DOI:
10.1039/C6SC03383K
(Edge Article)
Chem. Sci., 2017,
8, 1469-1473
Identification of monodentate oxazoline as a ligand for copper-promoted ortho-C–H hydroxylation and amination†
Received
30th July 2016
, Accepted 21st September 2016
First published on 26th September 2016
Abstract
The use of a weakly coordinating monodentate directing group for copper mediated ortho-hydroxylation and amination reactions allows for the identification of an external oxazoline ligand as a promoter.
Introduction
Over the past decade, directed C–H activation has emerged as a useful tool for organic synthesis.1 Diverse carbon–carbon bond and carbon–heteroatom bond forming reactions have been developed with various transition metals. In this regard, precious metals such as Pd, Rh and Ir have shown superior catalytic reactivity. Notably, Pd catalysts have demonstrated versatility in both C(sp2)–H activation and C(sp3)–H activation via different manifolds including Pd(0)/Pd(II), Pd(II)/Pd(0), Pd(II)/Pd(IV) and Pd(II)/Pd(II) catalysis.2 However, replacing these precious metals with first-row transition metals such as Fe and Cu is more desirable due to their high abundance and low toxicity.3,4 In particular, copper-mediated C–H functionalization has made rapid progress in recent years, with various C–H transformations developed via different redox manifolds corresponding to the oxidants employed. However, owing to their low reactivity, nearly all of the inexpensive metals, especially in copper mediated C–H activation reactions, rely on strongly coordinating directing groups, for example, pyridine or bidentate pyridine-based auxiliaries (Fig. 1).4,5 The advantage of weakly coordinating directing groups to form the less thermodynamically stable metallacycle, thereby kinetically facilitating the subsequent functionalization step, has been demonstrated with only precious metal catalysts.6 The use of a weakly coordinating monodentate directing group in combination with ligand acceleration remains to be demonstrated with Cu catalysts.
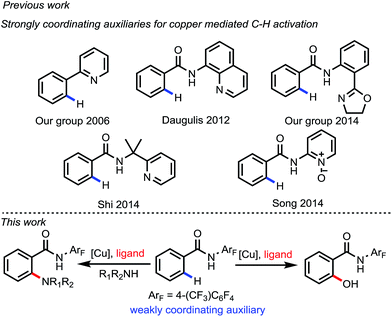 |
| Fig. 1 Auxiliaries used for copper-mediated C–H activation. | |
Phenols are a class of important structural motifs prevalent both in natural products and pharmaceuticals.7 Direct C–H hydroxylation is an appealing method for the synthesis of functionalized phenols. Pd-mediated hydroxylation of excess benzene at 180 °C was found to give phenol in less than 5% yield in an early study.8a Recently, directed ortho-C–H hydroxylation of simple substrates has reached synthetically useful yields with Pd and Ru catalysts.8,9 However, inexpensive metal-catalyzed or -mediated C–H hydroxylation reactions remain rare. In 2006, our group reported a pyridine-directed hydroxylation of inert C–H bonds using Cu(OAc)2 as a promoter, which involved the formation of acetoxylated products and subsequent hydrolysis.10 Recently, copper-mediated C–H hydroxylation of benzoic acid derivatives with the assistance of bidentate auxiliaries has also been disclosed.11 Guided by the development of Pd-catalyzed C–H activation reactions enabled by ligands, we set out to explore the combination of weakly coordinating monodentate directing groups and ligands for Cu-catalyzed C–H activation reactions. Herein, we report the first example of copper-mediated C–H hydroxylation and amination using a weakly coordinating directing group under the assistance of a monodentate ligand.
Results and discussion
Initial studies and reaction optimization
We commenced our studies with screening of a series of weakly coordinating amides (Table 1). Although displaying good reactivity in Pd-catalyzed C–H activation reactions, the N–OMe substituted amide gave no product. Other simple amides, such as N-isopropyl, N-phenyl and N-(4-NO2)-phenyl substituted amides, were also unreactive, with starting material fully recovered. Encouragingly, when N-(3,5-di-trifluoromethyl)-phenyl amide was employed as the substrate, trace product was observed. Further decreasing the electron density of the N-aryl group by using a 2,3,5,6-tetrafluoro-4-(trifluoromethyl) substituent increased the yield to 11%.12 When using 0.8 equiv. CuBr as an additive, the yield was improved to 23%. However, a side product (2a) arising from nucleophilic attack of the hydroxyl group to the C–F bond on the directing group was observed in 10% yield.
Table 1 Screening of weakly coordinating directing groupsa,b

|
Reaction conditions: 1a (0.1 mmol), Cu(OAc)2 (0.2 mmol), CsOAc (0.2 mmol), DMSO (1.0 mL), 100 °C, air, 6 h.
Yield determined by 1H NMR analysis of crude reaction mixture using CH2Br2 as an internal standard.
CuBr (0.8 eq.) as an additive.
|
|
During the past several years, we have developed two kinds of ligand, the N-protected amino acid ligand and pyridine- or quinoline-based ligand, for accelerating or promoting Pd catalyzed C(sp2)–H and C(sp3)–H bond activation.13 Based on these results, we next tested the ligand effect on this reaction (Table 2). N-protected amino acids, such as N-Ac-Leu (L1) and N-Boc-Gly (L2), and pyridine- (L4) or quinoline-based (L5) ligands are found to have negligible effect on the yield. Considering that oxazoline ligands have been demonstrated to be effective in various copper-mediated reactions, we subsequently focused on investigating theses ligands. To our delight, with ligand L6 as an additive, the yield of 3a could be increased to 33%, though it also promoted the nucleophilic attack process, correspondingly affording 2a in 17% yield. Modifying the phenyl moiety on the oxazoline ligand with a biaryl framework (L8–L11) provided no improvement. Interestingly, when employing readily available oxazoline L15 as a ligand, the yield was improved to 55%. Further optimization by changing the steric bulk and electron-donating ability of the ligands proved ineffective (L16–L19, and see ESI†).
Table 2 Ligand effect on C(sp2)–H hydroxylationa,b
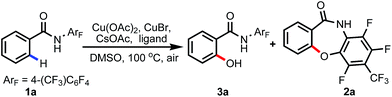
|
Reaction conditions: 1a (0.1 mmol), Cu(OAc)2 (0.2 mmol), CuBr (0.08 mmol), CsOAc (0.2 mmol), ligand (0.1 mmol), DMSO (1.0 mL), 100 °C, air, 6 h.
Yield determined by 1H NMR analysis of crude reaction mixture using CH2Br2 as an internal standard.
|
|
Having identified the optimal ligand, we next performed further optimization of the reaction conditions to suppress the undesired product 2a (Table 3). By using Cs2CO3 and PivOH as a combined additive to generate CsOPiv in situ, the yield of 3a could be increased to 59% while decreasing the yield of 2a to 12% (Table 3, entry 1). 1-Ad-COOH proved to be a better acid, which gave the product 3a in 61% yield (Table 3, entry 2). The yield could be slightly improved to 63% when increasing the amount of 1-Ad-COOH to 1.8 equiv. (Table 3, entry 3). The reaction proceeded in the presence of 1.5 equiv. Cu(OAc)2, 0.4 equiv. ligand and 0.5 mL DMSO to provide the product in 67% yield (Table 3, entry 4–6). After a brief survey of Cu(II) salts, we found that using Cu(OPiv)2 could inhibit the undesired product completely and increase the product yield to 75% (Table 3, entry 7–9). Finally, slightly increasing the temperature to 105 °C improved the yield to 80%.
Table 3 Further optimization of C(sp2)–H hydroxylationa,b
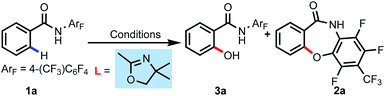
|
Entry |
Copper salts |
Base |
Acid |
Yield |
3a
|
2a
|
Reaction conditions: 1 (0.1 mmol), Cu(OAc)2 (0.2 mmol), CuBr (0.08 mmol), base (0.15 mmol), acid (0.15 mmol), ligand (0.1 mmol), DMSO (1.0 mL), 100 °C, air, 6 h.
Yield determined by 1H NMR analysis of crude reaction mixture using CH2Br2 as an internal standard.
Acid (0.18 mmol).
CuX2 (0.15 mmol).
DMSO (0.5 mL).
Ligand (0.04 mmol).
105 °C.
|
1 |
Cu(OAc)2 + CuBr |
Cs2CO3 |
PivOH |
59 |
12 |
2 |
Cu(OAc)2 + CuBr |
Cs2CO3 |
1-Ad-COOH |
61 |
13 |
3c |
Cu(OAc)2 + CuBr |
Cs2CO3 |
1-Ad-COOH |
63 |
12 |
4c,d |
Cu(OAc)2 + CuBr |
Cs2CO3 |
1-Ad-COOH |
63 |
11 |
5c,d,e |
Cu(OAc)2 + CuBr |
Cs2CO3 |
1-Ad-COOH |
66 |
8 |
6c,d,e,f |
Cu(OAc)2 + CuBr |
Cs2CO3 |
1-Ad-COOH |
67 |
8 |
7
,
,
,
|
Cu(OPiv)
2
+ CuBr
|
Cs
2
CO
3
|
1-Ad-COOH
|
75(80)
|
0
|
8c,d,e,f |
Cu(OCOiPr)2 + CuBr |
Cs2CO3 |
1-Ad-COOH |
68 |
0 |
9c,d,e,f |
Cu(OCO)2 + CuBr |
Cs2CO3 |
1-Ad-COOH |
45 |
0 |
Substrate scope
With the optimal conditions in hand, we next investigated the substrate scope (Table 4).‡ Arenes containing o-methyl and p-methyl substitutions gave yields of 41% and 65% respectively (3b and 3d), whereas an m-methyl-substituted arene afforded two regioisomers in a total of 60% yield (3c). Other electron-donating groups such as tert-butyl, methoxyl and phenyl were also well tolerated to give the corresponding products in 56–84% yields (3e–3g). An arene with m-fluoro substitution afforded a mixture of hydroxylation products at the C-2 and C-6 positions, with the sterically less hindered C-6 position as the major product (3h), while the reaction with p-fluoroarene proceeded smoothly to give the product in 84% yield (3i). Importantly, this reaction was also compatible with Cl-, Br-, and I-substituted arenes, which left synthetic handles for further functionalization (3j–3m). Arenes bearing strong electron-withdrawing functional groups underwent C–H activation efficiently, thus providing the hydroxylated products in good to excellent yields (3n–3q). Notably, this hydroxylation protocol was also compatible with a vinyl-substituted arene, which is rare in noble metal-catalyzed C–H activation reactions.
Table 4 Scope of C–H hydroxylationa,b
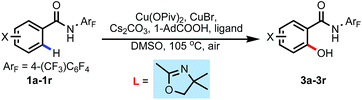
|
Reaction conditions: 1a–1r (0.1 mmol), Cu(OPiv)2 (0.15 mmol), CuBr (0.08 mmol), Cs2CO3 (0.15 mmol), 1-Ad-COOH (0.18 mmol), ligand (0.04 mmol), DMSO (0.5 mL), 105 °C, air, 6 h.
Isolated yield.
|
|
With the success of achieving C–H hydroxylation of weakly coordinating amides, we wondered whether this newly developed external ligand-promoted C–H activation protocol could be compatible with C–H amination reactions using a free alkyl amine donor, which is still an unsolved problem with weakly coordinating auxiliaries.14 To our delight, using unprotected morpholine as the amine coupling partner, the C–H amination reaction proceeded smoothly providing the desired products in moderate yields (Table 5). A variety of substituted arenes containing electron donating and electron withdrawing groups gave acceptable yields (5b–5g). Different cyclic alkyl amines also showed good compatibility with this reaction (5h–5k).
Table 5 Scope of C–H aminationa,b
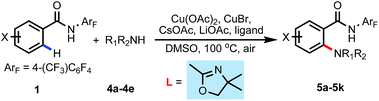
|
Reaction conditions: 1 (0.1 mmol), 4a–4e (0.3 mmol), Cu(OAc)2 (0.2 mmol), CuBr (0.1 mmol), CsOAc (0.2 mmol), LiOAc (0.1 mmol), ligand (0.05 mmol), DMSO (1.0 mL), 100 °C, air, 6 h.
Isolated yield.
|
|
Conclusions
In conclusion, we have developed a Cu-promoted C–H hydroxylation and amination of weakly coordinating amides with the assistance of an external oxazoline ligand. This finding provides guidance for further ligand development in promoting copper-catalyzed C–H activation reactions in the future.
Acknowledgements
We gratefully acknowledge the Shanghai Institute of Organic Chemistry, Chinese Academy of Sciences, the CAS/SAFEA International Partnership Program for Creative Research Teams, NSFC-21121062, NSFC-21472211, NSFC-21421091, Youth Innovation Promotion Association CAS (No.2014229), and The Recruitment Program of Global Experts for financial support. We gratefully acknowledge The Scripps Research Institute for financial support. This work was supported by NSF under the CCI Center for Selective C–H Functionalization, CHE-1205646, and Novartis for an unrestricted grant.
Notes and references
- For selected reviews on C–H functionalization using transition metals, see:
(a) F. Kakiuchi, S. Sekine, Y. Tanaka, A. Kamatani, M. Sonoda, N. Chatani and S. Murai, Bull. Chem. Soc. Jpn., 1995, 68, 62–83 CrossRef CAS;
(b) X. Chen, K. M. Engle, D.-H. Wang and J.-Q. Yu, Angew. Chem., Int. Ed., 2009, 48, 5094–5115 CrossRef CAS PubMed;
(c) R. Giri, B.-F. Shi, K. M. Engle, N. Maugel and J.-Q. Yu, Chem. Soc. Rev., 2009, 38, 3242–3272 RSC;
(d) T. W. Lyons and M. S. Sanford, Chem. Rev., 2010, 110, 1147–1169 CrossRef CAS PubMed;
(e) D. A. Colby, R. G. Bergman and J. A. Ellman, Chem. Rev., 2010, 110, 624–655 CrossRef CAS PubMed;
(f) J. Wencel-Delord, T. Dröge, F. Liu and F. Glorius, Chem. Soc. Rev., 2011, 40, 4740–4761 RSC;
(g) P. B. Arockiam, C. Bruneau and P. H. Dixneuf, Chem. Rev., 2012, 112, 5879–5918 CrossRef CAS PubMed;
(h) L. Ackermann, Acc. Chem. Res., 2014, 47, 281–295 CrossRef CAS PubMed.
- For Pd-catalyzed C–H functionalizations via various redox manifolds, see:
(a) O. Baudoin, A. Herrbach and F. Guéritte, Angew. Chem., Int. Ed., 2003, 42, 5736–5740 CrossRef CAS PubMed;
(b) L. S. Campeau, S. Rousseaux and K. Fagnou, J. Am. Chem. Soc., 2005, 127, 18020–18021 CrossRef CAS PubMed;
(c) M. Wasa, K. M. Engle and J.-Q. Yu, J. Am. Chem. Soc., 2009, 131, 9886–9887 CrossRef CAS PubMed;
(d) C. Jia, D. Piao, J. Oyamada, W. Lu, T. Kitamura and Y. Fujiwara, Science, 2000, 287, 1992–1995 CrossRef CAS PubMed;
(e) X. Chen, C. E. Goodhue and J.-Q. Yu, J. Am. Chem. Soc., 2006, 128, 12634–12635 CrossRef CAS PubMed;
(f) N. D. Ball, J. W. Kampf and M. S. Sanford, J. Am. Chem. Soc., 2010, 132, 2878–2879 CrossRef CAS PubMed;
(g) S. Ma, G. Villa, P. S. Thuy-Boun, A. Homs and J.-Q. Yu, Angew. Chem., Int. Ed., 2014, 53, 734–737 CrossRef CAS PubMed.
- For iron catalyzed sp2 and sp3 C–H functionalizations, see:
(a) A. Matsumoto, L. Ilies and E. Nakamura, J. Am. Chem. Soc., 2011, 133, 6557–6559 CrossRef CAS PubMed;
(b) S. Asako, L. Ilies and E. Nakamura, J. Am. Chem. Soc., 2013, 135, 17755–17757 CrossRef CAS PubMed;
(c) R. Shang, L. Ilies, A. Matsumoto and E. Nakamura, J. Am. Chem. Soc., 2013, 135, 6030–6032 CrossRef CAS PubMed;
(d) L. Ilies, T. Matsubara, S. Ichikawa, S. Asako and E. Nakamura, J. Am. Chem. Soc., 2014, 136, 13126–13129 CrossRef CAS PubMed;
(e) R. Shang, L. Ilies, S. Asako and E. Nakamura, J. Am. Chem. Soc., 2014, 136, 14349–14352 CrossRef CAS PubMed;
(f) R. Shang, L. Ilies and E. Nakamura, J. Am. Chem. Soc., 2015, 137, 7660–7663 CrossRef CAS PubMed.
- For recent development of Cu-catalyzed C–H functionalizations, see:
(a) G. Brasche and S. L. Buchwald, Angew. Chem., Int. Ed., 2008, 47, 1932–1934 CrossRef CAS PubMed;
(b) S. Ueda and H. Nagasawa, Angew. Chem., Int. Ed., 2008, 47, 6411–6413 CrossRef CAS PubMed;
(c) L. M. Huffman and S. S. Stahl, J. Am. Chem. Soc., 2008, 130, 9196–9197 CrossRef CAS PubMed;
(d) I. Ban, T. Sudo, T. Taniguchi and K. Itami, Org. Lett., 2008, 10, 3607–3609 CrossRef CAS PubMed;
(e) W. Wang, F. Luo, S. Zhang and J. Cheng, J. Org. Chem., 2010, 75, 2415–2418 CrossRef CAS PubMed;
(f) A. M. Suess, M. Z. Ertem, C. J. Cramer and S. S. Stahl, J. Am. Chem. Soc., 2013, 135, 9797–9804 CrossRef CAS PubMed;
(g) A. T. Parsons and S. L. Buchwald, Angew. Chem., Int. Ed., 2011, 50, 9120–9123 CrossRef CAS PubMed;
(h) A. John and K. M. Nicholas, J. Org. Chem., 2011, 76, 4158–4162 CrossRef CAS PubMed;
(i) L. D. Tran, I. Popov and O. Daugulis, J. Am. Chem. Soc., 2012, 134, 18237–18240 CrossRef CAS PubMed;
(j) Z.-K. Ni, Q. Zhang, T. Xiong, Y.-Y. Zheng, Y. Li, H.-W. Zhang, J.-P. Zhang and Q. Liu, Angew. Chem., Int. Ed., 2012, 51, 1244–1247 CrossRef CAS PubMed;
(k) J. Roane and O. Daugulis, Org. Lett., 2013, 15, 5842–5845 CrossRef CAS PubMed;
(l) J. Gallardo-Donaire and R. Martin, J. Am. Chem. Soc., 2013, 135, 9350–9353 CrossRef CAS PubMed;
(m) S. Bhadra, W. I. Dzik and L. J. Gooßen, Angew. Chem., Int. Ed., 2013, 52, 2959–2962 CrossRef CAS PubMed;
(n) M. Nishino, K. Hirano, T. Satoh and M. Miura, Angew. Chem., Int. Ed., 2013, 52, 4457–4461 CrossRef CAS PubMed;
(o) T. Truong, K. Klimovica and O. Daugulis, J. Am. Chem. Soc., 2013, 135, 9342–9345 CrossRef CAS PubMed;
(p) M. Shang, H.-L. Wang, S.-Z. Sun, H.-X. Dai and J.-Q. Yu, J. Am. Chem. Soc., 2014, 136, 11590–11593 CrossRef CAS PubMed;
(q) M. Shang, S.-Z. Sun, H.-L. Wang, B. N. Laforteza, H.-X. Dai and J.-Q. Yu, Angew. Chem., Int. Ed., 2014, 53, 10439–10442 CrossRef CAS PubMed;
(r) M. Shang, S.-Z. Sun, H.-X. Dai and J.-Q. Yu, Org. Lett., 2014, 16, 5666–5669 CrossRef CAS PubMed;
(s) J. Dong, F. Wang and J. You, Org. Lett., 2014, 16, 2884–2887 CrossRef CAS PubMed;
(t) S. Wang, R. Guo, G. Wang, S.-Y. Chen and X.-Q. Yu, Chem. Commun., 2014, 50, 12718–12721 RSC;
(u) F.-J. Chen, G. Liao, X. Li, J. Wu and B.-F. Shi, Org. Lett., 2014, 16, 5644–5647 CrossRef CAS PubMed;
(v) X.-Q. Hao, L.-J. Chen, B. Ren, L.-Y. Li, X.-Y. Yang, J.-F. Gong, J.-L. Niu and M.-P. Song, Org. Lett., 2014, 16, 1104–1107 CrossRef CAS PubMed;
(w) S. L. McDonald, C. E. Hendrick and Q. Wang, Angew. Chem., Int. Ed., 2014, 53, 4667–4670 CrossRef CAS PubMed;
(x) Z. Wang, J. Ni, Y. Ni and M. Kanai, Angew. Chem., Int. Ed., 2014, 53, 3496–3499 CrossRef CAS PubMed;
(y) Z. Wang, Y. Kuninobu and M. Kanai, Org. Lett., 2014, 16, 4790–4793 CrossRef CAS PubMed;
(z) K. Takamatsu, K. Hirano and M. Miura, Org. Lett., 2015, 17, 4066–4069 CrossRef CAS PubMed.
- For a few examples of Fe- and Co-catalyzed C–H functionalizations with a weakly coordinating DG, see:
(a) Q. Chen, L. Ilies, N. Yoshikai and E. Nakamura, Org. Lett., 2011, 13, 3232–3234 CrossRef CAS PubMed;
(b) Q. Chen, L. Ilies and E. Nakamura, J. Am. Chem. Soc., 2011, 133, 5221–5223 CrossRef PubMed;
(c) L. Ilies, E. Konno, Q. Chen and E. Nakamura, Asian J. Org. Chem., 2012, 1, 142–145 CrossRef CAS.
-
(a) K. M. Engle, T.-S. Mei, M. Wasa and J.-Q. Yu, Acc. Chem. Res., 2012, 45, 788–802 CrossRef CAS PubMed;
(b) S. D. Sarkar, W. Liu, S. I. Kozhushkov and L. Ackermann, Adv. Synth. Catal., 2014, 356, 1461–1479 CrossRef;
(c) D.-H. Wang and J.-Q. Yu, J. Am. Chem. Soc., 2011, 133, 5767–5769 CrossRef CAS PubMed;
(d) F. W. Patureau, T. Besset and F. Glorius, Angew. Chem., Int. Ed., 2011, 50, 1064–1067 CrossRef CAS PubMed;
(e) K. M. Engle, P. S. Thuy-Boun, M. Dang and J.-Q. Yu, J. Am. Chem. Soc., 2011, 133, 18183–18193 CrossRef CAS PubMed;
(f) D. Leow, G. Li, T.-S. Mei and J.-Q. Yu, Nature, 2012, 486, 518–522 CrossRef CAS PubMed;
(g) C. Grohmann, H. Wang and F. Glorius, Org. Lett., 2012, 14, 656–659 CrossRef CAS PubMed;
(h) L. Chu, K.-J. Xiao and J.-Q. Yu, Science, 2014, 346, 451–455 CrossRef CAS PubMed;
(i) Y.-J. Liu, H. Xu, W.-J. Kong, M. Shang, H.-X. Dai and J.-Q. Yu, Nature, 2014, 515, 389–393 CrossRef CAS PubMed;
(j) Z.-J. Du, J. Guan, G.-J. Wu, P. Xu, L.-X. Gao and F.-S. Han, J. Am. Chem. Soc., 2015, 137, 632–635 CrossRef CAS PubMed;
(k) S. Warratz, C. Kornhaaß, A. Cajaraville, B. Niepötter, D. Stalke and L. Ackermann, Angew. Chem., Int. Ed., 2015, 54, 5513–5517 CrossRef CAS PubMed;
(l) A. Bechtoldt, C. Tirler, K. Raghuvanshi, S. Warratz, C. Kornhaab and L. Ackermann, Angew. Chem., Int. Ed., 2016, 55, 264–267 CrossRef CAS PubMed.
-
(a)
J. H. P. Tyman, Synthetic and Natural Phenols, Elsevier, New York, 1996 Search PubMed;
(b)
Z. Rappoport, The Chemistry of Phenols, Wiley-VCH, Weinheim, 2003 CrossRef;
(c) W.-Y. Huang, Y.-Z. Cai and Y. Zhang, Nutr. Cancer, 2010, 62, 1–20 CrossRef CAS PubMed.
- For palladium-catalyzed direct hydroxylation of arenes, see:
(a) T. Jintoku, K. Nishimura, K. Takaki and Y. Fujiwara, Chem. Lett., 1991, 193–194 CAS;
(b) Y.-H. Zhang and J.-Q. Yu, J. Am. Chem. Soc., 2009, 131, 14654–14655 CrossRef CAS PubMed;
(c) G. Shan, X. Yang, L. Ma and Y. Rao, Angew. Chem., Int. Ed., 2012, 51, 13070–13074 CrossRef CAS PubMed;
(d) F. Mo, L. J. Trzepkowski and G. Dong, Angew. Chem., Int. Ed., 2012, 51, 13075–13079 CrossRef CAS PubMed;
(e) P. Y. Choy and F. Y. Kwong, Org. Lett., 2013, 15, 270–273 CrossRef CAS PubMed;
(f) Y. Yan, P. Feng, Q.-Z. Zheng, Y.-F. Liang, J.-F. Lu, Y. Cui and N. Jiao, Angew. Chem., Int. Ed., 2013, 52, 5827–5831 CrossRef CAS PubMed;
(g) H.-Y. Zhang, H.-M. Yi, G.-W. Wang, B. Yang and S.-D. Yang, Org. Lett., 2013, 15, 6186–6189 CrossRef CAS PubMed.
- For ruthenium-catalyzed direct hydroxylation of arenes, see:
(a) Y. Yang, Y. Lin and Y. Rao, Org. Lett., 2012, 14, 2874–2877 CrossRef CAS PubMed;
(b) V. S. Thirunavukkarasu, J. Hubrich and L. Ackermann, Org. Lett., 2012, 14, 4210–4213 CrossRef CAS PubMed;
(c) V. S. Thirunavukkarasu and L. Ackermann, Org. Lett., 2012, 14, 6206–6209 CrossRef CAS PubMed;
(d) F. Yang and L. Ackermann, Org. Lett., 2013, 15, 718–720 CrossRef CAS PubMed;
(e) X. Yang, G. Shan and Y. Rao, Org. Lett., 2013, 15, 2334–2337 CrossRef CAS PubMed;
(f) W. Liu and L. Ackermann, Org. Lett., 2013, 15, 3484–3486 CrossRef CAS PubMed;
(g) F. Yang, K. Rauch, K. Kettelhoit and L. Ackermann, Angew. Chem., Int. Ed., 2014, 53, 11285–11288 CrossRef CAS PubMed.
-
(a) X. Chen, X.-S. Hao, C. E. Goodhue and J.-Q. Yu, J. Am. Chem. Soc., 2006, 128, 6790–6791 CrossRef CAS PubMed;
(b) For a Cu-mediated C–H amidation see: T. Uemura, S. Imoto and N. Chatani, Chem. Lett., 2006, 35, 842–843 CrossRef CAS.
-
(a) X. Li, Y.-H. Liu, W.-J. Gu, B. Li, F.-J. Chen and B.-F. Shi, Org. Lett., 2014, 16, 3904–3907 CrossRef CAS PubMed;
(b) S.-Z. Sun, M. Shang, H.-L. Wang, H.-X. Lin, H.-X. Dai and J.-Q. Yu, J. Org. Chem., 2015, 80, 8843–8848 CrossRef CAS PubMed;
(c) B. K. Singh and R. Jana, J. Org. Chem., 2016, 81, 831–841 CrossRef CAS PubMed.
-
(a) M. Wasa, K. M. Engle and J.-Q. Yu, J. Am. Chem. Soc., 2009, 131, 9886–9887 CrossRef CAS PubMed;
(b) M. Wasa, K. M. Engle and J.-Q. Yu, J. Am. Chem. Soc., 2010, 132, 3680–3681 CrossRef CAS PubMed.
- For ligand-enabled or -promoted C–H activation reactions using Pd catalyst, see:
(a) D.-H. Wang, K. M. Engle, B.-F. Shi and J.-Q. Yu, Science, 2010, 327, 315–319 CrossRef CAS PubMed;
(b) K. M. Engle, D.-H. Wang and J.-Q. Yu, J. Am. Chem. Soc., 2010, 132, 14137–14151 CrossRef CAS PubMed;
(c) M. Wasa, K. S. L. Chan, X.-G. Zhang, J. He, M. Miura and J.-Q. Yu, J. Am. Chem. Soc., 2012, 134, 18570–18572 CrossRef CAS PubMed;
(d) J. He, S. Li, Y. Deng, H. Fu, B. N. Laforteza, J. E. Spangler, A. Homs and J.-Q. Yu, Science, 2014, 343, 1216–1220 CrossRef CAS PubMed;
(e) S. Li, G. Chen, C.-G. Feng, W. Gong and J.-Q. Yu, J. Am. Chem. Soc., 2014, 136, 5267–5270 CrossRef CAS PubMed;
(f) R.-Y. Zhu, K. Tanaka, G.-C. Li, J. He, H.-Y. Fu, S.-H. Li and J.-Q. Yu, J. Am. Chem. Soc., 2015, 137, 7067–7070 CrossRef CAS PubMed;
(g) J. Li, S. Warratz, D. Zell, S. D. Sarkar, E. E. Ishikawa and L. Ackermann, J. Am. Chem. Soc., 2015, 137, 13894–13901 CrossRef CAS PubMed;
(h) Y. Yang, X. Qiu, Y. Zhao, Y. Mu and Z. Shi, J. Am. Chem. Soc., 2016, 138, 495–498 CrossRef CAS PubMed.
- For selective examples of C–H amination reactions, see:
(a) H.-Y. Thu, W.-Y. Yu and C.-M. Che, J. Am. Chem. Soc., 2006, 128, 9048–9049 CrossRef CAS PubMed;
(b) K.-H. Ng, A. S. C. Chan and W.-Y. Yu, J. Am. Chem. Soc., 2010, 132, 12862–12864 CrossRef CAS PubMed;
(c) B. Xiao, T.-J. Gong, J. Xu, Z.-J. Liu and L. Liu, J. Am. Chem. Soc., 2011, 133, 1466–1470 CrossRef CAS PubMed;
(d) K. Sun, Y. Li, T. Xiong, J. Zhang and Q. Zhang, J. Am. Chem. Soc., 2011, 133, 1694–1697 CrossRef CAS PubMed;
(e) E. J. Yoo, S. Ma, T.-S. Mei, K. S. L. Chan and J.-Q. Yu, J. Am. Chem. Soc., 2011, 133, 7652–7655 CrossRef CAS PubMed;
(f) K.-H. Ng, Z. Zhou and W.-Y. Yu, Org. Lett., 2012, 14, 272–275 CrossRef CAS PubMed;
(g) C. Grohmann, H. Wang and F. Glorius, Org. Lett., 2012, 14, 656–659 CrossRef CAS PubMed;
(h) L. D. Tran, J. Roane and O. Daugulis, Angew. Chem., Int. Ed., 2013, 52, 6043–6046 CrossRef CAS PubMed;
(i) M. Shang, S.-Z. Sun, H.-X. Dai and J.-Q. Yu, J. Am. Chem. Soc., 2014, 136, 3354–3357 CrossRef CAS PubMed.
Footnotes |
† Electronic supplementary information (ESI) available: Data for new compounds and experimental procedures. CCDC 1496588, 1496590. For ESI and crystallographic data in CIF or other electronic format see DOI: 10.1039/c6sc03383k |
‡ General procedure for copper-promoted ortho-C–H hydroxylation: to a 15 mL sealed tube was added substrate 1 (0.1 mmol, 1.0 equiv.), Cu(OPiv)2 (0.15 mmol), CuBr (0.08 mmol), Cs2CO3 (0.15 mmol), 1-Ad-COOH (0.18 mmol), ligand L15 (0.04 mmol) and DMSO (0.5 mL). The reaction tube was then placed into a pre-heated oil bath and stirred at 105 °C for 6 h under air. Upon completion, EtOAc was added to dilute the mixture, which was then washed with NH3·H2O and saturated NaCl(aq). The organic fraction was dried over Na2SO4, evaporated and purified by preparative TLC (EtOAc/hexane) to provide the corresponding products as white solids. |
|
This journal is © The Royal Society of Chemistry 2017 |