DOI:
10.1039/C6OB02745H
(Communication)
Org. Biomol. Chem., 2017,
15, 1321-1324
Reversal of the sense of enantioselectivity between 1- and 2-aza[6]helicenes used as chiral inducers of asymmetric autocatalysis†
Received
15th December 2016
, Accepted 9th January 2017
First published on 9th January 2017
Abstract
Reversal of the sense of enantioselectivity was observed between 1-aza[6]helicene 2 and 2-aza[6]helicene 3 employed as chiral inducers of asymmetric autocatalysis of pyrimidyl alkanol. In the presence of (P)-(+)-1-aza[6]helicene 2, the reaction of pyrimidine-5-carbaldehyde 1 with diisopropylzinc afforded, in conjunction with asymmetric autocatalysis, (S)-pyrimidyl alkanol 4 with high ee. Surprisingly, the reaction in the presence of (P)-(+)-2-aza[6]helicene 3 gave the opposite enantiomer of (R)-alkanol 4 with high ee. In the same manner, (M)-(−)-2 and (M)-(−)-3 afforded (R)- and (S)-alkanol 4, respectively. The sense of enantioselectivity is controlled not only by the helicity of the azahelicene derivatives but also by the position of the nitrogen atom.
Enormous progress has been achieved in the absolute stereochemistry control of enantioselective catalytic organic reactions within the last few decades. Although various types of chirality inducers have systematically been explored, small helical organocatalysts or metal complexes bearing helical ligands have rarely been used in enantioselective catalysis until recently.1 Stimulated by a successful development of diverse synthetic methodologies for the preparation of functionalized (hetero)helicenes in a racemic or enantiopure form,2,3 their application to enantioselective catalysis has nowadays been attracting considerable attention and this field of chiroscience is rapidly expanding. Within the last few years, new helicene-derived chiral inducers have been prepared and their high efficiency in chirality transfer (or reactivity) in diverse fields of catalysis was demonstrated by Cauteruccio et al.,4 Cauteruccio, Benaglia et al.,5 Licandro, Hashmi et al.,6 Marinetti, Voituriez et al.,7 Suemune, Usui et al.,8 Tsujihara, Kawano et al.9 and Stará, Starý et al.10 However, to the best of our knowledge, only a few studies on azahelicenes (or their simple derivatives) in enantioselective catalysis have so far been carried out by Carbery et al.,11 Stará, Starý et al.12 and Takenaka et al.13
In the realm of enantioselective catalysis, the Soai reaction14–16 possesses a prominent position as it exhibits a unique chirality amplification through the asymmetric autocatalysis. Chirality inducers of a fascinating diversity (ranging from circularly polarized light17 to isotopically chiral compounds18 and chiral inorganic materials19) were found to control effectively the absolute configuration of enantiopure or highly enantioenriched 5-pyrimidyl alkanol as the product of diisopropylzinc addition to the corresponding pyrimidine-5-carbaldehyde. Intriguingly, the influence of helically chiral (hetero)helicenes on the stereochemical outcome of this reaction has already been examined to find a high level of chirality transfer (Scheme 1).20 Regardless of the absence/presence of heteroatoms and the length of the backbone, the use of the (P)-(+) enantiomers of [5]helicene 5, [6]helicene 6 or tetrathia[7]helicenes 7 in catalytic amounts resulted uniformly in the formation of (S)-(−)-4. Complementarily, the respective (M)-(−) helicene enantiomers induced the opposite chirality of (R)-(+)-4. Although different mechanisms of the chirality transfer were proposed (a noncovalent interaction between the helical hydrocarbons 5 or 6 and pyrimidine-5-carbaldehyde 1 to form a chiral aldehyde complex20a or, alternatively, coordination of diisopropylzinc to sulfur atoms of thiahelicenes 7 to generate a chiral active zinc species20b), a pivotal role of the helicity of the chirality inducer in the enantioselective reaction was experimentally demonstrated. Interestingly, the enantioselective addition of diethylzinc to benzaldehyde and its derivatives under catalysis by (P,P)-(+)-bis[5]helicenediol was pioneered by Katz et al.21 They observed the good ee's and predominant formation of (S)-(−)-1-phenylpropanol (and its o-chloro, p-chloro and p-methoxy derivatives of the same absolute configuration) in agreement with the aforementioned results by Soai et al. Thus, as so far found, the use of (P)-helicene as the chirality inducer in the asymmetric addition of dialkylzinc to (hetero)aromatic aldehydes leads generally to the (S)-configuration of the corresponding (hetero)aryl alkanol while (M)-helicene favors the formation of (R)-(hetero)aryl alkanol.
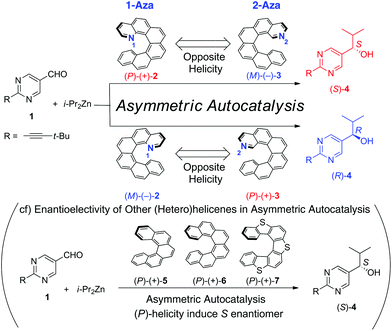 |
| Scheme 1 Reversal of enantioselectivity by the positions of the nitrogen atoms of 1- and 2-aza[6]helicenes. | |
Herein, we report on the use of the enantioenriched (P)-(+)/(M)-(−)-1-aza[6]helicene 2
22 and 2-aza[6]helicene 3
22 (Scheme 1) as chirality inducers in the enantioselective addition of diisopropylzinc (i-Pr2Zn) to pyrimidine-5-carbaldehyde 1 to demonstrate that the absolute configuration of the resulting pyrimidyl alkanol 4 is controlled not only by the helicity of the azahelicene derivatives but also the position of the nitrogen atom in them.
Both 1-aza[6]helicene 2 and 2-aza[6]helicene 3 were synthesized in the racemic form by employing [2 + 2 + 2] cycloisomerization of triynes to form their helical backbone.22 Optically pure (M) and (P) enantiomers were obtained by resolution of the corresponding racemates by co-crystallization with (+)-O,O′-dibenzoyl-d-tartaric acid or HPLC on a Chiralcel OD-H column (heptane/2-propanol 3
:
1). The helicity of the resolved enantiomers was unequivocally assigned by comparing their ECD spectra with that of (P)-(+)-[6]helicene23 whose helicity is known.24 Furthermore, the absolute helicities of both 1- and 2-aza[6]helicene were confirmed by single crystal X-ray diffraction analysis of enantiopure crystals with a Cu Kα X-ray source.‡
The results of asymmetric autocatalysis initiated by enantioenriched 1-aza[6]helicenes 2 or 2-aza[6]helicenes 3 are summarized in Table 1.§ When pyrimidine-5-carbaldehyde 1 was reacted with i-Pr2Zn using (P)-(+)-1-aza[6]helicene 2 with >99% ee as the chiral inducer, (S)-(−)-pyrimidyl alkanol 4 with 99% ee was obtained (Table 1, entry 1). On the other hand, the presence of (M)-(−)-2 with 99% ee gave the opposite enantiomer of (R)-(+)-alkanol 4 with 97% ee (entry 2). The ee of the products was amplified over >99.5% by additional rounds of asymmetric autocatalysis cycles.14b The correlation between the absolute configurations of 1-aza[6]helicene 2 and the alkanol 4 is reproducible (entries 3 and 4). Even when the chiral initiators of (P)-(+)- and (M)-(−)-2 of a low enantioenrichment (33% ee and 30% ee, respectively) were employed, the same correlation of absolute configurations was manifested by the formation of (S)-(−)- and (R)-(+)-alkanol 4 with 99% and 96% ee, respectively. This selectivity of the chiral 1-aza[6]helicene 2 as a chiral inducer of asymmetric autocatalysis, i.e., (P)-(+)-2 affords (S)-(−)-alkanol 4, is constant with the stereocontrol by (P)-(+)-[5]helicene 5, (P)-(+)-[6]helicene 6 and (P)-(+)-thia[7]helicene 7 affording also (S)-(−)-alkanol 4.
Table 1 Asymmetric autocatalysis initiated by enantioenriched 1-aza[6]helicene 2 and 2-aza[6]helicene 3
Entry |
1-Aza[6]helicene 2a or 2-aza[6]helicene 3b |
Pyrimidyl alkanol 4 |
|
ee [%] |
Yield [%] |
eec [%] |
Config. |
Reaction conditions: molar ratio 1 : 2 : i-Pr2Zn = 1 : 0.4 : 6.7 in toluene 0 °C, additional aldehyde 1 (6.7 equiv. and 26.7 equiv.) and i-Pr2Zn (20 equiv. and 53.3 equiv.) were added stepwise.
Molar ratio reaction conditions: 1 : 3 : i-Pr2Zn = 1 : 0.2 : 6.7 in toluene 0 °C, additional aldehyde 1 (6.7 equiv. and 26.7 equiv.) and i-Pr2Zn (20 equiv. and 53.3 equiv.) were added stepwise.
The ee value was determined by HPLC.
After additional rounds of asymmetric autocatalysis.14b
|
1 |
(P)-(+)-2 |
>99 |
89 |
99 (99.5)d |
S
|
2 |
(M)-(−)-2 |
>99 |
89 |
97 (99.5)d |
R
|
3 |
(P)-(+)-2 |
>99 |
85 |
77 |
S
|
4 |
(M)-(−)-2 |
>99 |
90 |
92 |
R
|
5 |
(P)-(+)-2 |
33 |
91 |
99 |
S
|
6 |
(M)-(−)-2 |
30 |
94 |
96 |
R
|
7 |
(P)-(+)-3 |
>99 |
88 |
93 |
R
|
8 |
(M)-(−)-3 |
>99 |
84 |
97 |
S
|
9 |
(P)-(+)-3 |
>99 |
72 |
91 |
R
|
10 |
(M)-(−)-3 |
>99 |
87 |
89 |
S
|
11 |
(P)-(+)-3 |
>99 |
73 |
91 |
R
|
12 |
(M)-(−)-3 |
>99 |
74 |
93 |
S
|
Unexpected results, however, were obtained when 2-aza[6]helicene 3 was used as the chiral inducer. In the presence of (P)-(+)-3, (R)-(+)-alkanol 4 with 93% ee was formed instead of (S)-(−)-4 (entry 7). The opposite enantiomer (M)-(−)-3 gave (S)-(−)-4 with 97% ee (entry 8) and, as shown in entries 9–12, these intriguing opposite enantioselectivity correlations were reproducible. Although the actual mechanism of the chirality transfer by helicenes in asymmetric autocatalysis is not clear so far, this enantioselectivity reversal induced by 2-aza[6]helicene 3 in contrast to other helicenes might be caused by the presence of an accessible coordinative nitrogen atom. As expected from the structure of 3, the steric hindrance around the 2-position of [6]helicene is much smaller than that around the 1-position. Furthermore, from the result of an X-ray crystallography analysis, 2-aza[6]helicene 3 has the larger helicity pitch than 1-aza[6]helicene 2 due to the steric repulsion between the C–H units in the 1- and 16-positions.22
‡ Accordingly, 2-aza[6]helicene 3 is proposed to be coordinated to alkyl zinc. It is worth noting that an interaction between the pyrimidine ring and zinc reagent was already considered to play a key role in the mechanism of the asymmetric autocatalysis reaction.25
Conclusions
In conclusion, we have demonstrated the asymmetric autocatalysis of pyrimidyl alkanol 4 with chiral 1- and 2-aza[6]helicenes 2 and 3. The absolute stereochemistry of the alkanol product can be controlled by the helicity of the used azahelicenes. Furthermore, we have confirmed the helicity of the enantiopure 1-aza[6]helicene 2 and 2-aza[6]helicene 3 by ECD spectra and X-ray single crystal analysis. We have found that the correlation between the helicity and enantioselectivity in asymmetric autocatalysis depends not only on the helicity of the chirality inducer but also on the position of the nitrogen atom. The mechanism of the chirality transfer remains still unclear but this unusual stereoselectivity reversal represents an interesting phenomenon that might be applied to asymmetric autocatalysis.
Acknowledgements
This work was supported in part by the T Grant-in-Aid for Scientific Research from the Promotion of Science (JSPS KAKENHI Grant Numbers 26810026 & 15H03781), the MEXT-Supported Program for the Strategic Research Foundation at Private Universities, 2012–2016, Czech Science Foundation (Reg. No. 14-29667S) and the Institute of Organic Chemistry and Biochemistry, Czech Academy of Sciences (RVO: 61388963). I. S. acknowledges the support by the Japan Society for the Promotion of Science for JSPS Fellowship. We are grateful to Miloš Tichý for the resolution of racemic azahelicenes into individual enantiomers.
Notes and references
-
(a) M. J. Narcis and N. Takenaka, Eur. J. Org. Chem., 2014, 21–34 CrossRef CAS;
(b) P. Aillard, A. Voituriez and A. Marinetti, Dalton Trans., 2014, 43, 15263–15278 RSC;
(c) N. Saleh, C. Shen and J. Crassous, Chem. Sci., 2014, 5, 3680–3694 RSC.
- For recent reviews, see:
(a)
I. G. Stará and I. Starý, in Science of Synthesis, ed. J. S. Siegel and Y. Tobe, Thieme, Stuttgart, 2010, vol. 45b, pp. 885–953 Search PubMed;
(b) Y. Shen and C.-F. Chen, Chem. Rev., 2011, 112, 1463–1535 CrossRef PubMed;
(c) M. Gingras, Chem. Soc. Rev., 2013, 42, 968–1006 RSC;
(d) M. Gingras, G. Félix and R. Peresutti, Chem. Soc. Rev., 2013, 42, 1007–1050 RSC;
(e) M. Gingras, Chem. Soc. Rev., 2013, 42, 1051–1095 RSC;
(f)
K. Tanaka, in Transition-Metal-Mediated Aromatic Ring Construction, ed. K. Tanaka, Wiley, Hoboken, 2013, pp. 281–298 Search PubMed;
(g) A. Urbano and M. C. Carreño, Org. Biomol. Chem., 2013, 11, 699–708 RSC;
(h) J. Bosson, J. Gouin and J. Lacour, Chem. Soc. Rev., 2014, 43, 2824–2840 RSC;
(i) N. Saleh, C. Shen and J. Crassous, Chem. Sci., 2014, 5, 3680–3694 RSC.
-
(a) Y. Kimura, N. Fukawa, Y. Miyauchi, K. Noguchi and K. Tanaka, Angew. Chem., Int. Ed., 2014, 53, 8480–8483 CrossRef CAS PubMed;
(b) T. Shibata, T. Uchiyama, Y. Yoshinami, S. Takayasu, K. Tsuchikama and K. Endo, Chem. Commun., 2012, 48, 1311–1313 RSC;
(c) H. Oyama, M. Akiyama, K. Nakano, M. Naito, K. Nobusawa and K. Nozaki, Org. Lett., 2016, 18, 3654–3657 CrossRef CAS PubMed;
(d) M. Yamaguchi, M. Arisawa, M. Shigeno and N. Saito, Bull. Chem. Soc. Jpn., 2016, 89, 1145–1169 CrossRef CAS.
- D. Dova, L. Viglianti, P. R. Mussini, S. Prager, A. Dreuw, A. Voituriez, E. Licandro and S. Cauteruccio, Asian J. Org. Chem., 2016, 5, 537–549 CrossRef CAS.
- S. Cauteruccio, D. Dova, M. Benaglia, A. Genoni, M. Orlandi and E. Licandro, Eur. J. Org. Chem., 2014, 2694–2702 CrossRef CAS.
- S. Cauteruccio, A. Loos, A. Bossi, M. C. B. Jaimes, D. Dova, F. Rominger, S. Prager, A. Dreuw, E. Licandro and A. S. K. Hashmi, Inorg. Chem., 2013, 52, 7995–8004 CrossRef CAS PubMed.
-
(a) K. Yavari, P. Aillard, Y. Zhang, F. Nuter, P. Retailleau, A. Voituriez and A. Marinetti, Angew. Chem., Int. Ed., 2014, 53, 861–865 CrossRef CAS PubMed;
(b) M. Gicquel, Y. Zhang, P. Aillard, P. Retailleau, A. Voituriez and A. Marinetti, Angew. Chem., Int. Ed., 2015, 54, 5470–5473 CrossRef CAS PubMed;
(c) P. Aillard, P. Retailleau, A. Voituriez and A. Marinetti, Chem. – Eur. J., 2015, 21, 11989–11993 CrossRef CAS PubMed;
(d) P. Aillard, D. Dova, V. Magné, P. Retailleau, S. Cauteruccio, E. Licandro, A. Voituriez and A. Marinetti, Chem. Commun., 2016, 52, 10984–10987 RSC.
- K. Yamamoto, T. Shimizu, K. Igawa, K. Tomooka, G. Hirai, H. Suemune and K. Usui, Sci. Rep., 2016, 6, 36211 CrossRef CAS PubMed.
- T. Tsujihara, N. Inada-Nozaki, T. Takehara, D.-Y. Zhou, T. Suzuki and T. Kawano, Eur. J. Org. Chem., 2016, 4948–4952 CrossRef CAS.
-
I. G. Sánchez, M. Šámal, J. Nejedlý, M. Karras, J. Klívar, J. Rybáček, M. Buděšínský, L. Bednárová, B. Seidlerová, I. G. Stará and I. Starý, submitted.
-
(a) M. R. Crittall, H. S. Rzepa and D. R. Carbery, Org. Lett., 2011, 13, 1250–1253 CrossRef CAS PubMed;
(b) M. R. Crittall, N. W. G. Fairhurst and D. R. Carbery, Chem. Commun., 2012, 48, 11181–11183 RSC.
- M. Šámal, J. Míšek, I. G. Stará and I. Starý, Collect. Czech. Chem. Commun., 2009, 74, 1151–1159 CrossRef.
-
(a) N. Takenaka, R. S. Sarangthem and B. Captain, Angew. Chem., Int. Ed., 2008, 47, 9708–9710 CrossRef CAS PubMed;
(b) J. Chen and N. Takenaka, Chem. – Eur. J., 2009, 15, 7268–7276 CrossRef CAS PubMed;
(c) N. Takenaka, J. Chen, B. Captain, R. S. Sarangthem and A. Chandrakumar, J. Am. Chem. Soc., 2010, 132, 4536–4537 CrossRef CAS PubMed;
(d) J. Chen, B. Captain and N. Takenaka, Org. Lett., 2011, 13, 1654–1657 CrossRef CAS PubMed;
(e) M. J. Narcis, D. J. Sprague, B. Captain and N. Takenaka, Org. Biomol. Chem., 2012, 10, 9134–9136 RSC.
-
(a) K. Soai, T. Shibata, H. Morioka and K. Choji, Nature, 1995, 378, 767–768 CrossRef CAS;
(b) I. Sato, H. Urabe, S. Ishiguro, T. Shibata and K. Soai, Angew. Chem., Int. Ed., 2003, 42, 315–317 CrossRef CAS PubMed.
-
(a) K. Soai and T. Kawasaki, Top. Curr. Chem., 2008, 284, 1–31 CrossRef CAS;
(b) T. Kawasaki and K. Soai, Bull. Chem. Soc. Jpn., 2011, 84, 879–892 CrossRef CAS;
(c)
K. Soai and T. Kawasaki, Asymmetric Autocatalysis – Discovery and State of The Art, in The Soai Reaction and Related Topic, ed. G. Palyi, C. Zicchi and L. Caglioti, Academia Nationale di Scienze Lettere e Arti Modena, Modena, Edizioni Artestampa, 2012, pp. 9–34 Search PubMed;
(d) T. Kawasaki and K. Soai, Isr. J. Chem., 2012, 52, 582–590 CrossRef CAS;
(e) T. Kawasaki, I. Sato, H. Mineki, A. Matsumoto and K. Soai, Yuki Gosei Kagaku Kyokaishi (J. Synth. Org. Chem., Jpn.), 2013, 71, 109–123 CrossRef CAS;
(f) K. Soai and T. Kawasaki, Top. Organomet. Chem., 2013, 44, 261–279 CrossRef CAS;
(g) K. Soai, T. Kawasaki and A. Matsumoto, Chem. Rec., 2014, 14, 70–83 CrossRef CAS PubMed;
(h) K. Soai, T. Kawasaki and A. Matsumoto, Acc. Chem. Res., 2014, 47, 3643–3654 CrossRef CAS PubMed.
-
(a) M. Avalos, R. Babiano, P. Cintas, J. L. Jiménez and J. C. Palacios, Chem. Commun., 2000, 887–892 RSC;
(b) D. G. Blackmond, Proc. Natl. Acad. Sci. U. S. A., 2004, 101, 5732–5736 CrossRef CAS PubMed;
(c) J. Podlech and T. Gehring, Angew. Chem., Int. Ed., 2005, 44, 5776–5777 CrossRef CAS PubMed;
(d)
J. M. Brown, I. D. Gridnev and J. Klankermayer, Amplification of Chirality, in Topics in Current Chemistry, ed. K. Soai, 2008, vol. 284, pp. 35–65 Search PubMed;
(e) T. Gehring, M. Busch, M. Schlageter and D. Weingand, Chirality, 2010, 22, E173–E182 CrossRef CAS PubMed;
(f) B. Barabás, J. Tóth and G. Pályi, J. Math. Chem., 2010, 48, 457–489 CrossRef;
(g) G. Lente, Symmetry, 2010, 2, 767–798 CrossRef CAS;
(h)
J.-C. Micheau, C. Coudret and T. Buhse, in The Soai Reaction and Related Topic, ed. G. Palyi, C. Zucchi and L. Caglioti, Accad. Nazl. Sci. Lett. Arti, Modena, Editioni Artestampa, 2012, pp. 169 Search PubMed;
(i) A. J. Bissette and S. P. Fletcher, Angew. Chem., Int. Ed., 2013, 52, 12800–12826 CrossRef CAS PubMed;
(j) O. Fülöp and B. Barabás, J. Math. Chem., 2016, 54, 10–17 CrossRef.
-
(a) I. Sato, R. Sugie, Y. Matsueda, Y. Furumura and K. Soai, Angew. Chem., Int. Ed., 2004, 43, 4490–4492 CrossRef CAS PubMed;
(b) T. Kawasaki, M. Sato, S. Ishiguro, T. Saito, Y. Morishita, I. Sato, H. Nishino, Y. Inoue and K. Soai, J. Am. Chem. Soc., 2005, 127, 3274–3275 CrossRef CAS PubMed.
-
(a) I. Sato, D. Omiya, T. Saito and K. Soai, J. Am. Chem. Soc., 2000, 122, 11739–11740 CrossRef CAS;
(b) T. Kawasaki, Y. Matsumura, T. Tsutsumi, K. Suzuki, M. Ito and K. Soai, Science, 2009, 324, 492–495 CrossRef CAS PubMed;
(c) T. Kawasaki, Y. Okano, E. Suzuki, S. Takano, S. Oji and K. Soai, Angew. Chem., Int. Ed., 2011, 50, 8131–8133 CrossRef CAS PubMed;
(d) A. Matsumoto, S. Oji, S. Takano, K. Tada, T. Kawasaki and K. Soai, Org. Biomol. Chem., 2013, 11, 2928–2931 RSC;
(e) A. Matsumoto, H. Ozaki, S. Harada, K. Tada, T. Ayugase, H. Ozawa, T. Kawasaki and K. Soai, Angew. Chem., Int. Ed., 2016, 55, 15246–15249 CrossRef CAS PubMed.
-
(a) K. Soai, S. Osanai, K. Kadowaki, S. Yonekubo, T. Shibata and I. Sato, J. Am. Chem. Soc., 1999, 121, 11235–11236 CrossRef CAS;
(b) I. Sato, K. Kadowaki and K. Soai, Angew. Chem., Int. Ed., 2000, 39, 1510–1512 CrossRef CAS;
(c) I. Sato, K. Kadowaki, H. Urabe, J. H. Jung, Y. Ono, S. Shinkai and K. Soai, Tetrahedron Lett., 2003, 44, 721–724 CrossRef CAS;
(d) H. Shindo, Y. Shirota, K. Niki, T. Kawasaki, K. Suzuki, Y. Araki, A. Matsumoto and K. Soai, Angew. Chem., Int. Ed., 2013, 52, 9135–9138 CrossRef CAS PubMed;
(e) A. Matsumoto, H. Ozawa, A. Inumaru and K. Soai, New J. Chem., 2015, 39, 6742–6745 RSC.
-
(a) I. Sato, R. Yamashima, K. Kadowaki, J. Yamamoto, T. Shibata and K. Soai, Angew. Chem., Int. Ed., 2001, 40, 1096–1098 CrossRef CAS;
(b) T. Kawasaki, K. Suzuki, E. Licandro, A. Bossi, S. Maiorana and K. Soai, Tetrahedron: Asymmetry, 2006, 17, 2050–2053 CrossRef CAS.
- S. D. Dreher, T. J. Katz, K.-C. Lam and A. L. Rheingold, J. Org. Chem., 2000, 65, 815–822 CrossRef CAS.
- J. Míšek, F. Teplý, I. G. Stará, M. Tichý, D. Šaman, I. Císařová, P. Vojtíšek and I. Starý, Angew. Chem., Int. Ed., 2008, 47, 3188–3191 CrossRef PubMed.
- M. S. Newnam, R. S. Darlak and L. Tsai, J. Am. Chem. Soc., 1967, 89, 6191–6193 CrossRef.
- D. A. Lightner, D. T. Hefelfinger, T. W. Powers, G. W. Frank and K. N. Trueblood, J. Am. Chem. Soc., 1972, 94, 3492–3497 CrossRef CAS.
-
(a) I. Sato, D. Omiya, H. Igarashi, K. Kato, Y. Ogi, K. Tsukiyama and K. Soai, Tetrahedron: Asymmetry, 2003, 14, 975–979 CrossRef CAS;
(b) G. Ercolani and L. Schiaffino, J. Org. Chem., 2011, 76, 2619–2626 CrossRef CAS PubMed;
(c) T. Gehring, M. Quaranta, B. Odell, D. G. Blackmond and J. M. Brown, Angew. Chem., Int. Ed., 2012, 51, 9539–9542 CrossRef CAS PubMed;
(d) I. D. Gridnev and A. K. Vorobiev, ACS Catal., 2012, 2, 2137–2149 CrossRef CAS;
(e) A. Matsumoto, T. Abe, A. Hara, T. Tobita, T. Sasagawa, T. Kawasaki and K. Soai, Angew. Chem., Int. Ed., 2015, 54, 15218–15221 CrossRef CAS PubMed;
(f) A. Matsumoto, S. Fujiwara, T. Abe, A. Hara, T. Tobita, T. Sasagawa, T. Kawasaki and K. Soai, Bull. Chem. Soc. Jpn., 2016, 1170–1177 CrossRef CAS.
Footnotes |
† CCDC 1521681 and 1521682. For crystallographic data in CIF or other electronic format see DOI: 10.1039/c6ob02745h |
‡ X-ray diffraction analysis was performed using a Rigaku R-AXIS RAPID II imaging plate system with a Cu rotating anode X-ray source. The Flack parameter was determined by using the Parsons quotients method (Parsons, Flack and Wagner, Acta Crystallogr., Sect. B: Struct. Sci., 2013, 69, 249–259) with SH. Due to the obtained crystal size and quality, the deviation of Flack parameters is slightly high (−0.1(4) for (M)-(−)-2 and 0.1(4) for (P)-(+)-3). However, considering the good accordance of the ECD spectrum with other helicene derivatives we conclude that the assignment of the absolute structure is unequivocal. Crystallographic data have been deposited with the Cambridge Crystallographic Data Centre as supplementary publication no. CCDC 1521682 for (M)-(−)-2 and CCDC 1521681 for (P)-(+)-3. |
§ Typical experimental procedure (Table 1, entry 1): 1-Aza[6]helicene (P)-(+)-2 (1.97 mg, 6.0 × 10−3 mmol, 0.4 equiv.) and pyrimidine-5-carbaldehyde 1 (2.8 mg, 0.015 mmol, 1 equiv.) were dissolved in 0.025 mL of toluene under an argon atmosphere. Then, diisopropylzinc in toluene (1.0 M, 0.10 mL, 0.10 mmol, 6.7 equiv.) was added dropwise over 2 h at 0 °C. After stirring overnight at 0 °C, one-pot scale up of asymmetric autocatalysis was performed by adding toluene (0.1 mL) and 1 M diisopropylzinc toluene solution (0.3 mL, 0.3 mmol) followed by the dropwise addition of aldehyde 1 (18.8 mg, 0.1 mmol) in toluene (0.5 mL) over 2 h. After 2 h stirring, the additional scale up of asymmetric autocatalysis was performed in the same way with toluene (3.5 mL), 1 M diisopropylzinc (0.8 mL, 0.8 mmol), and aldehyde 1 (75.3 mg, 0.4 mmol) in toluene (2 mL). The reaction mixture was stirred overnight, and the reaction was quenched using a mixture of sat. aq. NH4Cl and 30% aq. NH3 (2/1, v/v, 10 mL). The resulting mixture was extracted with AcOEt (3×). The combined organic layers were dried over anhydrous Na2SO4 and the volatiles were removed under reduced pressure. Purification of the residue using silica gel column chromatography (eluent: hexane/EtOAc = 2/1) gave (S)-(−)-5-pyrimidyl 4 (106.1 mg, 89% yield) with 99% ee. The ee value was determined by HPLC analysis on a chiral stationary phase (Daicel Chiralpak IB: 4.6 mm × 250 mm, 254 nm UV detector, RT, 5% 2-propanol in hexane, 1.0 mL min−1, retention time: 10.3 min for (S)-(−)-4 and 14.1 min for (R)-(+)-4). |
|
This journal is © The Royal Society of Chemistry 2017 |
Click here to see how this site uses Cookies. View our privacy policy here.