Structural diversification of the aminobicyclo[4.3.0]nonane skeleton using alkynylsilyl-derived allylic trichloroacetimidates†
Received
23rd February 2017
, Accepted 13th March 2017
First published on 14th March 2017
Abstract
The amino substituted bicyclo[4.3.0]nonane is a molecular scaffold found in a wide range of natural products and medicinal agents. Despite this, synthetic methods for the general preparation of this structural motif are sparse. Here we evaluate a diastereoselective approach for the preparation of vinylsilyl derived aminobicyclo[4.3.0]nonanes using a one-pot multi-bond forming process involving a Pd(II)-catalysed Overman rearrangement, a Ru(II)-catalysed ring closing enyne metathesis reaction, followed by a hydrogen bonding directed Diels–Alder reaction. We show that a benzyldimethylsilyl-substituted alkene analogue is amenable to further functionalisation and the late stage generation of diverse sp3-rich, drug-like aminobicyclo[4.3.0]nonane scaffolds with up to six stereogenic centres.
Introduction
The amino substituted bicyclo[4.3.0]nonane core is found in various natural products,1 such as guanidine alkaloid netamine A (1),2 the antitumour antibiotics (+)-ptilocaulin (2)3 and kinamycin A (3),4 as well as the lycopodium alkaloid serratinine (4) (Fig. 1).5 Amino-indanes, a partially saturated form of the aminobicyclo[4.3.0]nonane scaffold are also found in a diverse range of pharmacologically important compounds including the monoamine transporter inhibitor (+)-indatraline (5)6 and, rasagiline (6) (Azilect), used for the treatment of Parkinson's disease.7 While there are synthetic methods for the preparation of specific aminobicyclo[4.3.0]nonanes,8 there are relatively few general strategies for the stereoselective synthesis of this scaffold that is modular, allowing late-stage structural diversification.9
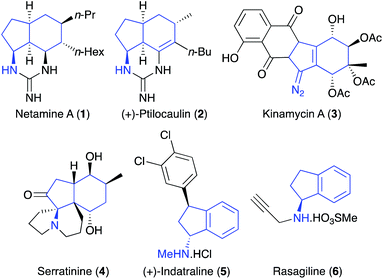 |
| Fig. 1 Biologically active aminobicyclo[4.3.0]nonanes and amino-indanes. | |
With the aim of developing new strategies for the preparation of drug-like scaffolds, we reported a one-pot, three-step multi-bond forming process of alkyne derived allylic alcohols that utilised an Overman rearrangement, a ring closing enyne metathesis (RCEYM) step and a Diels–Alder reaction for the general preparation of aminobicyclo[4.3.0]nonanes.10 More recently the diversity of this library was extended by using C-7 substituted hept-2-en-6-yn-1-ols (Scheme 1a).11 As well as yielding aminobicyclo[4.3.0]nonanes with additional functionality, the presence of a C-7 substituent within the allylic trichloroacetimidate substrate, allowed the use of mild palladium(II)-catalysed conditions for the Overman rearrangement step.12 Although diversity could be introduced into the aminobicyclo[4.3.0]nonane core during the final-stage Diels–Alder reaction, the other point of diversity was via a Sonogashira reaction during the first step. This required substantial effort to generate a small library of these compounds with various R-groups. To overcome this limitation, we decided to investigate an alternative C-7 substituent that would be compatible with the synthesis of alkyne-derived allylic trichloroacetimidates, allow a Pd(II)-catalysed Overman rearrangement and be used as a functional handle for late-stage diversification.
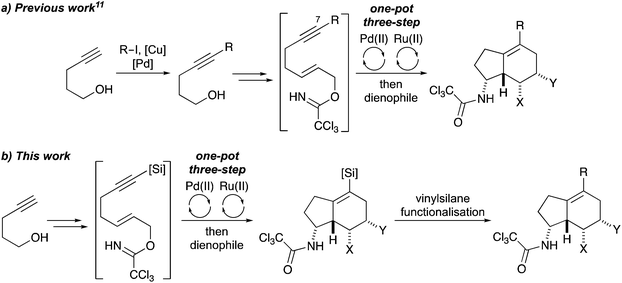 |
| Scheme 1 One-pot methods for the synthesis of aminobicyclo[4.3.0]nonanes. | |
We now report the use of alkynylsilyl derived allylic trichloroacetimidates as substrates for the one-pot multi-bond forming process and the diastereoselective synthesis of aminobicyclo[4.3.0]nonanes bearing a vinylsilane functional handle (Scheme 1b). We also demonstrate the synthetic utility of a benzyldimethylsilyl (BDMS) analogue for the late-stage synthesis of a small library of novel, drug-like aminobicyclo[4.3.0]nonanes with up to six stereogenic centres.
Results and discussion
The first aim of this project was the synthesis of a hept-2-en-6-yn-1-ol bearing a C-7 silyl group. Although a wide-range of silanes have been developed for cross-coupling reactions,13 the BDMS group14 was chosen as this was likely to be stable to the various steps required for allylic alcohol synthesis and the conditions of the one-pot process. While the vinyl-BDMS functionality is relatively robust, it has been used in a number of Hiyama–Denmark type couplings.15 To probe the steric requirements of the one-pot three-step process, the tert-butyldimethylsilyl (TBDMS) analogue was also prepared. Initially, pent-4-yn-1-ol bearing a C-5 TBDMS group was prepared in a one-pot operation by silylation of both the alkyne and alcohol moieties, followed by acid-mediated hydrolysis of the silyloxy group (Scheme 2).16 While this allowed rapid access to 5-(tert-butyldimethylsilyl)pent-4-yn-1-ol (8), the overall yield was only 31%. Therefore, preparation of the corresponding BDMS analogue 11 was performed in a stepwise fashion, with protection of the hydroxyl group as a THP ether before silylation of the alkyne with benzyldimethylsilyl chloride under basic conditions.17 Removal of the THP protecting group then gave 5-(benzyldimethylsilyl)pent-4-yn-1-ol (11) in quantitative yield over the three steps. The pent-4-yn-1-ols 8 and 11 were converted to the (E)-α,β-unsaturated ethyl esters 12 and 13 in high yields using a one-pot Swern oxidation and Horner–Wadsworth–Emmons reaction under mild Masamune–Roush condtions.18,19 Reduction of the ester groups using DIBAL-H then gave allylic alcohols 14 and 15 in excellent yields. The highly efficient six-step route (90% overall yield) for the preparation of BDMS-derived allylic alcohol 15 was easily amenable to scale-up, allowing synthesis of multi-gram quantities of this key substrate.
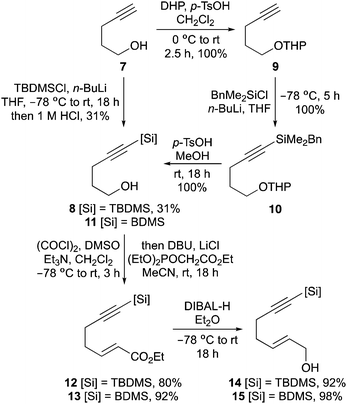 |
| Scheme 2 Synthesis of alkynyl derived allylic alcohols 14 and 15. | |
Having prepared the alkynylsilyl derived allylic alcohols, conditions for an optimal one-pot synthesis of the corresponding aminobicyclo[4.3.0]nonanes were next explored. The TBDMS-analogue that was designed to probe the steric limitation of the one-pot process was initially investigated (Scheme 3). The allylic trichloroacetimidate was formed by reaction of 14 with trichloroacetonitrile and DBU and without purification,20 this was subjected to the one-pot three-step process. In our previous study that evaluated C-7 substituted alkyne derived allylic alcohols for the one-pot process, it was found that while a relatively bulky substituent prevented coordination of the Pd(II)-catalyst to the alkyne and facilitated an efficient Overman rearrangement, the presence of this group hindered the following RCEYM step.11b With the TBDMS-derived allylic trichloroacetimidate, a similar outcome was observed for both steps. The Pd(II)-catalysed Overman rearrangement proceeded under standard conditions to give the allylic trichloroacetamide after 12 hours,21 however, the RCEYM reaction required forcing conditions. A combination of the use of 1,7-octadiene (an in situ source of ethylene) to accelerate the reaction,22 high loading of Grubbs 2nd generation catalyst (20 mol%) and a 120 hour reaction time was required for complete conversion to the enyne.23 Following the hydrogen bonding directed Diels–Alder reaction with N-phenyl maleimide, aminobicyclo[4.3.0]nonane 16 was isolated as a single diastereomer in 19% yield over the four steps. This relatively low yield is likely due to the extended RCEYM step and demonstrates the steric limitation of the one-pot process.
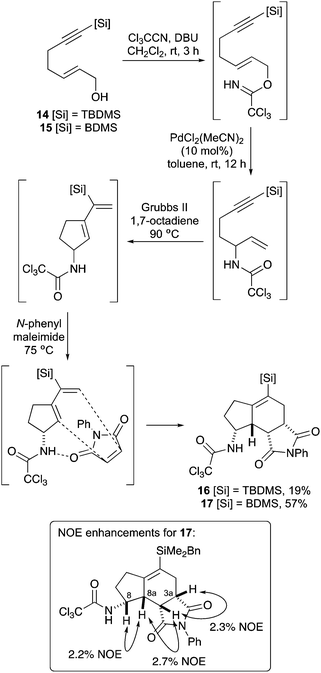 |
| Scheme 3 Synthesis of aminobicyclo[4.3.0]nonanes 16 and 17. | |
Application of the one-pot process using BDMS-allylic alcohol 15 was more straightforward (Scheme 3). Again, allylic trichloroacetimidate formation and Pd(II)-catalysed Overman rearrangement proceeded under standard conditions. The RCEYM reaction did require the presence of 1,7-octadiene, but needed only 7 mol% catalyst loading and was complete after 18 hours. Diels–Alder reaction with N-phenyl maleimide then gave aminobicyclo[4.3.0]nonane 17 as a single diastereomer in 57% yield over the four steps. As previously reported for the Diels–Alder reaction of trichloroacetamide derived cyclic exo-dienes, the reaction proceeds via a hydrogen bonding controlled endo transition state, forming the syn-products (syn relationship of hydrogen atoms at C-3a, C-8, C-8a and C-8b) with excellent diastereoselectivity (>20
:
1).10,11 Analysis of BDMS-derived aminobicyclo[4.3.0]nonane 17 using difference NOE experiments confirmed the relative stereochemistry and that the Diels–Alder reaction has proceeded in the same manner as other trichloroacetamide derived cyclic exo-diene substrates (Scheme 3).24
Having used the BDMS-group to perform a mild Pd(II)-catalysed Overman rearrangement and efficiently access the aminobicyclo[4.3.0]nonane core, we next wanted to demonstrate that the resulting vinylsilane could be used for the late-stage synthesis of a wide range of derivatives. Initially, removal of the trialkylsilyl group to access the parent scaffold was investigated (Scheme 4). During introduction of the BDMS-group for cross-coupling reactions, Trost and co-workers showed that BDMS-vinylsilanes were stable to proto-desilylation under typical fluoride conditions.14 This was confirmed on treatment of vinylsilane 17 with TBAF, which showed no reaction. Increasing the temperature or duration of the reaction only led to decomposition. Cleavage of the C–Si bond was achieved under acidic conditions. While the use of dilute hydrochloric acid solutions (2 M or 4 M) gave only partial proto-desilylation, treatment of 17 with 6 M hydrochloric acid at 60 °C gave 18 cleanly, in 74% yield. The reactivity of tri-substituted alkene 18 to oxidation was next studied. Osmium tetroxide mediated dihydroxylation under Donohoe conditions gave the desired diol product 19 as a single diastereomer in 86% yield.25 In a similar fashion, treatment of 18 with m-CPBA proceeded with high selectivity and the major diastereomer 20 was isolated in 73% yield.26,27 The high selectivity for both reactions is a consequence of the relative stereochemistry at the C-3a, C-8, C-8a and C-8b positions of the tricyclic core of 18. This creates a curved shape to the molecule where reactions readily take place at the more exposed convex face.
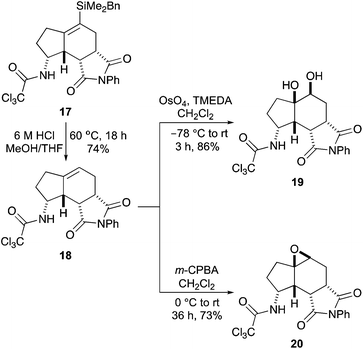 |
| Scheme 4 Synthesis and oxidation of aminobicyclo[4.3.0]nonane 18. | |
The final stage of this project then investigated cross-coupling reactions of vinylsilane 17 for the late-stage diversification of the aminobicyclo[4.3.0]nonane core. Using standard conditions for Hiyama–Denmark reactions with the BDMS-group,14 attempts were made to couple 17 with various electron-rich and electron-deficient aryl iodides. However, these reactions showed only decomposition of vinylsilane 17. Due to the inability of 17 to undergo cross-coupling reactions, an alternative strategy was sought using a more reactive vinyl functionality. Iodo-desilylation of vinylsilane 17 was found to proceed readily with iodine monochloride and gave vinyl iodide 21 in 88% yield (Scheme 5).28 This was then used to explore various palladium-catalysed cross-coupling reactions.
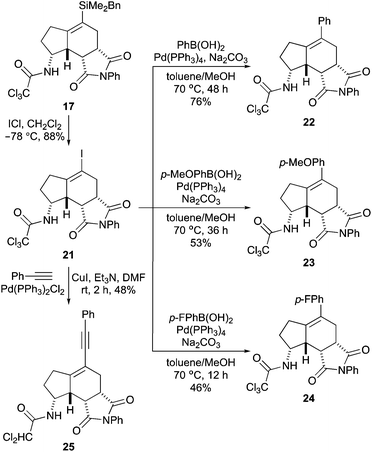 |
| Scheme 5 Synthesis and cross-coupling reactions of vinyl iodide 21. | |
After some optimisation, vinyl iodide 21 was found to be an efficient cross-coupling partner for Suzuki–Miyaura reactions. Using Pd(PPh3)4 (10 mol%) and phenylboronic acid under typical conditions gave the cross-coupled product 22 in 76% yield. Reaction of an electron-rich variant, p-methoxyphenylboronic acid also proceed smoothly, giving 23 in 53% yield. In previous studies, we have found that the trichloroacetamide group is prone to dechlorination during Pd(0)-catalysed reactions.11b,29 Similarly, in the Suzuki–Miyaura reactions to form 22 and 23, small amounts of dichloroacetamide analogues of these compounds were observed in the reaction mixture (<10%). This became a more significant issue in attempting a cross-coupling reaction with electron-defficient p-fluorophenylboronic acid, where substantial amounts of the reduced coupled product were also detected (∼20%). However, this by-product could be minimised (<10%) by using a shorter reaction time, which allowed the synthesis of 24 in 46% yield. To expand the diversity of aminobicyclo[4.3.0]nonanes at the C-5 position, a Sonogashira reaction with phenylacetylene was also performed. For this example, both palladium-mediated coupling and reduction of the trichloroacetamide were found to be rapid, leading to isolation of the dichloroacetamide-derived enyne 25 as the major product, in 48% yield.
Conclusions
In conclusion, two alkynylsilyl derived allylic trichloroacetimidates have been prepared and evaluated as substrates for a one-pot multi-reaction process for the preparation of novel aminobicyclo[4.3.0]nonanes. While a TBDMS-derivative showed the steric limitations of this process, a BDMS-analogue was efficiently converted to the corresponding aminobicyclo[4.3.0]nonane in good overall yield. The BDMS-compound was then explored for the late-stage diversification of the aminobicyclo[4.3.0]nonane core. Vinylsilane 17 was found to undergo both proto-desilylation and iodo-silylation reactions, leading to derivatives that could undergo a range of oxidation and cross-coupling reactions. Overall, this study has developed a general route to novel, sp3-rich aminobicyclo[4.3.0]nonanes incorporating diversity at a late-stage of the synthesis. To exemplify the strategy, the project focused on using a single dienophile, N-phenyl maleimide during the Diels–Alder reaction. However, as shown in previous studies,11 we believe that other alkenes will react readily with the BDMS-derived diene allowing further late-stage expansion of the compounds that can be formed via this process. Work is currently underway to achieve this goal and explore further reactions of BDMS-derived aminobicyclo[4.3.0]nonanes.
Experimental
All reagents and starting materials were obtained from commercial sources and used as received. All dry solvents were purified using a PureSolv 500 MD solvent purification system. All reactions were performed under an atmosphere of argon unless otherwise mentioned. Brine refers to a saturated solution of sodium chloride. Flash column chromatography was performed using Fisher matrix silica 60. Macherey-Nagel aluminium-backed plates pre-coated with silica gel 60 (UV254) were used for thin layer chromatography and were visualised by staining with KMnO4. 1H and 13C NMR spectra were recorded on a Bruker DPX 400 (1H: 400 MHz; 13C: 101 MHz) spectrometer or a Bruker 500 (1H: 500 MHz; 13C: 126 MHz) spectrometer with chemical shift values reported in ppm relative to a residual solvent peak and in the solvent stated. Assignment of 1H NMR signals is based on COSY experiments. Assignment of 13C NMR signals is based on HSQC and/or DEPT experiments. All coupling constants, J, are quoted in Hz. Mass spectra were obtained using a JEOL JMS-700 spectrometer for EI and CI or a Bruker Microtof-q for ESI. Infrared spectra were obtained neat using a Shimadzu IRPrestige-21 spectrometer. Melting points were determined on a Reichert platform melting point apparatus.
5-(tert-Butyldimethylsilyl)pent-4-yn-1-ol (8)30
n-Butyllithium (0.520 mL, 1.31 mmol, 2.5 M in hexane) was added dropwise to a solution of 4-pentyn-1-ol (7) (0.050 g, 0.590 mmol) in tetrahydrofuran (20 mL) at −78 °C. The reaction mixture was stirred for 0.75 h at −78 °C. tert-Butyldimethylsilyl chloride (0.220 g, 1.48 mmol) was then added. The reaction mixture was allowed to warm to room temperature and stirred for 18 h. A 1 M aqueous solution of hydrochloric acid (2 mL) was then added and the mixture was stirred for 2 h. The resulting mixture was diluted with ethyl acetate (10 mL), washed with water (2 × 15 mL) and a saturated solution of sodium bicarbonate (15 mL). The aqueous layers were washed with ethyl acetate (2 × 15 mL), and the combined organic phase was washed with brine (20 mL), dried (MgSO4), filtered and concentrated in vacuo. Purification by flash column chromatography (petroleum ether/ethyl acetate, 9
:
1) gave 5-(tert-butyldimethylsilyl)pent-4-yn-1-ol (8) (0.036 g, 31%) as a colourless oil. Spectroscopic data were consistent with the literature.30δH (400 MHz, CDCl3) 0.06 (6H, s, Si(CH3)2), 0.90 (9H, s, SiC(CH3)3), 1.75 (2H, quin., J 6.5 Hz, 2-H2), 1.99 (1H, br s, OH), 2.34 (2H, t, J 6.5 Hz, 3-H2), 3.74 (2H, t, J 6.5 Hz, 1-H2); δC (101 MHz, CDCl3) −4.5 (2 × CH3), 16.5 (C), 16.5 (CH2), 26.1 (3 × CH3), 31.3 (CH2), 61.8 (CH2), 83.3 (C), 107.2 (C); m/z (ESI) 221 (MNa+. 100%).
1-(Tetrahydropyran-2′-yloxy)-4-pentyne (9)31
To a solution of 4-pentyn-1-ol (7) (0.900 g, 10.8 mmol) and a catalytic amount of p-toluenesulfonic acid monohydrate (0.020 g) in dichloromethane (25 mL) at 0 °C was added 3,4-dihydro-2H-pyran (2.71 g, 32.2 mmol). The reaction mixture was allowed to warm to room temperature and stirred for 2.5 h. Ethyl acetate (50 mL) was then added and the solution was poured into a solution of sodium hydrogen carbonate (100 mL). The mixture was then extracted with ethyl acetate (3 × 50 mL). The organic layers were combined, washed with brine (100 mL), dried (MgSO4), filtered and concentrated in vacuo. Purification by flash column chromatography (petroleum ether/diethyl ether, 10
:
1) gave 1-(tetrahydropyran-2′-yloxy)-4-pentyne (9) (1.81 g, 100%) as a colourless oil. Spectroscopic data were consistent with the literature.31δH (400 MHz, CDCl3) 1.46–1.86 (8H, m, 2-H2, 3′-H2, 4′-H2 and 5′-H2), 1.94 (1H, t, J 2.7 Hz, 5-H), 2.28–2.35 (2H, m, 3-H2), 3.45–3.55 (2H, m, 1-HH and 6′-HH), 3.80–3.91 (2H, m, 1-HH and 6′-HH), 4.60 (1H, t, J 3.3 Hz, 2′-H); δC (101 MHz, CDCl3) 15.1 (CH2), 19.2 (CH2), 25.4 (CH2), 28.6 (CH2), 30.4 (CH2), 61.7 (CH2), 65.4 (CH2), 68.5 (CH), 83.6 (C), 98.4 (CH); m/z (EI) 168 (M+. 4%), 149 (8), 125 (11), 111 (12), 84 (74), 67 (28), 49 (100).
5-(Benzyldimethylsilyl)-1-(tetrahydropyran-2′-yloxy)pent-4-yne (10)32
n-Butyllithium (2.56 mL, 6.40 mmol, 2.5 M in hexane) was slowly added to a stirred solution of 1-(tetrahydropyran-2′-yloxy)-4-pentyne (9) (0.980 g, 5.82 mmol) in tetrahydrofuran (28 mL) at −78 °C. The resulting solution was stirred at −78 °C for 0.5 h at which time benzyldimethylsilyl chloride (1.16 mL, 6.40 mmol) was added. The resulting solution was stirred at −78 °C for 5 h. The reaction was quenched with a saturated solution of ammonium chloride (20 mL) and extracted with diethyl ether (3 × 20 mL). The combined organic layers were dried (MgSO4), filtered, and concentrated in vacuo. Purification by flash column chromatography (petroleum ether/diethyl ether, 8
:
2) gave 5-(benzyldimethylsilyl)-1-(tetrahydropyran-2′-yloxy)-4-pentyne (10) (1.84 g, 100%) as a colourless oil. Spectroscopic data were consistent with the literature.32δH (400 MHz, CDCl3) 0.10 (6H, s, Si(CH3)2), 1.47–1.87 (8H, m, 2-H2, 3′-H2, 4′-H2 and 5′-H2), 2.18 (2H, s, SiCH2), 2.35 (2H, t, J 7.1 Hz, 3-H2), 3.42–3.54 (2H, m, 1-HH and 6′-HH), 3.79–3.91 (2H, m, 1-HH and 6′-HH), 4.60 (1H, t, J 3.3 Hz, 2′-H), 7.05–7.12 (3H, m, 3 × ArH), 7.19–7.25 (2H, m, 2 × ArH); δC (101 MHz, CDCl3) 0.0 (2 × CH3), 18.7 (CH2), 21.4 (CH2), 27.4 (CH2), 28.4 (CH2), 30.7 (CH2), 32.6 (CH2), 64.0 (CH2), 67.7 (CH2), 85.0 (C), 100.6 (CH), 110.2 (C), 126.2 (CH), 130.0 (2 × CH), 130.3 (2 × CH), 141.1 (C); m/z (ESI) 339 (MNa+. 100%).
5-(Benzyldimethylsilyl)pent-4-yn-1-ol (11)17
p-Toluenesulfonic acid monohydrate (0.008 g, 0.042 mmol) was added to a solution of 5-(benzyldimethylsilyl)-1-(tetrahydropyran-2′-yloxy)pent-4-yne (10) (0.085 g, 0.270 mmol) in methanol (3 mL). The resulting solution was stirred at room temperature for 18 h. The reaction was quenched by addition of brine (50 mL). The aqueous layer was extracted with ethyl acetate (3 × 50 mL). The combined organic layers were dried (MgSO4), filtered, and concentrated in vacuo. Purification by flash column chromatography (petroleum ether/diethyl ether, 1
:
1) gave 5-(benzyldimethylsilyl)pent-4-yn-1-ol (11) (0.065 g, 100%) as a colourless oil. Spectroscopic data were consistent with the literature.17δH (400 MHz, CDCl3) 0.12 (6H, s, Si(CH3)2), 1.76 (2H, quin., J 6.5 Hz, 2-H2), 1.86 (1H, s, OH), 2.19 (2H, s, SiCH2), 2.35 (2H, t, J 6.5 Hz, 3-H2), 3.72 (2H, t, J 6.5 Hz, 1-H2), 7.06–7.13 (3H, m, 3 × ArH), 7.21–7.27 (2H, m, 2 × ArH); δC (101 MHz, CDCl3) 0.0 (2 × CH3), 18.4 (CH2), 28.4 (CH2), 33.0 (CH2), 63.6 (CH2), 85.5 (C), 110.0 (C), 126.2 (CH), 130.0 (2 × CH), 130.3 (2 × CH), 141.1 (C); m/z (ESI) 255 (MNa+. 100%).
Ethyl (2E)-7-(tert-butyldimethylsilyl)hept-2-en-6-ynoate (12)
Dimethyl sulfoxide (0.176 mL, 2.48 mmol) was added to a stirred solution of oxalyl chloride (0.120 mL, 1.39 mmol) in dichloromethane (5 mL) at −78 °C. The mixture was stirred for 0.3 h before 5-(tert-butyldimethylsilyl)-pent-4-yn-1-ol (8) (0.196 g, 0.990 mmol) in dichloromethane (2 mL) was slowly added. The mixture was stirred for a further 0.3 h before triethylamine (0.690 mL, 4.95 mmol) was added. This reaction mixture was stirred for 0.5 h at −78 °C and then allowed to warm to room temperature and stirred for a further 3 h. A solution of lithium chloride (0.750 g, 1.78 mmol), triethyl phosphonoacetate (0.354 mL, 1.78 mmol) and 1,8-diazabicyclo[5,4,0]undec-7-ene (2.66 mL, 1.78 mmol) in acetonitrile (4 mL) was then prepared and stirred for 1 h. The Swern solution was concentrated in vacuo, then the Horner–Wadsworth–Emmons solution was added and the reaction mixture was stirred at room temperature overnight. The reaction was quenched with a saturated solution of ammonium chloride (2.5 mL) and concentrated to give an orange residue, which was then extracted with diethyl ether (4 × 5 mL). The organic layers were combined, dried (MgSO4), filtered and concentrated in vacuo. Purification by flash column chromatography (petroleum ether/ethyl acetate, 7
:
3) gave ethyl (2E)-7-(tert-butyldimethylsilyl)hept-2-en-6-ynoate (12) (0.211 g, 80%) as a colourless oil. νmax/cm−1 (neat) 2929 (CH), 2364, 1723 (C
O), 1472, 1250, 1040, 837; δH (400 MHz, CDCl3) 0.07 (6H, s, Si(CH3)2), 0.91 (9H, s, SiC(CH3)3), 1.28 (3H, t, J 7.1 Hz, OCH2CH3), 2.34–2.45 (4H, m, 4-H2 and 5-H2), 4.18 (2H, q, J 7.1 Hz, OCH2CH3), 5.88 (1H, d, J 15.7 Hz, 2-H), 6.97 (1H, dt, J 15.7, 6.6 Hz, 3-H); δC (101 MHz, CDCl3) −4.6 (2 × CH3), 14.2 (CH3), 16.4 (C), 18.8 (CH2), 26.0 (3 × CH3), 31.3 (CH2), 60.2 (CH2), 84.0 (C), 105.8 (C), 122.5 (CH), 146.5 (CH), 166.3 (C); m/z (ESI) 289.1590 (MNa+. C15H26NaO2Si requires 289.1594).
Ethyl (2E)-7-(benzyldimethylsilyl)hept-2-en-6-ynoate (13)
Ethyl (2E)-7-(benzyldimethylsilyl)hept-2-en-6-ynoate (13) was synthesised as described for ethyl (2E)-7-(tert-butyldimethylsilyl)hept-2-en-6-ynoate (12) using 5-(benzyldimethylsilyl)pent-4-yn-1-ol (11) (0.990 g, 4.28 mmol). Purification by flash column chromatography (petroleum ether/diethyl ether, 8
:
2) gave ethyl (2E)-7-(benzyldimethylsilyl)hept-2-en-6-ynoate (13) (1.19 g, 92%) as a colourless oil. νmax/cm−1 (neat) 2958 (CH), 1720 (C
O), 1656 (C
C), 1494, 1249, 1154, 1039, 833, 758; δH (400 MHz, CDCl3) 0.10 (6H, s, Si(CH3)2), 1.29 (3H, t, J 7.1 Hz, OCH2CH3), 2.18 (2H, s, SiCH2), 2.34–2.45 (4H, m, 4-H2 and 5-H2), 4.20 (2H, q, J 7.1 Hz, OCH2CH3), 5.87 (1H, dt, J 15.7, 1.4 Hz, 2-H), 6.97 (1H, dt, J 15.7, 6.6 Hz, 3-H), 7.03–7.12 (3H, m, 3 × ArH), 7.19–7.25 (2H, m, 2 × ArH); δC (101 MHz, CDCl3) 0.0 (2 × CH3), 16.3 (CH3), 20.8 (CH2), 28.3 (CH2), 33.1 (CH2), 62.2 (CH2), 86.3 (C), 108.8 (C), 124.5 (CH), 126.3 (CH), 130.1 (2 × CH), 130.3 (2 × CH), 141.0 (C), 148.4 (CH), 168.2 (C); m/z (ESI) 323.1422 (MNa+. C18H24NaO2Si requires 323.1438).
(2E)-7-(tert-Butyldimethylsilyl)hept-2-en-6-yn-1-ol (14)
Ethyl (2E)-7-(tert-butyldimethylsilyl)hept-2-en-6-ynoate (12) (0.165 g, 0.620 mmol) was dissolved in diethyl ether (4 mL) and cooled to −78 °C. DIBAL-H (1 M in hexane) (1.36 mL, 1.36 mmol) was added dropwise and the reaction mixture was stirred at −78 °C for 3 h, before warming to room temperature overnight. The solution was cooled to 0 °C and quenched by the addition of a saturated solution of Rochelle salt (2 mL) and warmed to room temperature with vigorous stirring for 1 h, producing a white precipitate that was filtered through a pad of Celite® and washed with diethyl ether (3 × 4 mL). The filtrate was then dried (MgSO4), filtered and concentrated in vacuo. Purification by flash column chromatography (petroleum ether/ethyl acetate, 8
:
2) gave (2E)-7-(tert-butyldimethylsilyl)hept-2-en-6-yn-1-ol (14) (0.128 g, 92%) as a colourless oil. νmax/cm−1 (neat) 3332 (OH), 2929 (CH), 2174, 1472, 1250, 1007, 968, 837, 825, 774; δH (400 MHz, CDCl3) 0.07 (6H, s, Si(CH3)2), 0.92 (9H, s, SiC(CH3)3), 1.32 (1H, br s, OH), 2.23–2.39 (4H, m, 4-H2 and 5-H2), 4.09 (2H, br t, J 4.6 Hz, 1-H2), 5.66–5.78 (2H, m, 2-H and 3-H); δC (101 MHz, CDCl3) −4.5 (2 × CH3), 16.5 (C), 19.9 (CH2), 26.0 (3 × CH3), 31.5 (CH2), 63.5 (CH2), 83.1 (C), 107.0 (C), 130.4 (CH), 130.8 (CH); m/z (ESI) 247.1479 (MNa+. C13H24NaOSi requires 247.1489).
(2E)-7-(Benzyldimethylsilyl)hept-2-en-6-yn-1-ol (15)
(2E)-7-(Benzyldimethylsilyl)hept-2-en-6-yn-1-ol (15) was synthesised as described for (2E)-7-(tert-butyldimethylsilyl)hept-2-en-6-yn-1-ol (14) using ethyl (2E)-7-(benzyldimethylsilyl)hept-2-en-6-ynoate (13) (1.35 g, 4.49 mmol). Purification by flash column chromatography (petroleum ether/ethyl acetate, 9
:
1) gave (2E)-7-(benzyldimethylsilyl)hept-2-en-6-yn-1-ol (15) (1.14 g, 98%) as a colourless oil. νmax/cm−1 (neat) 3367 (OH), 2922 (CH), 2175, 1494, 1249, 838, 762, 698; δH (500 MHz, CDCl3) 0.11 (6H, s, Si(CH3)2), 1.43 (1H, br s, OH), 2.18 (2H, s, SiCH2), 2.23–2.34 (4H, m, 4-H2 and 5-H2), 4.10 (2H, d, J 4.4 Hz, 1-H2), 5.65–5.76 (2H, m, 2-H and 3-H), 7.06–7.12 (3H, m, 3 × ArH), 7.20–7.25 (2H, m, 2 × ArH); δC (101 MHz, CDCl3) 0.0 (2 × CH3) 21.8 (CH2), 28.4 (CH2), 33.2 (CH2), 65.4 (CH2), 85.5 (C), 109.9 (C), 126.2 (CH), 130.0 (2 × CH), 130.3 (2 × CH), 132.3 (CH), 132.6 (CH), 141.1 (C); m/z (ESI) 281.1323 (MNa+. C16H22NaOSi requires 281.1332).
(3aS*,8R*,8aS*,8bR*)-5-(tert-Butyldimethylsilyl)-3a,4,6,7,8a,8b-hexahydro-2-phenyl-8-(2′,2′,2′-trichloromethylcarbonylamino)cyclopent[e]isoindole-1,3(2H,3aH)-dione (16)
(2E)-7-(tert-Butyldimethylsilyl)hept-2-en-6-yn-1-ol (14) (0.054 g, 0.240 mmol) was dissolved in dichloromethane (6 mL) and cooled to 0 °C. To the solution 1,8-diazabicyclo[5.4.0]undec-7-ene (0.007 mL, 0.048 mmol) and trichloroacetonitrile (0.036 mL, 0.360 mmol) were added. The reaction mixture was allowed to warm to room temperature and stirred for 3 h. The reaction mixture was filtered through a short pad of silica gel with diethyl ether (100 mL) and the filtrate concentrated in vacuo to give the allylic trichloroacetimidate, which was used without further purification. The allylic trichloroacetimidate was dissolved in toluene (6 mL) and bis(acetonitrile)palladium chloride (0.006 g, 0.024 mmol) was then added and the reaction mixture was stirred at room temperature for 12 h. Grubbs second generation catalyst (0.020 g, 0.024 mmol) was added with 1,7-octadiene (0.142 mL, 0.960 mmol) and the reaction mixture was stirred for 60 h at 90 °C. A second portion of Grubbs second generation catalyst (0.020 g, 0.024 mmol) was added with 1,7-octadiene (0.142 mL, 0.960 mmol) and the reaction mixture was stirred for further 60 h at 90 °C. N-Phenyl maleimide (0.062 g, 0.360 mmol) was added with hydroquinone (0.003 g, 0.030 mmol). The reaction mixture was stirred for 18 h at 75 °C. The reaction mixture was then cooled and the solvent was evaporated. Flash column chromatography (petroleum ether/ethyl acetate, 8
:
2) gave (3aS*,8R*,8aS*,8bR*)-5-(tert-butyldimethylsilyl)-3a,4,6,7,8a,8b-hexahydro-2-phenyl-8-(2′,2′,2′-trichloromethylcarbonylamino)cyclopent[e]isoindole-1,3(2H,3aH)-dione (16) (0.025 g, 19%) as a yellow oil. νmax/cm−1 (neat) 3319 (NH), 2954 (CH), 1699 (C
O), 1517 (C
C), 1388, 1199, 827, 755; δH (400 MHz, CDCl3) 0.08 (3H, s, SiCH3), 0.15 (3H, s, SiCH3), 0.86 (9H, s, SiC(CH3)3), 1.83 (1H, ddd, J 18.6, 12.4, 7.6 Hz, 7-HH), 2.12–2.21 (2H, m, 4-HH and 7-HH), 2.24–2.38 (1H, m, 6-HH), 2.65 (1H, dd, J 16.5, 7.6 Hz, 6-HH), 2.92–2.98 (1H, m, 8a-H), 3.01 (1H, dd, J 14.8, 1.3 Hz, 4-HH), 3.32 (1H, ddd, J 8.9, 7.2, 1.3 Hz, 3a-H), 3.40 (1H, dd, J 8.9, 6.3 Hz, 8b-H), 4.77–4.88 (1H, m, 8-H), 7.12–7.17 (2H, m, 2 × ArH), 7.37–7.50 (3H, m, 3 × ArH), 8.84 (1H, d, J 9.6 Hz, NH); δC (126 MHz, CDCl3) −5.1 (CH3), −4.2 (CH3), 18.3 (C), 26.8 (3 × CH3), 30.3 (CH2), 30.5 (CH2), 31.9 (CH2), 39.3 (CH), 40.6 (CH), 43.1 (CH), 52.3 (CH), 92.9 (C), 126.4 (2 × CH), 128.8 (C), 129.0 (CH), 129.3 (2 × CH), 131.5 (C), 156.1 (C), 162.3 (C), 178.1 (C), 179.8 (C); m/z (ESI) 563.1045 (MNa+. C25H3135Cl3N2NaO3Si requires 563.1062).
(3aS*,8R*,8aS*,8bR*)-5-(Benzyldimethylsilyl)-3a,4,6,7,8a,8b-hexahydro-2-phenyl-8-(2′,2′,2′-trichloromethylcarbonylamino)cyclopent[e]isoindole-1,3(2H,3aH)-dione (17)
(3aS*,8R*,8aS*,8bR*)-5-(Benzyldimethylsilyl)-3a,4,6,7,8a,8b-hexahydro-2-phenyl-8-(2′,2′,2′-trichloromethylcarbonylamino)cyclopent[e]isoindole-1,3(2H,3aH)-dione (17) was synthesised as described for (3aS*,8R*,8aS*,8bR*)-5-(tert-butyldimethylsilyl)-3a,4,6,7,8a,8b-hexahydro-2-phenyl-8-(2′,2′,2′-trichloromethylcarbonylamino)cyclopent[e]isoindole-1,3(2H,3aH)-dione (16) using (2E)-7-(benzyldimethylsilyl)hept-2-en-6-yn-1-ol (15) (0.500 g, 1.92 mmol), except that the reaction mixture was stirred with Grubbs second generation catalyst (0.114 g, 0.134 mmol) and 1,7-octadiene (1.13 mL, 7.66 mmol) for 18 h at 90 °C before N-phenyl maleimide (0.500 g, 2.87 mmol) was added with hydroquinone (0.026 g, 0.24 mmol). The reaction mixture was stirred for 12 h at 75 °C. The reaction mixture was cooled to room temperature and the solvent was evaporated. Purification by flash column chromatography (petroleum ether/diethyl ether, 9
:
1) gave (3aS*,8R*,8aS*,8bR*)-5-(benzyldimethylsilyl)-3a,4,6,7,8a,8b-hexahydro-2-phenyl-8-(2′,2′,2′-trichloromethylcarbonylamino)cyclopent[e]isoindole-1,3(2H,3aH)-dione (17) (0.631 g, 57%) as a yellow oil. νmax/cm−1 (neat) 3313 (NH), 3023 (CH), 2957 (CH), 1698 (C
O), 1518 (C
C), 1389, 1198, 830, 754; δH (500 MHz, CDCl3) 0.05 (3H, s, SiCH3), 0.09 (3H, s, SiCH3), 1.73–1.89 (1H, m, 7-HH), 2.06–2.27 (5H, m, SiCH2, 4-HH, 6-HH and 7-HH), 2.52 (1H, dd, J 16.5, 7.6 Hz, 6-HH), 2.90–2.96 (1H, m, 8a-H), 2.99 (1H, dd, J 14.7, 1.6 Hz, 4-HH), 3.34 (1H, ddd, J 8.9, 6.8, 1.6 Hz, 3a-H), 3.42 (1H, dd, J 8.9, 6.5 Hz, 8b-H), 4.75–4.88 (1H, m, 8-H), 6.91–6.96 (2H, m, 2 × ArH), 7.04–7.10 (1H, m, ArH), 7.14–7.21 (4H, m, 4 × ArH), 7.38–7.50 (3H, m, 3 × ArH), 8.91 (1H, d, J 9.5 Hz, NH); δC (126 MHz, CDCl3) −3.2 (CH3), −2.6 (CH3), 25.2 (CH2), 29.4 (CH2), 29.8 (CH2), 31.9 (CH2), 39.5 (CH), 40.7 (CH), 43.1 (CH), 52.3 (CH), 92.9 (C), 124.3 (CH), 126.3 (2 × CH), 128.2 (2 × CH), 128.3 (2 × CH), 129.0 (C), 129.1 (CH), 129.4 (2 × CH), 131.5 (C), 139.3 (C), 155.7 (C), 162.3 (C), 178.4 (C), 179.9 (C); m/z (ESI) 597.0898 (MNa+. C28H2935Cl3N2NaO3Si requires 597.0905).
(3aS*,8R*,8aS*,8bR*)-4,6,7,8,8a,8b-Hexahydro-2-phenyl-8-(2′,2′,2′-trichloromethylcarbonylamino)cyclopent[e]isoindole-1,3(2H,3aH)-dione (18)10
To a solution of (3aS*,8R*,8aS*,8bR*)-5-(benzyldimethylsilyl)-3a,4,6,7,8a,8b-hexahydro-2-phenyl-8-(2′,2′,2′-trichloromethylcarbonylamino)cyclopent[e]isoindole-1,3(2H,3aH)-dione (17) (0.038 g, 0.066 mmol) in tetrahydrofuran (1 mL) was added methanol (2 mL) and 6 M hydrochloric acid (3 mL). The reaction mixture was stirred at 60 °C for 18 h. A 20% solution of aqueous sodium carbonate (6 mL) was added and the mixture was extracted with ethyl acetate (3 × 10 mL). The combined organic layers were dried (MgSO4), filtered and concentrated in vacuo. Purification by flash column chromatography (petroleum ether/ethyl acetate, 7
:
3) gave (3aS*,8R*,8aS*,8bR*)-4,6,7,8,8a,8b-hexahydro-2-phenyl-8-(2′,2′,2′-trichloromethylcarbonylamino)cyclopent[e]isoindole-1,3(2H,3aH)-dione (18) (0.021 g, 74%) as a white solid. Mp 174–176 °C; νmax/cm−1 (neat) 3304 (NH), 2955 (CH), 2924 (CH), 1695 (C
O), 1516 (C
C), 1388, 1288, 1202, 1182, 822, 750; δH (500 MHz, CDCl3) 1.81 (1H, qd, J 12.5, 7.6 Hz, 7-HH), 2.10–2.18 (1H, m, 7-HH), 2.19–2.38 (2H, m, 6-HH and 4-HH), 2.47 (1H, dd, J 16.2, 7.6 Hz, 6-HH), 2.85 (1H, ddd, J 15.1, 7.2, 1.1 Hz, 4-HH), 2.89–2.96 (1H, m, 8a-H), 3.33 (1H, ddd, J 8.7, 7.2, 1.1 Hz, 3a-H), 3.43 (1H, dd, J 8.7, 6.4 Hz, 8b-H), 4.80–4.91 (1H, m, 8-H), 5.75–5.81 (1H, m, 5-H), 7.15–7.20 (2H, m, 2 × ArH), 7.39–7.51 (3H, m, 3 × ArH), 8.95 (1H, d, J 9.2 Hz, NH); δC (126 MHz, CDCl3) 26.1 (CH2), 28.6 (CH2), 31.7 (CH2), 39.4 (CH), 41.2 (CH), 41.5 (CH), 52.9 (CH), 92.9 (C), 117.1 (CH), 126.5 (2 × CH), 129.1 (CH), 129.3 (2 × CH), 131.5 (C), 145.8 (C), 162.3 (C), 178.5 (C), 179.6 (C); m/z (CI) 427.0373 (MH+. C19H1835Cl3N2O3 requires 427.0383), 393 (65%), 359 (100), 325 (65), 311 (20), 266 (25), 174 (25), 113 (25), 71 (73).
(3aS*,5S*,5aR*,8R*,8aR*,8bR*)-5,5a-Dihydroxy-2-phenyl-3a,4,5,5a,6,7,8a,8b-octahydro-8-(2′,2′,2′-trichloromethylcarbonylamino)cyclopent[e]isoindole-1,3(2H,3aH)-dione (19)
(3aS*,8R*,8aS*,8bR*)-4,6,7,8,8a,8b-Hexahydro-2-phenyl-8-(2′,2′,2′-trichloromethylcarbonylamino)cyclopent[e]isoindole-1,3(2H,3aH)-dione (18) (0.029 g, 0.068 mmol) was dissolved in dichloromethane (2 mL) at −78 °C. Tetramethylethylenediamine (0.011 mL, 0.075 mmol) was added and the reaction mixture stirred for 0.1 h, before the addition of osmium tetroxide (0.019 g, 0.075 mmol). The dark coloured solution was stirred for 1 h at −78 °C before warming to room temperature and then stirred for a further 2 h. The solvent was removed in vacuo and the dark coloured solid was dissolved in methanol (2 mL). 12 M Hydrochloric acid (0.5 mL) was added and the reaction stirred for a further 2 h. The solvent was removed in vacuo to afford a dark solid. Flash column chromatography (dichloromethane/methanol, 19
:
1) gave (3aS*,5S*,5aR*,8R*,8aR*,8bR*)-5,5a-dihydroxy-2-phenyl-3a,4,5,5a,6,7,8a,8b-octahydro-8-(2′,2′,2′-trichloromethylcarbonylamino)cyclopent[e]isoindole-1,3(2H,3aH)-dione (19) (0.027 g, 86%) as a white solid. Mp 168–170 °C; νmax/cm−1 (neat) 3471 (OH), 3329 (NH), 2946 (CH), 1698 (C
O), 1515 (C
C), 1386, 1175, 821, 754; δH (500 MHz, CDCl3) 1.61–1.76 (1H, m, 6-HH), 1.85–2.03 (2H, m, 6-HH and 7-HH), 2.09 (1H, dt, J 13.5, 3.7 Hz, 4-HH), 2.18 (1H, ddd, J 13.5, 10.8, 6.7 Hz, 4-HH), 2.41–2.55 (1H, m, 7-HH), 2.70–2.89 (2H, m, 8a-H and OH), 3.00 (1H, br s, OH), 3.22 (1H, dd, J 9.0, 7.1 Hz, 8b-H), 3.35 (1H, ddd, J 9.0, 6.7, 3.7 Hz, 3a-H), 3.62 (1H, d, J 10.8 Hz, 5-H), 5.04–5.16 (1H, m, 8-H), 7.21–7.25 (2H, m, 2 × ArH), 7.40–7.56 (3H, m, 3 × ArH), 8.56 (1H, d, J 9.5 Hz, NH); δC (126 MHz, CDCl3) 29.7 (CH2), 30.3 (CH2), 37.1 (CH2), 38.6 (CH), 39.3 (CH), 48.1 (CH), 51.9 (CH), 70.1 (CH), 81.9 (C), 92.8 (C), 126.3 (2 × CH), 129.2 (CH), 129.5 (2 × CH), 131.2 (C), 162.1 (C), 178.0 (C), 178.8 (C); m/z (ESI) 483.0242 (MNa+. C19H1935Cl3N2NaO5 requires 483.0252).
(3aS*,5S*,5aR*,8R*,8aR*,8bR*)-5,5a-Epoxy-3a,4,5,5a,6,7,8a,8b-octahydro-2-phenyl-8-(2′,2′,2′-trichloromethylcarbonylamino)cyclopent[e]isoindole-1,3(2H,3aH)-dione (20)
3-Chloroperbenzoic acid (0.052 g, 0.300 mmol) was added to a stirred solution of (3aS*,8R*,8aS*,8bR*)-4,6,7,8,8a,8b-hexahydro-2-phenyl-8-(2′,2′,2′-trichloromethylcarbonylamino)cyclopent[e]isoindole-1,3(2H,3aH)-dione (18) (0.032 g, 0.075 mmol) in dichloromethane (2 mL) at 0 °C. The reaction mixture was warmed from 0 °C to room temperature over 18 h. The mixture was then cooled to 0 °C before 3-chloroperbenzoic acid (0.052 g, 0.300 mmol) was added. The reaction mixture was stirred for a further 24 h at room temperature, quenched by the addition of a saturated solution of sodium sulfite (3 mL) and extracted with dichloromethane (2 × 3 mL). The combined organic layers were washed with a saturated solution of sodium hydrogen carbonate (3 × 6 mL), water (6 mL), brine (6 mL), then dried (MgSO4), filtered and concentrated in vacuo. Purification by flash column chromatography (petroleum ether/ethyl acetate, 7
:
3) gave (3aS*,5S*,5aR*,8R*,8aR*,8bR*)-5,5a-epoxy-3a,4,5,5a,6,7,8a,8b-octahydro-2-phenyl-8-(2′,2′,2′-trichloromethylcarbonylamino)cyclopent[e]isoindole-1,3(2H,3aH)-dione (20) (0.024 g, 73%) as a light brown solid. Mp 154–156 °C; νmax/cm−1 (neat) 3265 (NH), 2934 (CH), 1697 (C
O), 1520 (C
C), 1394, 1192, 824, 773; δH (500 MHz, CDCl3) 1.65–1.72 (1H, m, 6-HH), 1.94–2.11 (2H, m, 6-HH and 7-HH), 2.23 (1H, dd, J 15.5, 8.5 Hz, 4-HH), 2.26–2.34 (1H, m, 7-HH), 2.77 (1H, dd, J 15.5, 4.1 Hz, 4-HH), 2.96 (1H, dd, J 11.2, 5.0 Hz, 8a-H), 3.05–3.12 (1H, m, 3a-H), 3.17 (1H, dd, J 9.8, 5.0 Hz, 8b-H), 3.59 (1H, d, J 4.1 Hz, 5-H), 4.70–4.82 (1H, m, 8-H), 7.27–7.33 (2H, m, 2 × ArH), 7.38–7.53 (3H, m, 3 × ArH), 9.33 (1H, d, J 9.8 Hz, NH); δC (126 MHz, CDCl3) 25.6 (CH2), 29.1 (CH2), 32.8 (CH2), 38.4 (CH), 40.0 (CH), 40.5 (CH), 50.5 (CH), 56.5 (CH), 67.9 (C), 92.8 (C), 126.7 (2 × CH), 128.9 (CH), 129.3 (2 × CH), 132.3 (C), 162.5 (C), 178.9 (C), 180.3 (C); m/z (ESI) 465.0144 (MNa+. C19H1735Cl3N2NaO4 requires 465.0146).
(3aS*,8R*,8aS*,8bR*)-3a,4,6,7,8a,8b-Hexahydro-5-iodo-2-phenyl-8-(2′,2′,2′-trichloromethylcarbonylamino)cyclopent[e]isoindole-1,3(2H,3aH)-dione (21)
To a solution of (3aS*,8R*,8aS*,8bR*)-5-(benzyldimethylsilyl)-3a,4,6,7,8a,8b-hexahydro-2-phenyl-8-(2′,2′,2′-trichloromethylcarbonylamino)cyclopent[e]isoindole-1,3(2H,3aH)-dione (17) (0.470 g, 0.820 mmol) in dichloromethane (10 mL) at −78 °C was added iodine monochloride (0.057 mL, 1.14 mmol). The reaction mixture was stirred at −78 °C for 3 h, then the excess iodine monochloride was destroyed by adding a 10% solution of sodium thiosulfate (20 mL) and the mixture was extracted with dichloromethane (3 × 20 mL). The combined organic layers were dried (MgSO4), filtered and concentrated in vacuo. Purification by flash column chromatography (petroleum ether/ethyl acetate, 8
:
2) gave (3aS*,8R*,8aS*,8bR*)-3a,4,6,7,8a,8b-hexahydro-5-iodo-2-phenyl-8-(2′,2′,2′-trichloromethylcarbonylamino)cyclopent[e]isoindole-1,3(2H,3aH)-dione (21) (0.400 g, 88%) as a white solid. Mp 130–132 °C; νmax/cm−1 (neat) 3309 (NH), 2954 (CH), 1698 (C
O), 1517 (C
C), 1391, 1200, 823; δH (400 MHz, CDCl3) 1.86 (1H, qd, J 12.3, 8.2 Hz, 7-HH), 2.12–2.34 (2H, m, 6-HH and 7-HH), 2.46 (1H, dd, J 16.9, 8.2 Hz, 6-HH), 2.84–2.94 (1H, m, 4-HH), 3.02–3.08 (1H, m, 8a-H), 3.28 (1H, dd, J 15.7, 1.2 Hz, 4-HH), 3.37 (1H, ddd, J 8.6, 6.7, 1.2 Hz, 3a-H), 3.42 (1H, dd, J 8.6, 5.8 Hz, 8b-H), 4.86–4.99 (1H, m, 8-H), 7.16–7.21 (2H, m, 2 × ArH), 7.41–7.53 (3H, m, 3 × ArH), 8.84 (1H, d, J 9.6 Hz, NH); δC (101 MHz, CDCl3) 30.6 (CH2), 33.6 (CH2), 39.8 (CH2), 40.6 (CH), 41.1 (CH), 44.5 (CH), 53.9 (CH), 82.0 (C), 92.8 (C), 126.5 (2 × CH), 129.3 (CH), 129.4 (2 × CH), 131.4 (C), 151.0 (C), 162.1 (C), 177.0 (C), 178.9 (C); m/z (ESI) 574.9151 (MNa+. C19H1635Cl3IN2NaO3 requires 574.9163).
(3aS*,8R*,8aS*,8bR*)-2,5-Diphenyl-3a,4,6,7,8a,8b-hexahydro-8-(2′,2′,2′-trichloromethylcarbonylamino)cyclopent[e]isoindole-1,3(2H,3aH)-dione (22)11b
To a solution of (3aS*,8R*,8aS*,8bR*)-3a,4,6,7,8a,8b-hexahydro-5-iodo-2-phenyl-8-(2′,2′,2′-trichloromethylcarbonylamino)cyclopent[e]isoindole-1,3(2H,3aH)-dione (21) (0.019 g, 0.045 mmol) in a 5
:
1 mixture of toluene/methanol (3 mL) at room temperature was added phenylboronic acid (0.008 g, 0.070 mmol), tetrakis(triphenylphosphine)palladium(0) (0.005 g, 0.005 mmol) and sodium carbonate (0.025 g, 0.180 mmol). The reaction mixture was heated to 70 °C and stirred for 48 h. The mixture was allowed to cool to room temperature and then concentrated in vacuo. The residue was dissolved in ethyl acetate (5 mL), washed with water (5 mL), brine (5 mL), dried (MgSO4), and then concentrated in vacuo. Flash column chromatography (petroleum ether/diethyl ether, 7
:
3) gave (3aS*,8R*,8aS*,8bR*)-2,5-diphenyl-3a,4,6,7,8a,8b-hexahydro-8-(2′,2′,2′-trichloromethylcarbonylamino)cyclopent[e]isoindole-1,3(2H,3aH)-dione (22) (0.017 g, 76%) as a yellow solid. Mp 151–153 °C; νmax (neat) 3358 (NH), 2936 (CH), 1695 (C
O), 1517, 1498, 1387, 1154, 822 cm−1; δH (400 MHz, CDCl3) 1.75 (1H, dq, J 12.3, 10.2 Hz, 7-HH), 2.10–2.20 (1H, m, 7-HH), 2.53–2.66 (3H, m, 4-HH and 6-H2), 3.12 (1H, dd, J 9.1, 5.8 Hz, 8a-H), 3.30 (1H, dd, J 15.2, 1.4 Hz, 4-HH), 3.46–3.56 (2H, m, 3a-H and 8b-H), 4.88–5.01 (1H, m, 8-H), 7.06–7.10 (2H, m, 2 × ArH), 7.23–7.47 (8H, m, 8 × ArH), 8.96 (1H, d, J 9.6 Hz, NH); δC (126 MHz, CDCl3) 28.4 (CH2), 31.6 (CH2), 31.7 (CH2), 40.3 (CH), 41.7 (CH), 43.7 (CH), 52.9 (CH), 93.0 (C), 126.6 (2 × CH), 127.2 (CH), 127.5 (2 × CH), 128.5 (2 × CH), 129.2 (CH), 129.4 (2 × CH), 130.2 (C), 131.5 (C), 139.0 (C), 139.7 (C), 162.3 (C), 178.6 (C), 179.8 (C); m/z (ESI) 525 ([MNa]+. 100%), 481 (18), 454 (7), 413 (7), 345 (24), 323 (21), 297 (9), 236 (11), 218 (7), 196 (6); m/z (ESI) 525.0947 (MNa+. C25H2135Cl3N2NaO3 requires 525.0510).
(3aS*,8R*,8aS*,8bR*)-3a,4,6,7,8a,8b-Hexahydro-5-(4′′-methoxyphenyl)-2-phenyl-8-(2′,2′,2′-trichloromethylcarbonylamino)cyclopent[e]isoindole-1,3(2H,3aH)-dione (23)11b
The reaction was carried out according to the previously described procedure for (3aS*,8R*,8aS*,8bR*)-2,5-diphenyl-3a,4,6,7,8a,8b-hexahydro-8-(2′,2′,2′-trichloromethylcarbonylamino)cyclopent[e]isoindole-1,3(2H,3aH)-dione (22) using (3aS*,8R*,8aS*,8bR*)-3a,4,6,7,8a,8b-hexahydro-5-iodo-2-phenyl-8-(2′,2′,2′-trichloromethylcarbonylamino)cyclopent[e]isoindole-1,3(2H,3aH)-dione (21) (0.025 g, 0.045 mmol) and 4-methoxyphenylboronic acid (0.010 g, 0.070 mmol) and performed at 70 °C for 36 h. Flash column chromatography (petroleum ether/diethyl ether, 8
:
2) gave (3aS*,8R*,8aS*,8bR*)-3a,4,6,7,8a,8b-hexahydro-5-(4′′-methoxyphenyl)-2-phenyl-8-(2′,2′,2′-trichloromethylcarbonylamino)cyclopent[e]isoindole-1,3(2H,3aH)-dione (23) (0.013 g, 53%) as a yellow solid. Mp 115–117 °C; νmax/cm−1 (neat) 3308 (NH), 2959 (CH), 1698 (C
O), 1512 (C
C), 1391, 1247, 823; δH (400 MHz, CDCl3) 1.66–1.81 (1H, m, 7-HH), 2.09–2.19 (1H, m, 7-HH), 2.52–2.62 (3H, m, 4-HH and 6-H2), 3.10 (1H, dd, J 8.9, 6.3 Hz, 8a-H), 3.27 (1H, dd, J 15.2, 1.2 Hz, 4-HH), 3.44–3.54 (2H, m, 3a-H and 8b-H), 3.81 (3H, s, OCH3), 4.87–4.99 (1H, m, 8-H), 6.85–6.92 (2H, m, 3′′-H and 5′′-H), 7.04–7.09 (2H, m, 2 × ArH), 7.17–7.23 (2H, m, 2′′-H and 6′′-H), 7.34–7.46 (3H, m, 3 × ArH), 8.97 (1H, d, J 9.6 Hz, NH); δC (101 MHz, CDCl3) 28.3 (CH2), 31.6 (CH2), 31.7 (CH2), 40.3 (CH), 41.7 (CH), 43.7 (CH), 52.9 (CH), 55.3 (CH3), 92.9 (C), 113.8 (2 × CH), 126.5 (2 × CH), 128.7 (2 × CH), 129.1 (CH), 129.4 (2 × CH), 129.8 (C), 131.4 (C), 131.4 (C), 138.1 (C), 158.7 (C), 162.3 (C), 178.6 (C), 179.8 (C); m/z (ESI) 555.0599 (MNa+. C26H2335Cl3N2NaO4 requires 555.0616).
(3aS*,8R*,8aS*,8bR*)-5-(4′′-Fluorophenyl)-3a,4,6,7,8a,8b-hexahydro-2-phenyl-8-(2′,2′,2′-trichloromethylcarbonylamino)cyclopent[e]isoindole-1,3(2H,3aH)-dione (24)
The reaction was carried out according to the previously described procedure for (3aS*,8R*,8aS*,8bR*)-2,5-diphenyl-3a,4,6,7,8a,8b-hexahydro-8-(2′,2′,2′-trichloromethylcarbonylamino)cyclopent[e]isoindole-1,3(2H,3aH)-dione (22) using (3aS*,8R*,8aS*,8bR*)-3a,4,6,7,8a,8b-hexahydro-5-iodo-2-phenyl-8-(2′,2′,2′-trichloromethylcarbonylamino)cyclopent[e]isoindole-1,3(2H,3aH)-dione (21) (0.030 g, 0.054 mmol) and 4-fluorophenylboronic acid (0.019 g, 0.135 mmol) and performed at 70 °C for 12 h. Flash column chromatography (petroleum ether/ethyl acetate, 9
:
1) gave (3aS*,8R*,8aS*,8bR*)-5-(4′′-fluorophenyl)-3a,4,6,7,8a,8b-hexahydro-2-phenyl-8-(2′,2′,2′-trichloromethylcarbonylamino)cyclopent[e]isoindole-1,3(2H,3aH)-dione (24) (0.013 g, 46%) as a white solid. Mp 124–126 °C; νmax/cm−1 (neat) 3312 (NH), 2926 (CH), 1700 (C
O), 1510 (C
C), 1500, 1391, 838, 756; δH (500 MHz, CDCl3) 1.69–1.82 (1H, m, 7-HH), 2.12–2.20 (1H, m, 7-HH), 2.48–2.66 (3H, m, 4-HH and 6-H2), 3.12 (1H, dd, J 8.6, 6.6 Hz, 8a-H), 3.25 (1H, dd, J 15.2, 1.2 Hz, 4-HH), 3.45–3.56 (2H, m, 3a-H and 8b-H), 4.88–4.98 (1H, m, 8-H), 7.00–7.09 (4H, m, 3′′-H, 5′′-H and 2 × ArH), 7.19–7.25 (2H, m, 2′′-H and 6′′-H), 7.37–7.48 (3H, m, 3 × ArH), 8.95 (1H, d, J 9.6 Hz, NH); δC (126 MHz, CDCl3) 28.3 (CH2), 31.7 (CH2), 31.7 (CH2), 40.3 (CH), 41.6 (CH), 43.7 (CH), 52.8 (CH), 92.9 (C), 115.4 (2 × CH, 2JCF 21.4 Hz), 126.5 (2 × CH), 129.2 (2 × CH, 3JCF 8.0 Hz), 129.2 (CH), 129.4 (2 × CH), 129.4 (C), 131.4 (C), 134.9 (C, 4JCF 3.2 Hz), 139.6 (C), 161.8 (C, 1JCF 247.3 Hz), 162.3 (C), 178.5 (C), 179.7 (C); m/z (ESI) 543.0393 (MNa+. C25H20F35Cl3N2NaO3 requires 543.0416).
(3aS*,8R*,8aS*,8bR*)-3a,4,6,7,8a,8b-Hexahydro-5-(phenylethynyl)-2-phenyl-8-(2′,2′-dichloromethylcarbonylamino)cyclopent[e]isoindole-1,3(2H,3aH)-dione (25)
To a solution of (3aS*,8R*,8aS*,8bR*)-3a,4,6,7,8a,8b-hexahydro-5-iodo-2-phenyl-8-(2′,2′,2′-trichloromethylcarbonylamino)cyclopent[e]isoindole-1,3(2H,3aH)-dione (21) (0.016 g, 0.029 mmol) in N,N-dimethylformamide (0.5 mL) were added copper iodide (0.001 g, 0.006 mmol) and bis(triphenylphosphine)palladium dichloride (0.002 g, 0.003 mmol). Phenylacetylene (0.004 mL, 0.037 mmol) was dissolved in degassed triethylamine (2 mL) and added to the reaction mixture. The solution was briefly heated to 60 °C and then left to stir at room temperature for 2 h. The solution was concentrated in vacuo and purified by flash column chromatography (petroleum ether/ethyl acetate, 3
:
2) to give (3aS*,8R*,8aS*,8bR*)-3a,4,6,7,8a,8b-hexahydro-5-(phenylethynyl)-2-phenyl-8-(2′,2′-dichloromethylcarbonylamino)cyclopent[e]isoindole-1,3(2H,3aH)-dione (25) (0.007 g, 48%) as a colourless oil. νmax/cm−1 (neat) 3021 (CH), 2361, 1705 (C
O), 1522 (C
C), 1393, 1215, 754; δH (400 MHz, CDCl3) 1.78–1.92 (1H, m, 7-HH), 2.09–2.22 (1H, m, 7-HH), 2.35–2.57 (2H, m, 4-HH and 6-HH), 2.84 (1H, dd, J 17.6, 7.7 Hz, 6-HH), 2.99–3.02 (1H, m, 8a-H), 3.06 (1H, dd, J 15.0, 1.3 Hz, 4-HH), 3.39 (1H, ddd, J 8.8, 7.7, 1.3 Hz, 3a-H), 3.47 (1H, dd, J 8.8, 6.3 Hz, 8b-H), 4.94 (1H, dtd, J 12.3, 9.8, 7.1 Hz, 8-H), 5.97 (1H, s, CHCl2), 7.18–7.23 (2H, m, 2 × ArH), 7.29–7.34 (3H, m, 3 × ArH), 7.38–7.53 (5H, m, 5 × ArH), 8.58 (1H, d, J 9.8 Hz, NH); δC (101 MHz, CDCl3) 28.7 (CH2), 31.0 (CH2), 31.3 (CH2), 39.4 (CH), 41.1 (CH), 42.8 (CH), 51.7 (CH), 66.6 (CH), 86.7 (C), 93.5 (C), 111.8 (C), 123.0 (C), 126.5 (2 × CH), 128.3 (2 × CH), 128.4 (CH), 129.1 (CH), 129.3 (2 × CH), 131.5 (2 × CH), 131.5 (C), 151.4 (C), 164.5 (C), 177.7 (C), 179.0 (C); m/z (ESI) 515.0875 (MNa+. C27H2235Cl2N2NaO3 requires 515.0900).
Acknowledgements
Financial support from the Ministry of Higher Education and Scientific Research, Omar Al-Mukhtar University, Libya (studentship to M. A. B. M.) and the University of Glasgow is gratefully acknowledged.
Notes and references
-
S. V. Bhat, B. A. Nagasampagi and M. Sivakumar, in Chemistry of Natural Products, Springer, Berlin, Germany, 2005 Search PubMed.
-
(a) H. Sorek, A. Rudi, S. Gueta, F. Reyes, M. J. Martin, M. Aknin, E. Gaydou and J. Vacelet, Tetrahedron, 2006, 62, 8838 CrossRef CAS;
(b) E. Gros, A. Al-Mourabit, M.-T. Martin, J. Sorres, J. Vacelet, M. Frederich, M. Aknin, Y. Kashman and A. Gauvin-Bialecki, J. Nat. Prod., 2014, 77, 818 CrossRef CAS PubMed.
- A. E. Walts and W. R. Roush, Tetrahedron, 1985, 41, 3463 CrossRef CAS and references therein.
-
(a) S. Ito, T. Matsuya, S. Omura, M. Otani and A. Nakagawa, J. Antibiot., 1970, 23, 315 CrossRef CAS PubMed;
(b) S. Mithani, G. Weeratunga, N. J. Taylor and G. I. Dmitrienko, J. Am. Chem. Soc., 1994, 116, 2209 CrossRef CAS;
(c) K. C. Nicolaou, H. Li, A. L. Nold, D. Pappo and A. Lenzen, J. Am. Chem. Soc., 2007, 129, 10356 CrossRef CAS PubMed.
-
(a) Y. Inubushi, H. Ishii, B. Yasui, M. Hashimoto and T. Harayama, Chem. Pharm. Bull., 1968, 16, 92 CrossRef CAS;
(b) T. Harayama, M. Ohtani, M. Oki and Y. Inubushi, J. Chem. Soc., Chem. Commun., 1974, 827 RSC.
- For recent developments, see: H. Yu, I. J. Kim, J. E. Folk, X. Tian, R. B. Rothman, M. H. Baumann, C. M. Dersch, J. L. Flippen-Anderson, D. Parrish, A. E. Jacobsen and K. C. Rice, J. Med. Chem., 2004, 47, 2624 CrossRef CAS PubMed.
-
(a) A. Graul and J. Castaner, Drugs Future, 1998, 23, 903 Search PubMed;
(b) J. Sterling, A. Veinberg, D. Lerner, W. Goldenberg, R. Levy, M. Youdim and J. Finberg, J. Neural Transm. Suppl., 1998, 52, 301 CAS.
- For examples, see:
(a) F. Lhermitte and P. Knochel, Angew. Chem., Int. Ed., 1998, 37, 2459 CrossRef;
(b) S. H. Lee, S. J. Park, I. S. Kim and Y. H. Jung, Tetrahedron, 2013, 69, 1877 CrossRef CAS.
- For examples, see:
(a) K. M. Brummond, D. Chen, T. O. Painter, S. Mao and D. D. Seifried, Synlett, 2008, 759 CrossRef CAS;
(b) M. W. Grafton, L. J. Farrugia and A. Sutherland, J. Org. Chem., 2013, 78, 7199 CrossRef CAS PubMed;
(c) D. R. White, J. T. Hutt and J. P. Wolfe, J. Am. Chem. Soc., 2015, 137, 11246 CrossRef CAS PubMed.
- M. W. Grafton, L. J. Farrugia, H. M. Senn and A. Sutherland, Chem. Commun., 2012, 48, 7994 Search PubMed.
-
(a) M. W. Grafton, S. A. Johnston, L. J. Farrugia and A. Sutherland, Tetrahedron, 2014, 70, 7133 CrossRef CAS;
(b) M. A. B. Mostafa, M. W. Grafton, C. Wilson and A. Sutherland, Org. Biomol. Chem., 2016, 14, 3284 RSC.
- Mono-substituted alkyne derived allylic trichloroacetimidates are poor substrates for a palladium(II)-catalysed Overman rearrangement due to binding of the catalyst to the alkyne moiety. For these compounds, a high temperature thermal Overman rearrangement is required for preparation of the allylic trichloroacetamide.
-
(a) S. E. Denmark, J. Org. Chem., 2009, 74, 2915 CrossRef CAS PubMed;
(b) Y. Nakao and T. Hiyama, Chem. Soc. Rev., 2011, 40, 4893 RSC.
- B. M. Trost, M. R. Mackacek and Z. T. Ball, Org. Lett., 2003, 5, 1895 CrossRef CAS PubMed.
- For example, see:
(a) C. S. Junker, M. E. Welker and C. S. Day, J. Org. Chem., 2010, 75, 8155 CrossRef CAS PubMed;
(b) J. Bergueiro, J. Montenegro, F. Cambeiro, C. Saá and S. López, Chem. – Eur. J., 2012, 18, 4401 CrossRef CAS PubMed;
(c) C. S. Junker and M. E. Welker, Tetrahedron, 2012, 68, 5341 CrossRef CAS.
- B. C. Bishop, I. F. Cottrell and D. Hands, Synthesis, 1997, 1315 CrossRef CAS.
- D. Gallenkamp and A. Fürstner, J. Am. Chem. Soc., 2011, 133, 9232 CrossRef CAS PubMed.
- R. E. Ireland and D. W. Norbeck, J. Org. Chem., 1985, 50, 2198 CrossRef CAS.
- M. A. Blanchette, W. Choy, J. T. Davis, A. P. Essenfeld, S. Masamune, W. R. Roush and T. Sakai, Tetrahedron Lett., 1984, 25, 2183 CrossRef CAS.
- C. E. Anderson, L. E. Overman and M. P. Watson, Org. Synth., 2005, 82, 134 CrossRef CAS.
-
L. E. Overman and N. E. Carpenter, in Organic Reactions, ed. L. E. Overman, Wiley, Hoboken, NJ, 2005, vol. 66, pp. 1–107 and references therein Search PubMed.
- S. Fustero, P. Bello, J. Miró, A. Simón and C. del Pozo, Chem. – Eur. J., 2012, 18, 10991 Search PubMed.
-
(a) M. Scholl, S. Ding, C. W. Lee and R. H. Grubbs, Org. Lett., 1999, 1, 953 CrossRef CAS PubMed;
(b) M. S. Sanford, J. A. Love and R. H. Grubbs, J. Am. Chem. Soc., 2001, 123, 6543 CrossRef CAS PubMed.
- Similar NOE enhancements were observed for aminobicyclo[4.3.0]nonane 16. See ESI† for full details.
-
(a) T. J. Donohoe, K. Blades, M. Helliwell, P. R. Moore and J. J. G. Winter, J. Org. Chem., 1999, 64, 2980 CrossRef CAS PubMed;
(b) K. Blades, T. J. Donohoe, J. J. G. Winter and G. Stemp, Tetrahedron Lett., 2000, 41, 4701 CrossRef CAS;
(c) T. J. Donohoe, K. Blades, P. R. Moore, M. J. Waring, J. J. G. Winter, M. Helliwell, N. J. Newcombe and G. Stemp, J. Org. Chem., 2002, 67, 7946 CrossRef CAS PubMed;
(d) T. J. Donohoe, Synlett, 2002, 1223 CrossRef CAS.
- P. O'Brien, A. C. Childs, G. J. Ensor, C. L. Hill, J. P. Kirby, M. J. Dearden, S. J. Oxenford and C. M. Rosser, Org. Lett., 2003, 5, 4955 CrossRef PubMed.
- The relative stereochemistry of diol 19 and epoxide 20 was confirmed by difference NOE experiments. See ESI† for full details.
- For examples of iodo-desilylation with iodine monochloride, see:
(a) M. A. Campo and R. C. Larock, Org. Lett., 2000, 2, 3675 CrossRef CAS PubMed;
(b) K.-I. Kanno, Y. Liu, A. Iesato, K. Nakajima and T. Takahashi, Org. Lett., 2005, 7, 5453 CrossRef CAS PubMed.
- E. D. D. Calder, S. A. I. Sharif, F. I. McGonagle and A. Sutherland, J. Org. Chem., 2015, 80, 4683 CrossRef CAS PubMed.
- T. M. Bertolini, Q. H. Nguyen and D. F. Harvey, J. Org. Chem., 2002, 67, 8675 CrossRef CAS PubMed.
- K. Uwai, Y. Oshima, T. Sugihara and T. Ohta, Tetrahedron, 1999, 55, 9469 CrossRef CAS.
- K. Miura, S. Okajima, T. Hondo, T. Nakagawa, T. Takahashi and A. Hosomi, J. Am. Chem. Soc., 2000, 122, 11348 CrossRef CAS.
Footnote |
† Electronic supplementary information (ESI) available: NOE data for compounds 16–25 and, 1H and 13C NMR spectra of all compounds. See DOI: 10.1039/c7ob00456g |
|
This journal is © The Royal Society of Chemistry 2017 |