New horizons in microbiological food safety: Photodynamic Decontamination based on a curcumin derivative†
Received
9th May 2017
, Accepted 5th October 2017
First published on 6th November 2017
Abstract
Outbreaks of foodborne diseases are regularly reported worldwide. In particular, uncooked plant food is considered risky in terms of microbiological safety. Food is also the most important transmission route for resistant microorganisms from animals to humans. Photodynamic Decontamination (PDc) of foodstuff was recently introduced as a novel approach for increasing microbiological food safety. We investigated the efficiency of PDc on plant food with different geometries (flat, spherical and complex) using a two-dimensional LED array as a light source (435 nm, 33.8 J cm−2) and the cationic curcumin derivative SACUR-3 as a photosensitiser. A photoantibacterial effect (>3 log10 CFU reduction) was achieved on all flat substrates (slices of cucumber, tomato and lettuce) with 10 μM, 50 μM or 100 μM SACUR-3. The maximal photokilling with a relative inactivation of 5.6
log10 was measured on lettuce using 50 μM of the photoactive compound. Phototreatment of non-germinated fenugreek seeds and mung beans was successful if the spherical objects were rotated while under illumination (antibacterial effect at 100 μM SACUR-3). The decontamination of mung bean germlings with a more complex geometry using the PDc approach was ineffective with the two-dimensional light source. In conclusion, PDc based on the cationic curcumin derivative SACUR-3 is very effective at improving the microbiological safety of plant food with a flat or spherical geometry. More complex objects will require the development of novel illumination devices.
Introduction
Access to safe, healthy, high quality and affordable food represents a fundamental human need. Outbreaks of foodborne diseases have been reported on every continent in the past decade, demonstrating the relevance of the development of strategies to further improve microbiological food safety. Foodborne and waterborne diarrhoeal diseases kill an estimated 2.2 million people annually, most of whom are children.1 Also, the use of antimicrobials in animal husbandry and food production has an impact on human health: exposure through food is the most important transmission route for resistant microorganisms from animals to people.2 Contamination with microbes and exchange of resistance genes among different bacteria occur at different stages along the food production chain. For example, shortly after the introduction of streptothricin as a growth promoter in 1983, a streptothricin-resistance gene in Escherichia coli was isolated from pigs. Subsequently, it was detected in E. coli from farmers, their family members, from urinary tract infections of urban citizens, and then in isolates of Salmonella and Shigella.3
Food is traded globally, which enables the worldwide dissemination of dangerous microorganisms, as evidenced by the haemolytic uraemic syndrome epidemic in 2011: fenugreek sprouts produced in Egypt acted as vehicles for Shigatoxin-producing E. coli O104:H4, causing 54 regrettable deaths in Europe and North America and inducing compensatory payments to European farmers of 200 million euros.4 Enterobacteria producing extended-spectrum β-lactamases (ESBL) in food and food-producing animals are generally identified as a public health risk due to their resistance against β-lactam antibiotics.5
Photodynamic Inactivation (PDI), a technique which uses light-activated photoactive compounds (photosensitisers, PS) to kill pathogens by the generation of reactive oxygen species (ROS),6 was recently introduced as a promising approach for food decontamination.7–9 Photosensitisers derived from natural sources, such as curcumin10 or chlorophyllin11–13 are suitable for this application due to their approval as food additives (E100 for curcumin, E140/E141 for chlorophyllin),14 which proves their excellent biocompatibility and even allows for the ingestion of the compounds together with food. They do not, however, carry a positive net charge. Addition of cell wall permeabilizers such as CaCl2, employment of complexes with macromolecular biomolecules (e.g. Chitosan12), preparation of supersaturated solutions in combination with cyclodextrin15 or usage of lyophilized solid dispersions16 represent strategies used to facilitate the docking of curcumin or chlorophyllin to the Gram(−) bacterial cell wall.17 In addition to curcumin and chlorophyllin, complexes of hypericin with β-lactoglobulin18,19 and zinc-substituted myoglobin, that forms during the maturation of non-cooked ham,20 have recently been suggested as photosensitisers for application in the dairy industry.
Effective photodecontamination of food requires the activation of the photosensitiser all-round three-dimensional targets. Currently, mainly two-dimensional LED arrays,21 halogen lamps or lasers are employed in the photodynamic inactivation of microorganisms, which provide unidirectional illumination only.
We here test a novel cationic and water soluble curcumin derivative – SACUR-3 – for its applicability in Photodynamic Decontamination (PDc) of plant food with different geometries (flat, spherical and complex) and discuss approaches to solve the problem of photosensitiser activation on three-dimensional objects. SACUR-3 was recently proved to be PDI effective against E. coli and Staphylococcus aureus in an ex vivo porcine skin model.22 According to a list published by the Center for Science in the Public Interest (CSPI), ten foods alone – the Food and Drug Administration “FDA Top Ten” – account for 40 percent of all foodborne outbreaks linked to FDA-regulated foods since 1990 (see Table 1 and ref. 23). This list served as the basis for the selection of plant food subject to PDc in our study.
Table 1 The FDA Top Ten riskiest foods regulated by the FDA, modified from ref. 23
Rank |
Food |
Outbreaks |
1 |
Leafy greens |
363 outbreaks involving 13.568 reported cases of illness |
2 |
Eggs |
352 outbreaks involving 11.163 reported cases of illness |
3 |
Tuna |
268 outbreaks involving 2.341 reported cases of illness |
4 |
Oysters |
132 outbreaks involving 3.409 reported cases of illness |
5 |
Potatoes |
108 outbreaks involving 3.659 reported cases of illness |
6 |
Cheese |
83 outbreaks involving 2.761 reported cases of illness |
7 |
Ice cream |
74 outbreaks involving 2.594 reported cases of illness |
8 |
Tomatoes |
31 outbreaks involving 3.292 reported cases of illness |
9 |
Sprouts |
31 outbreaks involving 2.022 reported cases of illness |
10 |
Berries |
25 outbreaks involving 3.397 reported cases of illness |
Experimental
Preparation of SACUR-3 stock solution
The cationic curcumin derivative SACUR-3 (Fig. 1)22,24 was kindly provided by Dr Andreas Spaeth (Institute of Organic Chemistry, University of Regensburg, Regensburg, Germany) and dissolved in ultrapure water to a final concentration of 5 mM. The stock solution was stored in the dark at −20 °C until usage.
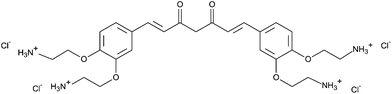 |
| Fig. 1 Chemical structure of SACUR-3. Details can be found in ref. 22 and 24. | |
Preparation of cucumbers, tomatoes, lettuce, fenugreek seeds and mung beans
All products were obtained from local food stores.
Cucumbers and tomatoes were washed with mains water (no chlorine content, pH: 7.8–7.9) and cut in plane pieces (size 1.5 × 1.5 cm). They were placed in Petri dishes with the unbroken epidermis facing up and embedded in 15% Agar–Agar (Kobe I, Carl Roth GmbH + Co. KG, Karlsruhe, Germany) as described previously.7 To remove any pre-existing microbial decontamination the samples were covered with 70% (v/v) ethanol for one minute. The ethanol was carefully removed and the samples were left to dry. Pieces of lettuce 6 × 6 cm in size were washed with water containing a 1% dishwashing solution (Aro Citrus, Goldhand, Duesseldorf, Germany), thoroughly rinsed with water and dried at room temperature. They were placed in Petri dishes without agar-fixation. Fenugreek seeds (Fig. 2A) were covered with 70% (v/v) ethanol for 3 minutes. The ethanol was removed and the samples were left to dry. Mung beans (Fig. 2B) and mung bean germlings (Fig. 2C) were used without prior decontamination. To obtain germinated mung beans the beans were placed in ultrapure sterile water and incubated overnight at room temperature. As a result of this treatment, the seeds swell, the seed coats open and a root 1–3 mm in length grows, impeding the rotation of the germlings in 12 well microplates. This is considered a complex geometry (Fig. 2C).
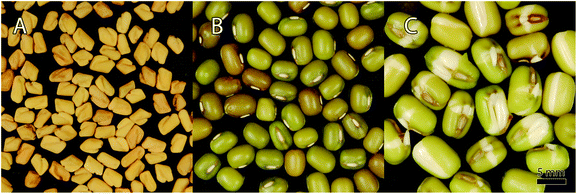 |
| Fig. 2 Photographs of fenugreek seeds (A), mung beans (B) and mung bean germlings (C). Bar represents 5 mm. | |
Bacterial culture
Escherichia coli (ATCC 25922) was grown overnight in 20 ml Todd–Hewitt–Bouillon (Roth) containing 3 g l−1 yeast-extract (AppliChem, Darmstadt, Deutschland) at 37 °C under constant agitation at 200 rpm on a shaking incubator (MaxQ 4000, Thermo Scientific, Marietta Ohio, USA). The bacterial suspension was diluted to an optical attenuation of 0.05 at λ = 600 nm (Infinite M200, Tecan, Switzerland) and incubated for 2 hours at 37 °C under constant agitation at 200 rpm. This way the bacterial culture reaches its logarithmic growth phase (the optical attenuation of about 0.3 corresponds to a CFU count of about 1 × 107). The culture was centrifuged at 830 rcf (Centrifuge 5417R, Eppendorf, Hamburg, Germany) for 3 min. The supernatant was removed and the pellet was reconstituted in DPBS (Dulbecco's Phosphate Buffered Saline, Sigma Aldrich Chemie GmbH, Steinheim, Germany).
PDI against E. coli on cucumbers, tomatoes and lettuce
Two hundred and fifty microliters of the bacterial suspension were carefully applied to the upper surface of the cucumber, tomato or lettuce using a pipette. Afterwards, 250 μl of SACUR-3 in PBS at different concentrations (10 μM, 50 μM, and 100 μM) was added to the bacterial suspension and the samples were illuminated from above with an LED-array consisting of 432 LEDs (diode type LED435-12-30,25 Roithner Lasertechnik, Vienna, Austria; dominant wavelength: 435 nm ± 10 nm).21 The distance between the diodes and the samples was 15 cm, the irradiance was 9.4 mW cm−2 (measured using a LI-189 light meter equipped with a PY pyranometer detector (LI-COR, Lincoln, Nebraska, USA)), and the radiant exposure was 33.8 J cm−2. Three controls were included: Co −/− (no light, no PS), light only (light, DPBS with no SACUR-3) and PS (no light, SACUR-3 at the highest concentration). The samples were processed using sterile cotton sticks to transfer bacteria from the surface into micro-tubes filled with DPBS. The probes were diluted in series and plated on petri dishes with Todd–Hewitt broth 1.5% agar (Roth). The plates were incubated overnight at 37 °C and analysed by counting the colony forming units (CFU). All experiments were repeated at least three times.
PDI against E. coli on fenugreek seeds, mung beans and mung bean germlings
The 2 hour culture of E. coli was centrifuged and reconstituted in DPBS. Fenugreek seeds were placed in 1.5 ml micro-tubes and covered with 1 ml of the bacterial suspension for 3 min. For each sample three seeds were transferred to the wells of a 24 well plate and 400 μl of SACUR-3 in DPBS (10 μM, 50 μM, 100 μM) was added to each well.
For the PDI on mung beans and mung bean germlings for each sample five beans or germlings were placed in micro-tubes. 250 μl of the bacterial suspension were added to the tubes. The tubes were vortexed to spread the bacterial suspension on the substrates. For each PS concentration 5 beans or germlings were placed in the wells of a 12 well plate and covered with 250 μl of SACUR-3 in DPBS.
The samples were illuminated under constant agitation from below (435 nm, 9.4 mW cm−2, and 33.8 J cm−2). The treated seeds and beans were transferred into micro-tubes filled with DPBS and vortexed vigorously. After serial dilution the probes were transferred onto THB agar plates and the colonies were counted as described above. All experiments were repeated at least three times.
Data analysis
The relative inactivation was calculated by dividing the CFU of the double negative control by the CFU of the sample for each biological replicate. All values represent the mean and standard deviation of at least three biological replicates. The black dashed line in Fig. 3–8 corresponds to a reduction of 3 log10 units as the criterion for an antibacterial effect.
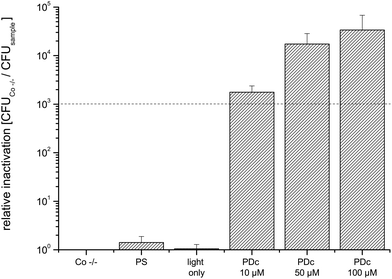 |
| Fig. 3 Photodynamic Decontamination of E. coli on cucumber shown as relative inactivation (CFUCo −/−/CFUsample). PDc was done at three different concentrations (10 μM, 50 μM, and 100 μM) of SACUR-3 using a 435 nm LED-array (radiant exposure: 33.8 J cm−2, irradiance: 9.4 mW cm−2). Counting of CFU took place 24 h after illumination. Relative inactivation was calculated for each experiment separately. The bars represent the average of three independent biological replicates including the standard deviations as error bars. The black dashed line corresponds to a reduction of 3 log10 (99.9% killing efficacy). Three controls were included in this experiment. Co −/−: double negative control; PS: dark control; and light only: light control. | |
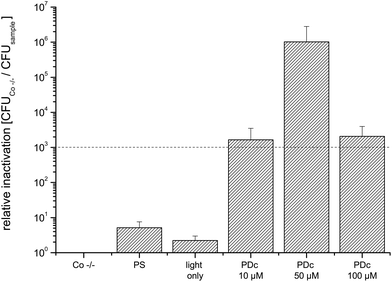 |
| Fig. 4 Photodynamic Decontamination of E. coli on tomatoes shown as relative inactivation. PDc was done at three different concentrations (10 μM, 50 μM, and 100 μM) of SACUR-3 using a 435 nm LED-array (radiant exposure: 33.8 J cm−2, irradiance: 9.4 mW cm−2). The bars represent the average of three independent biological replicates. The black dashed line corresponds to a reduction of 3 log10 (99.9% killing efficacy). Three controls were included in this experiment. Co −/−: double negative control; PS: dark control; and light only: light control. | |
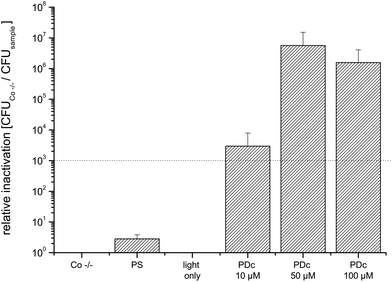 |
| Fig. 5 Results of PDc of E. coli on lettuce shown as relative inactivation (CFUCo −/−/CFUsample). PDc was done at three different concentrations (10 μM, 50 μM, and 100 μM) of SACUR-3 using a 435 nm LED-array (radiant exposure: 33.8 J cm−2, irradiance: 9.4 mW cm−2). Counting of CFU took place 24 h after illumination. Relative inactivation was calculated for each experiment separately. The bars represent the average of three independent biological replicates including the standard deviations as error bars. The black dashed line corresponds to a reduction of 3 log10 (99.9% killing efficacy). Three controls were included in this experiment. Co −/−: double negative control; PS: dark control; and light only: light control. | |
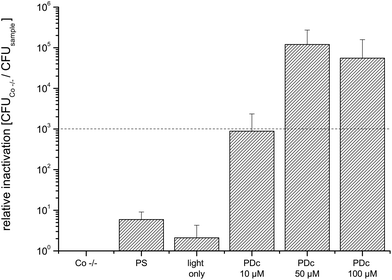 |
| Fig. 6 Results of PDc of E. coli on fenugreek seeds shown as relative inactivation (CFUCo −/−/CFUsample). PDc was done at three different concentrations (10 μM, 50 μM, and 100 μM) of SACUR-3 using a 435 nm LED-array (radiant exposure: 33.8 J cm−2, irradiance: 9.4 mW cm−2). Counting of CFU took place 24 h after illumination. Relative inactivation was calculated for each experiment separately. The bars represent the average of three independent biological replicates including the standard deviations as error bars. The black dashed line corresponds to a reduction of 3 log10 (99.9% killing efficacy). Three controls were included in this experiment. Co −/−: double negative control; PS: dark control; and light only: light control. | |
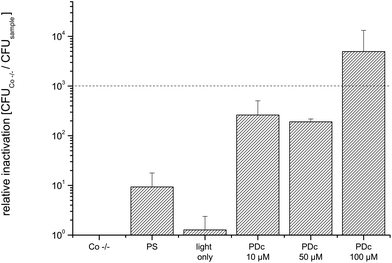 |
| Fig. 7 Results of PDc of E. coli on mung beans shown as relative inactivation (CFUCo −/−/CFUsample). PDc was done at three different concentrations (10 μM, 50 μM, and 100 μM) of SACUR-3 using a 435 nm LED-array (radiant exposure: 33.8 J cm−2, irradiance: 9.4 mW cm−2). Counting of CFU took place 24 h after illumination. Relative inactivation was calculated for each experiment separately. The bars represent the average of three independent biological replicates including the standard deviations as error bars. The black dashed line corresponds to a reduction of 3 log10 (99.9% killing efficacy). Three controls were included in this experiment. Co −/−: double negative control; PS: dark control; and light only: light control. | |
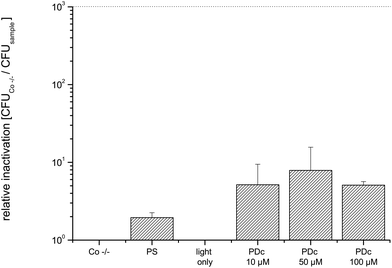 |
| Fig. 8 Results of PDc of E. coli on mung bean germlings shown as relative inactivation (CFUCo−/−/CFUsample). PDc was done at three different concentrations (10 μM, 50 μM, and 100 μM) of SACUR-3 using a 435 nm LED-array (radiant exposure: 33.8 J cm−2, irradiance: 9.4 mW cm−2). Counting of CFU took place 24 h after illumination. Relative inactivation was calculated for each experiment separately. The bars represent the average of three independent biological replicates including the standard deviations as error bars. The black dashed line corresponds to a reduction of 3 log10 (99.9% killing efficacy). Three controls were included in this experiment. Co −/−: double negative control; PS: dark control; and light only: light control. | |
Results
The numerical values of all experiments can be found in ESI Table 1.†
PDI against E. coli on flat objects: cucumber, tomato, and lettuce
The simplest geometry tested in this study is flat slices of plant food. The mean values of CFU of the controls of E. coli inoculated on cucumbers are in the same order of magnitude (8.2 × 107 for the Co −/− control). Photodynamic Decontamination with all three concentrations tested achieves an antibacterial effect (3
log10 criterion, Fig. 3). The mean CFU value of the untreated controls of PDc on tomatoes was 2.5 × 108. Again, PDc with all tested concentrations achieves an antibacterial effect against the coliform bacterial strain tested (Fig. 4); the maximum killing efficacy of six orders of magnitude is achieved at a concentration of 50 μM.
Salads and other food items containing leafy greens account for 24 percent of all of the outbreaks linked to the FDA Top Ten.23 The mean CFU of the untreated control (Co −/−) was 4.6 × 107. Phototreatment with all tested concentrations achieves a higher reduction than 3 log10 (Fig. 5). The highest killing efficacy with a relative inactivation of 5.6 × 106 is reached at 50 μM. As for cucumbers, doubling the PS concentration to 100 μM does not further increase the phototoxicity.
PDI of E. coli on spherical objects: fenugreek seeds and mung beans
Decontamination of three-dimensional objects with PDI using a flat light source is more difficult to handle, since the light has to activate the PS all-round the target. To overcome this challenge, the round fenugreek seeds and mung beans were continuously rotated by shaking.
Due to a different experimental contamination strategy when compared to that for the flat objects the CFU of untreated controls was lower (7.3 × 105). The PDc treatment of fenugreek seeds infected with E. coli with a PS concentration reaches the highest inactivation at 50 μM SACUR-3 (Fig. 6) with no further increase in photoefficiency upon doubling of the PS concentration. As a result of the bigger diameter (average: 4 mm) when compared to those of fenugreek seeds, the controls of mung beans were able to hold more bacteria: the mean value of CFU of the Co −/− was 1.4 × 106. The highest killing efficacy is reached at 100 μM (Fig. 7).
PDI of E. coli on complex objects: mung bean germlings
Mung bean sprouts are often eaten raw in salads. In this study such mung bean germlings with opened seed coats were used as examples for complex substrate geometries. The mean value of CFU of the Co −/− was about one order of magnitude higher when compared to that of the beans (9.6 × 106). The phototreatment with all tested concentrations did not result in an antibacterial effect (Fig. 8).
Discussion
The complex, globalised food system and the rise of industrial large scale production and processing have challenging implications on the control of microbiological food safety.
In consideration of the FDA Top Ten (Table 1) we here prove the principle of PDc as a valid approach for increasing the microbiological food safety of lettuce, cucumbers, tomatoes, seeds and sprouts. Cooking of the food increases food safety by the thermal inactivation of microbiological contaminations, but these plant-based and healthy foods are commonly not heat-treated before consumption to avoid the loss of the valuable nutrients and vitamins. Additionally, cooking of fruits and vegetables changes their consistency, which is unwanted. Escherichia coli, a Gram(−) coliform bacterium, serves as a model pathogen for all experiments justified by its high relevance in food poisoning. The contamination of plant food such as lettuce with E. coli has been shown to happen by the transfer from slurry fertilizer, with E. coli serving as an indicator of fecal contamination and potential bacterial enteric pathogens.26 Coliform contaminations in fertilized soil generally persist for more than 100 days after the application of manure with a decrease of ∼1 log10 per month from the initial levels of about 4 log10 CFU g−1. Photodynamic Decontamination based on a cationic derivate of curcumin induces an antibacterial effect (3 log10 criterion) at concentrations of the photoactive compound of 10 μM or higher on samples with a “flat” geometry, i.e. slices of cucumber and tomato, and leaves of lettuce. The maximal photoinactivation (5.6 × 106) is achieved with lettuce as the substrate and a concentration of 50 μM of SACUR-3. Upon doubling the amount of the photosensitiser (100 μM) the photoantibacterial effect does not increase within the experimental error, indicative of saturation with the photoactive compound. Other mechanisms such as PS aggregation may also contribute to the observed effect. SACUR-3 – in contrast to curcumin – carries four cationic charges, and therefore overcomes the limitations of its natural parent compound in inactivating Gram(−) bacteria, where cell wall permeabilisation with CaCl2 is needed to induce photokilling.10 A water-soluble formulation of curcumin has been demonstrated to be applicable for the food decontamination of Gram(+) Staphylococcus aureus,7 but it failed to kill E. coli inoculated onto porcine skin without the addition of permeabilizers.7 Other very promising strategies exist to render uncharged or negatively charged natural photosensitisers effective against Gram-negatives in food. So, for example, the group of Luksiene successfully employed a complex of chlorophyllin and chitosan to decontaminate strawberries from Gram(−) and Gram(+) food pathogens.8,11,12 Both natural compounds, curcumin (E100) and chlorophyllin (E140, E141), hold approval as food additives,14 which may prove advantageous for the rapid implementation of PDc in industrial applications. The curcumin derivate SACUR-3 lacks approval by food authorities, but its high photoefficiency against Gram(−) bacteria even without cell wall permeabilising agents and its chemical similarity to the natural parent compound renders this photosensitiser a valuable alternative to formulations of curcumin.
This study compares the antibacterial efficiency of PDc applied to food of different geometries and usage of a two-dimensional light source providing light from one direction. Slices of cucumbers, tomatoes and leaves of lettuce are considered as flat. This geometry is considered as model for convenience and trendy food such as pre-cut salads, which are usually not washed before consumption and therefore have higher requirements for hygiene and microbiological food safety.27,28
Beans and seeds represent food with a spherical – i.e. more or less round – shape. All-round illumination with the unidirectional light source was achieved by continuous shaking of the samples, which induced a rotational movement and activation of the photosensitiser on all sides of the samples. Interestingly, the efficiency of bacterial photodecontamination was higher for the smaller fenugreek seeds (maximum 1.2 × 105 at 50 μM SACUR-3) compared to that of mung beans (5.0 × 103 at 100 μM). The rotation is – among other factors – induced by the frequency and the amplitude of the shaking device. Therefore, the optimization of these parameters might even out the differences in the photoantibacterial efficiencies. The difference in the PDc-efficiency between the smaller fenugreek seeds and the somewhat bigger mung beans is not likely due to variations in the radiant exposure, as at 33.8 J cm−2 light is by far overdosed and not rate-limiting in all experiments (unpublished results).
Fenugreek seeds were identified as the vehicle for Shigatoxin-producing E. coli O104:H4, the source of a severe outbreak of food intoxication in 2011 in the EU and North America.4 Germination of seeds and consumption of home-grown sprouts are a healthy nutritional trend. However, microbial contaminants present on the seeds will multiply during germination (usually done in water over night), which renders home-grown sprouts problematic in terms of food safety. Most likely as a result of the complex geometry, the bacterial load of mung bean germlings was not significantly reduced after PDc (see Fig. 8). The rotation of the objects by shaking to allow for all-round illumination with the unidirectional light source was not effective for mung bean germlings due to swelling and growth of a root. Also, during germination the seed coats open, resulting in a more uneven surface. Therefore, the decontamination of seeds and beans is preferably done before germination. As an alternative, the challenge of a complex, three-dimensional geometry may be resolved by (i) the active and direct rotation of the object in the light field of a unidirectional light source, (ii) the rotation of the light source or (iii) the development of three-dimensional light sources for food decontamination. The construction and technical layout of such light sources allowing high-throughput PDc of alimentary products as well as the precise light dosimetry still require investigation.
Conclusion
Plant food is quite often not cooked before consumption. As a consequence, many aliments on the FDA Top Ten of riskiest food are vegetables, fruits and seeds. Photodynamic Decontamination based on the cationic curcumin derivative SACUR-3 combines the high phototoxicity against Escherichia coli of four to seven orders of magnitude in flat or round-shaped objects with high biocompatibility. Experiments on the organoleptic properties of the phototreated samples still have to prove health and safety, but PDc does not alter the optical appearance of the aliments and is therefore invisible to consumers. For the effective photodecontamination of food with a more complex geometry, the development of new illumination devices is necessary.
Abbreviations
CFU | Colony forming unit(s) |
DPBS | Dulbecco's phosphate buffered saline |
E. coli
|
Escherichia coli
|
ESBL | Extended-spectrum β-lactamases |
PDI | Photodynamic inactivation |
PDc | Photodynamic Decontamination of food |
PS | Photosensitiser |
ROS | Reactive oxygen species |
Conflicts of interest
The authors declare no competing interests.
Acknowledgements
The authors are grateful to Dr Andreas Spaeth, Institute of Organic Chemistry, University of Regensburg for providing SACUR-3 and to Barbara Krammer, University of Salzburg, for her support. Melanie Steinbauer BSc and Peter Posch BSc are acknowledged for their experimental support.
References
-
W. H. Organisation, Advancing food safety initiatives: strategic plan for food safety including foodborne zoonoses 2013–2022, 2014, p. 31 Search PubMed.
- R. Laxminarayan, A. Duse, C. Wattal, A. K. M. Zaidi, H. F. L. Wertheim, N. Sumpradit, E. Vlieghe, G. L. Hara, I. M. Gould, H. Goossens, C. Greko, A. D. So, M. Bigdeli, G. Tomson, W. Woodhouse, E. Ombaka, A. Q. Peralta, F. N. Qamar, F. Mir, S. Kariuki, Z. A. Bhutta, A. Coates, R. Bergstrom, G. D. Wright, E. D. Brown and O. Cars, Antibiotic resistance—the need for global solutions, Lancet Infect. Dis., 2013, 13, 1057–1098 CrossRef PubMed.
- W. Witte, Selective pressure by antibiotic use in livestock, Int. J. Antimicrob. Agents, 2000, 16(Supplement 1), 19–24 CrossRef.
- L. A. King, F. Nogareda, F. X. Weill, P. Mariani-Kurkdjian, E. Loukiadis, G. Gault, N. Jourdan-DaSilva, E. Bingen, M. Mace, D. Thevenot, N. Ong, C. Castor, H. Noel, D. Van Cauteren, M. Charron, V. Vaillant, B. Aldabe, V. Goulet, G. Delmas, E. Couturier, Y. Le Strat, C. Combe, Y. Delmas, F. Terrier, B. Vendrely, P. Rolland and H. de Valk, Outbreak of Shiga toxin-producing Escherichia coli O104:H4 associated with organic fenugreek sprouts, France, June 2011, Clin. Infect. Dis., 2012, 54, 1588–1594 CrossRef CAS PubMed.
- E. Liebana, A. Carattoli, T. M. Coque, H. Hasman, A. P. Magiorakos, D. Mevius, L. Peixe, L. Poirel, G. Schuepbach-Regula, K. Torneke, J. Torren-Edo, C. Torres and J. Threlfall, Public health risks of enterobacterial isolates producing extended-spectrum beta-lactamases or AmpC beta-lactamases in food and food-producing animals: an EU perspective of epidemiology, analytical methods, risk factors, and control options, Clin. Infect. Dis., 2013, 56, 1030–1037 CrossRef PubMed.
- M. Wainwright, T. Maisch, S. Nonell, K. Plaetzer, A. Almeida, G. P. Tegos and M. R. Hamblin, Photoantimicrobials - are we afraid of the light?, Lancet Infect. Dis., 2016, 17, e49–e55 CrossRef PubMed.
- N. Tortik, A. Spaeth and K. Plaetzer, Photodynamic decontamination of foodstuff from Staphylococcus aureus based on novel formulations of curcumin, Photochem. Photobiol. Sci., 2014, 13, 1402–1409 CAS.
- Z. Luksiene and L. Brovko, Antibacterial Photosensitization-Based Treatment for Food Safety, Food Eng. Rev., 2013, 5, 185–199 CrossRef CAS.
- Z. Luksiene and E. Paskeviciute, Microbial control of food-related surfaces: Na-Chlorophyllin-based photosensitization, J. Photochem. Photobiol., B, 2011, 105, 69–74 CrossRef CAS PubMed.
- S. Winter, N. Tortik, A. Kubin, B. Krammer and K. Plaetzer, Back to the roots: photodynamic inactivation of bacteria based on water-soluble curcumin bound to polyvinylpyrrolidone as a photosensitizer, Photochem. Photobiol. Sci., 2013, 12, 1795–1802 CAS.
- Z. Luksiene and E. Paskeviciute, Novel approach to the microbial decontamination of strawberries: chlorophyllin-based photosensitization, J. Appl. Microbiol., 2011, 110, 1274–1283 CrossRef CAS PubMed.
- I. Buchovec, V. Lukseviciute, A. Marsalka, I. Reklaitis and Z. Luksiene, Effective photosensitization-based inactivation of Gram (-) food pathogens and molds using the chlorophyllin-chitosan complex: towards photoactive edible coatings to preserve strawberries, Photochem. Photobiol. Sci., 2016, 15, 506–516 CAS.
- G. López-Carballo, P. Hernández-Muñoz, R. Gavara and M. J. Ocio, Photoactivated chlorophyllin-based gelatin
films and coatings to prevent microbial contamination of food products, Int. J. Food Microbiol., 2008, 126, 65–70 CrossRef PubMed.
-
T. E. Commission, Commission Regulation (EU) No 1129/2011, http://eur-lex.europa.eu/LexUriServ/LexUriServ.do?uri=OJ:L:2011:295:0001:0177:EN:PDF, (accessed 28.03.2017).
- A. B. Hegge, T. T. Nielsen, K. L. Larsen, E. Bruzell and H. H. Tønnesen, Impact of curcumin supersaturation in antibacterial photodynamic therapy—effect of cyclodextrin type and amount: Studies on curcumin and curcuminoides XLV, J. Pharm. Sci., 2012, 101, 1524–1537 CrossRef CAS PubMed.
- K. O. Wikene, A. B. Hegge, E. Bruzell and H. H. Tonnesen, Formulation and characterization of lyophilized curcumin solid dispersions for antimicrobial photodynamic therapy (aPDT): studies on curcumin and curcuminoids LII, Drug Dev. Ind. Pharm., 2015, 41, 969–977 CrossRef CAS PubMed.
- S. George, M. R. Hamblin and A. Kishen, Uptake pathways of anionic and cationic photosensitizers into bacteria, Photochem. Photobiol. Sci., 2009, 8, 788–795 CAS.
- B. Rodríguez-Amigo, P. Delcanale, G. Rotger, J. Juárez-Jiménez, S. Abbruzzetti, A. Summer, M. Agut, F. J. Luque, S. Nonell and C. Viappiani, The complex of hypericin with β-lactoglobulin has antimicrobial activity with potential applications in dairy industry, J. Dairy Sci., 2015, 98, 89–94 CrossRef PubMed.
- P. Delcanale, B. Rodriguez-Amigo, J. Juarez-Jimenez, F. J. Luque, S. Abbruzzetti, M. Agut, S. Nonell and C. Viappiani, Tuning the local solvent composition at a drug carrier surface: the effect of dimethyl sulfoxide/water mixture on the photofunctional properties of hypericin-β-lactoglobulin complexes, J. Mater. Chem. B, 2017, 5, 1633–1641 RSC.
- P. Delcanale, C. Montali, B. Rodríguez-Amigo, S. Abbruzzetti, S. Bruno, P. Bianchini, A. Diaspro, M. Agut, S. Nonell and C. Viappiani, Zinc-Substituted Myoglobin Is a Naturally Occurring Photo-antimicrobial Agent with Potential Applications in Food Decontamination, J. Agric. Food Chem., 2016, 64, 8633–8639 CrossRef CAS PubMed.
- A. Pieslinger, K. Plaetzer, C. B. Oberdanner, J. Berlanda, H. Mair, B. Krammer and T. Kiesslich, Characterization of a simple and homogeneous irradiation device based on light-emitting diodes: A possible low-cost supplement to conventional light sources for photodynamic treatment, Med. Laser Appl., 2006, 21, 277–283 CrossRef.
- N. Tortik, P. Steinbacher, T. Maisch, A. Spaeth and K. Plaetzer, A comparative study on the antibacterial photodynamic efficiency of a curcumin derivative and a formulation on a porcine skin model, Photochem. Photobiol. Sci., 2016, 15, 187–195 CAS.
-
J. W. Sarah Klein, A. Tian and C. S. DeWaal, The ten riskiest foods regulated by the U.S. Food and Drug Administration, https://cspinet.org/sites/default/files/attachment/cspi_top_10_fda.pdf, (accessed 28.03.2017).
-
A. Spaeth, K. Plaetzer, T. Maisch and A. Eichner, WIPO Pat, 2017032892A1, 2017 Search PubMed.
- LED435-12-30, http://www.datasheetlib.com/datasheet/1093158/led435-12-30_roithner-lasertechnik/download.html, (accessed 06.07.2017).
- A. N. Jensen, C. Storm, A. Forslund, D. L. Baggesen and A. Dalsgaard, Escherichia coli contamination of lettuce grown in soils amended with animal slurry, J. Food Prot., 2013, 76, 1137–1144 CrossRef CAS PubMed.
- C. L. Little and I. A. Gillespie, Prepared salads and public health, J. Appl. Microbiol., 2008, 105, 1729–1743 CrossRef CAS PubMed.
- M. Z. Jeddi, M. Yunesian, M. E. h. Gorji, N. Noori, M. R. Pourmand and G. R. J. Khaniki, Microbial Evaluation of Fresh, Minimally-processed Vegetables and Bagged Sprouts from Chain Supermarkets, J. Health Popul. Nutr., 2014, 32, 391–399 Search PubMed.
Footnote |
† Electronic supplementary information (ESI) available. See DOI: 10.1039/c7pp00165g |
|
This journal is © The Royal Society of Chemistry and Owner Societies 2017 |