, C. H. Lin, L. Toupet and B. Carboni, Org. Lett., 2007, 9, 1717–1720 CrossRef CAS PubMed.
-
(a) J. R. Falck, M. Bondlela, J. Ye and S.-D. Cho, Tetrahedron Lett., 1999, 40, 5647–5650 CrossRef CAS;
(b) J. Kister, D. H. Ess and W. R. Roush, Org. Lett., 2013, 15, 5436–5439 CrossRef CAS PubMed.
-
(a) Z. He, A. Zajdlik and A. K. Yudin, Acc. Chem. Res., 2014, 47, 1029–1040 CrossRef CAS PubMed;
(b) J. D. St Denis, C. C. Scully, C. F. Lee and A. K. Yudin, Org. Lett., 2014, 16, 1338–1341 CrossRef CAS PubMed;
(c) J. D. St Denis, A. Zajdlik, J. Tan, P. Trinchera, C. F. Lee, Z. He, S. Adachi and A. K. Yudin, J. Am. Chem. Soc., 2014, 136, 17669–17673 CrossRef CAS PubMed;
(d) S. Adachi, S. K. Liew, C. F. Lee, A. Lough, Z. He, J. D. St Denis, G. Poda and A. K. Yudin, Org. Lett., 2015, 17, 5594–5597 CrossRef CAS PubMed;
(e) J. D. St Denis, Z. He and A. K. Yudin, ACS Catal., 2015, 5, 5373–5379 CrossRef CAS;
(f) Z. He and A. K. Yudin, J. Am. Chem. Soc., 2011, 133, 13770–13773 CrossRef CAS PubMed;
(g) P. Trinchera, V. B. Corless and A. K. Yudin, Angew. Chem., Int. Ed., 2015, 54, 9038–9041 CrossRef CAS PubMed.
-
(a) E. P. Gillis and M. D. Burke, Aldrichimica Acta, 2009, 42, 17–27 CAS;
(b) J. Li, S. G. Ballmer, E. P. Gillis, S. Fujii, M. J. Schmidt, A. M. E. Palazzolo, J. W. Lehmann, G. F. Morehouse and M. D. Burke, Science, 2015, 347, 1221–1226 CrossRef CAS PubMed;
(c) G. R. Dick, E. M. Woerly and M. D. Burke, Angew. Chem., Int. Ed., 2012, 51, 2667–2672 CrossRef CAS PubMed;
(d) E. P. Gillis and M. D. Burke, J. Am. Chem. Soc., 2007, 129, 6716–6717 CrossRef CAS PubMed;
(e) D. M. Knapp, E. P. Gillis and M. D. Burke, J. Am. Chem. Soc., 2009, 131, 6961–6963 CrossRef CAS PubMed.
-
(a) B. H. Lipshutz, Chem. Rev., 1986, 86, 795–819 CrossRef CAS;
(b) G. Rassu, F. Zanardi, L. Battistini and G. Casiraghi, Chem. Soc. Rev., 2000, 29, 109–118 RSC.
- M. Paris, M. Porcelloni, M. Binaschi and D. Fattori, J. Med. Chem., 2008, 51, 1505–1529 CrossRef CAS PubMed.
- M. S. Yen and I. J. Wang, Dyes Pigm., 2004, 61, 243–250 CrossRef CAS.
-
(a) Y. Shirota, J. Mater. Chem., 2000, 10, 1–25 RSC;
(b) H. Yu, A. E. Pullen, M. G. Büschel and T. M. Swager, Angew. Chem., Int. Ed., 2004, 43, 3700–3012 CrossRef CAS PubMed;
(c) J. Roncali, Chem. Rev., 1992, 92, 711–738 CrossRef CAS;
(d) C. Li, M. Liu, N. G. Pschirer, M. Baumgarten and K. Müllen, Chem. Rev., 2010, 110, 6817–6855 CrossRef CAS PubMed;
(e) Y. Lin, Y. Li and X. Zhan, Chem. Soc. Rev., 2012, 41, 4245–4272 RSC;
(f) C. Wang, H. Dong, W. Hu, Y. Liu and D. Zhu, Chem. Rev., 2012, 112, 2208–2267 CrossRef CAS PubMed.
-
(a)
Bioisosteres in Medicinal Chemistry, ed. N. Brown, Wiley-VCH, 2012 Search PubMed;
(b) N. A. Meanwell, J. Med. Chem., 2011, 54, 2529–2591 CrossRef CAS PubMed.
- D. J. St Jeans Jr and C. Fotsch, J. Med. Chem., 2012, 55, 6002–6020 CrossRef PubMed.
-
(a) Z. Puterová, A. Krutošíková and D. Véghc, ARKIVOC, 2010, 209–242 Search PubMed;
(b) P. Slobbe, E. Ruijter and R. V. A. Orru, MedChemComm, 2012, 3, 1189–1218 RSC.
-
(a) T. Ishiyama, M. Murata and N. Miyaura, J. Org. Chem., 1995, 60, 7508–7510 CrossRef CAS;
(b) M. Murata, T. Oyama, S. Watanabe and Y. Masuda, J. Org. Chem., 2000, 65, 164–168 CrossRef CAS PubMed;
(c) C. Kleeberg, L. Dang, Z. Lin and T. B. Marder, Angew. Chem., Int. Ed., 2009, 48, 5350–5354 CrossRef CAS PubMed;
(d) C. Moldoveanu, D. A. Wilson, C. J. Wilson, P. Leowanawat, A.-M. Resemerita, C. Liu, B. M. Rosen and V. Percec, J. Org. Chem., 2010, 75, 5438–5452 CrossRef CAS PubMed;
(e) P. Leowanawat, A.-M. Resmerita, C. Moldoveanu, C. Liu, N. Zhang, D. A. Wilson, L. M. Hoang, B. M. Rosen and V. Percec, J. Org. Chem., 2010, 75, 7822–7828 CrossRef CAS PubMed;
(f) G. A. Molander, L. N. Cavalcanti and C. Garcia-Garcia, J. Org. Chem., 2013, 78, 6427–6439 CrossRef CAS PubMed;
(g) K.-T. Wong, Y.-Y. Chien, Y.-L. Liao, C.-C. Lin, M.-Y. Chou and M.-K. Leung, J. Org. Chem., 2002, 67, 1041–1044 CrossRef CAS PubMed.
-
(a) D. W. Robbins and J. F. Hartwig, Org. Lett., 2012, 14, 4266–4269 CrossRef CAS PubMed;
(b) T. M. Boller, J. M. Murphy, M. Hapke, T. Ishiyama, N. Miyaura and J. F. Hartwig, J. Am. Chem. Soc., 2005, 127, 14263–14278 CrossRef CAS PubMed;
(c) V. A. Kallenpalli, K. A. Gore, F. Shi, L. Sanchez, G. A. Chotana, S. L. Miller, R. E. Maleczka and M. R. Smith, J. Org. Chem., 2015, 80 Search PubMed;
(d) T. Ishiyama, J. Takagi, Y. Yonekawa, J. F. Hartwig and N. Miyaura, Adv. Synth. Catal., 2003, 345, 1103–1106 CrossRef CAS;
(e) P. Harrisson, J. Morris, T. B. Marder and P. G. Steel, Org. Lett., 2009, 11, 3586–3589 CrossRef CAS PubMed;
(f) G. Wang, L. Xu and P. Li, J. Am. Chem. Soc., 2015, 137, 8058–8061 CrossRef CAS PubMed;
(g) G. A. Chotana, V. A. Kallepalli, R. E. Maleczka and M. R. Smith, Tetrahedron, 2008, 64, 6103–6114 CrossRef CAS PubMed.
- A. Del Grosso, P. J. Singleton, C. A. Muryn and M. J. Ingleson, Angew. Chem., Int. Ed., 2011, 50, 2102–2106 CrossRef CAS PubMed.
-
(a) F. Di Maria, I. E. Palama, M. Baroncini, A. Barbieri, A. Bongini, R. Bizzarri, G. Gigli and G. Barbarella, Org. Biomol. Chem., 2014, 12, 1603–1610 RSC;
(b) V. Bagutski, A. Del Grosso, J. A. Carrillo, I. A. Cade, M. D. Helm, J. R. Lawson, P. J. Singleton, S. A. Solomon, T. Marcelli and M. J. Ingleson, J. Am. Chem. Soc., 2013, 135, 474–487 CrossRef CAS PubMed.
- D. M. Barnes, A. R. Haight, T. Hameury, M. A. McLaughlin, J. Mei, J. S. Tedrow and J. D. Riva Toma, Tetrahedron, 2006, 62, 11311–11319 CrossRef CAS.
- J. A. Carrillo, M. J. Ingleson and M. L. Turner, Macromolecules, 2015, 48, 979–986 CrossRef CAS.
-
(a) P. P. Khlyabich, A. E. Rudenko and B. C. Thompson, J. Polym. Sci., Part A: Polym. Chem., 2014, 52, 1055–1058 CrossRef CAS;
(b) S. Noh, N. S. Gobalasingham and B. C. Thompson, Macromolecules, 2016, 49, 6835–6845 CrossRef CAS.
- L. Aurelio, C. Valant, B. L. Flynn, P. M. Sexton, J. M. White, A. Christopoulos and P. J. Scammells, J. Med. Chem., 2010, 53, 6550–6559 CrossRef CAS PubMed.
-
(a) A. M. Bernardino, L. C. da Silva Pinheiro, C. R. Rodrigues, N. I. Loureiro, H. C. Castro, A. Lanfredi-Rangel, J. Sabatini-Lopes, J. C. Borges, J. M. Carvalho, G. A. Romeiro, V. F. Ferreira, I. C. Frugulhetti and M. A. Vannier-Santos, Bioorg. Med. Chem., 2006, 14, 5765–5770 CrossRef CAS PubMed;
(b) L. Nathan Tumey, D. H. Boschelli, J. Lee and D. Chaudhary, Bioorg. Med. Chem. Lett., 2008, 18, 4420–4423 CrossRef CAS PubMed;
(c) R. Romagnoli, P. G. Baraldi, M. Kimatrai Salvador, D. Preti, M. Aghazadeh Tabrizi, M. Bassetto, A. Brancale, E. Hamel, I. Castagliuolo, R. Bortolozzi, G. Basso and G. Viola, J. Med. Chem., 2013, 56, 2606–2618 CrossRef CAS PubMed.
-
(a) S. V. Ryabukhin, V. S. Naumchik, A. S. Plaskon, O. O. Grygorenko and A. A. Tolmachev, J. Org. Chem., 2011, 76, 5774–5781 CrossRef CAS PubMed;
(b) S. V. Ryabukhin, A. S. Plaskon, V. S. Naumchik, D. M. Volochnyuk, S. E. Pipko and A. A. Tolmachev, Heterocycles, 2007, 71, 2397–2411 CrossRef CAS.
Footnote |
† Electronic supplementary information (ESI) available. See DOI: 10.1039/c7sc00831g |
|
This journal is © The Royal Society of Chemistry 2017 |