DOI:
10.1039/C7NJ03389C
(Paper)
New J. Chem., 2018,
42, 535-540
Novel polymerization of aniline and pyrrole by carbon dots
Received
7th September 2017
, Accepted 7th November 2017
First published on 7th November 2017
Abstract
The polymerization of aniline requires an initiator, such as ammonium persulfate, iron chloride (FeCl3), potassium persulfate, or silver nitrate. In this study, we introduced a novel one-pot synthesis of polyaniline using carbon dots (C-dots) and UV light that act as catalysts without any additional initiator. Chemical oxidative polymerization is a facile one-step process where C-dots are prepared from polyethylene glycol-400 (PEG-400). Polyaniline was also synthesized by employing carbon dots coated on a glass plate and irradiation of UV light. The synthesized polymer was characterized using Fourier transform infrared (FT-IR) spectroscopy, X-ray diffraction (XRD), thermogravimetric analysis (TGA), scanning electron microscopy (SEM) and solid-state NMR spectroscopy. A similar procedure also allowed the synthesis of polypyrrole from pyrrole. The approach using only carbon dots and UV light offers a novel and facile route towards the formation of polymers.
Introduction
C-dots are emerging as new materials that show great potential applications in the fields of nanomedicine and medical engineering. They are used as a new nanoplatform for cell imaging and drug delivery. C-dots exhibit outstanding fluorescence, excellent aqueous solubility, biocompatibility, biodegradation ability, negligible cytotoxicity, easy functionalization and environmental friendliness.1–5 They can be synthesized by a large variety of methods including microwave6 and ultrasonic irradiation.7,8 Remarkably, the characteristic properties of C-dots are partially dependent on the starting raw materials (such as amino acids, sugar, proteins, carbohydrates, juice, milk, glucose, etc.).9 For example, C-dots prepared from polyethylene glycol (PEG-400) show a higher quantum yield (QY) than those prepared from other precursors.7 C-dots obtained from PEG were used in various fields of science and technology owing to their high luminescence, low-cost preparation, biocompatibility, no cell cytotoxicity, high stable fluorescence and excellent photostability. In addition, the preparation of C-dots from PEG provides a good passivating agent of the surface.7,10 So far we are not aware of any report on the synthesis of polymers by using C-dots and UV light. The synthesis of conductive polymers such as polyaniline and polypyrrole relies on strong oxidizing agents as initiators. The chemical oxidative method is the best method to polymerize aniline or similar molecules. Ammonium persulfate,11–14 potassium persulfate15–17 and copper(II) chloride18,19 are among the materials used as initiators for this polymerization process. These oxidizing agents are harmful as they cause irritation to the eyes, nose, throat, lungs and skin upon contact. Persulfate salts also cause asthmatic effects. In recent years, silver nitrate (AgNO3) has also been used for the synthesis of polyaniline.20–22 It was found that the mechanism of polymer formation involves negatively charged metallic silver nanoparticles, which absorb the positively charged protonated aniline through electrostatic interactions, prior to the formation of polymers with a stable aniline silver composite polymer. It is known that silver nitrate is toxic and corrosive and when used in high concentrations can cause black color stains on the skin, irritation and even a carcinogenic effect.23 Nowadays, polyaniline is a very attractive polymer due to its good conductivity. In the field of electronics it is used as a supercapacitor.24 Polyaniline is also used in bioanalytical applications,25 such as optical devices,11 sensors,26 and selective chemiresistor gas and vapor sensors,27 and in hydrogel applications28 and liquefied petroleum gas sensing.13
C-dots form free radicals in aqueous solution, which led to their unique properties and their high potential for many applications.8,39 These free radicals are involved in the mechanism of polymerization. It is worth emphasizing that carbon dots have not been involved in catalyzing the polymerization process. In this work, we present the synthesis of polyaniline and polypyrrole from the monomers using carbon dots and UV light as initiators. The surface of the C-dots has many unshared or unpaired electrons, making it negatively charged.7 During the course of the reaction, the positively charged aniline is attracted to the carbon dots. This attraction occurs prior to the polymerization. This is to our knowledge the first report on the synthesis of polyaniline and polypyrrole using carbon dots and UV light.
Experimental section
Chemicals
Polyethylene glycol-400 (99.998%), aniline, pyrrole, and nitric acid were purchased from Sigma-Aldrich, Israel.
Preparation of C-dots
30 mL of polyethylene glycol (PEG-400) was transferred into a 50 mL beaker which was placed in a hot water bath at 70 °C. The tip of an ultrasonic transducer was dipped in PEG-400 and sonicated for 3 h with 65% amplitude.
In some experiments a glass slide (2.5 × 2.5 cm) was placed in the beaker, and sonication was continued for another 30 minutes at 25 °C in order to deposit the formed C-dots onto the glass. The glass slides were washed with water and ethanol and dried in an oven at 50 °C for 1 hour under an ambient atmosphere. The free C-dots and those adhered to the glass slide were used for the polymerization of aniline. Ultrasonic waves, and particularly micro jets, were formed after the collapse of acoustic bubbles caused by the embedding of the formed C-dots in the glass slide.
Synthesis of polyaniline (PANI)
Aniline (1.75 g) was dissolved in 50 mL of 4 M solution of nitric acid in a 100 mL beaker to start oxidation that was carried out at room temperature. Then 5 mL of an aqueous solution of C-dots was added which consists of 15 mg of the dots. The same procedure was followed to prepare polyaniline using C-dots coated on a glass plate instead of a C-dot solution. To activate the reaction, the solution was kept under UV light (USV-18 EL series UV lamp and 8 watt, 365 nm wavelength) for one week, after which we observed the formation of blackish brown solids. A high degree of polymerization was achieved and the blackish-brown precipitate was collected by filtration. The as-formed blackish-brown solids were washed several times with distilled water and dried at room temperature. PANI was also synthesized using different molar concentrations of nitric acid and aniline. Control experiments were carried out by conducting reactions without carbon dots and with UV light and with carbon dots and without UV light, and all the parameters were kept unchanged. The control reactions did not yield the blackish brown product. The terms of PANI-1 are used for the formation of polyaniline with the C-dot suspended PEG-400 and PANI-2 for the C-dot decorated glass slide, respectively. Similarly, Ppy is used to denote the polypyrrole synthesized with the suspended and embedded C-dots, respectively.
Synthesis of polypyrrole
Pyrrole (1.0 g) was dissolved in 30 mL of 1 M nitric acid in a 100 mL beaker at room temperature. 3 mL of an aqueous solution containing 9 mg of C-dots was added. The polymerization reaction was activated by illumination with UV light for three days, after which a brown solid was obtained and collected by filtration. It was washed several times with distilled water and dried at room temperature.
Analysis
Synthesized polymers were characterized using several instrumental techniques. FTIR spectra were obtained using Transon 27 in the range of 4000–400 cm−1. The crystalline properties of the synthesized materials were recorded using a Bruker AXS D8 Advance diffractometer (Bruker Inc., Germany) with a reflection θ-geometry (40 kV and 30 mA) using Cu Kα (λ = 1.5418 Å) as a radiation source, with receiving slits of 0.2 mm and a high-resolution energy-dispersive detector. The morphology of the products was observed using high resolution scanning electron microscopy (HRSEM) using an FEI Magellon 400L microscope. The samples were prepared by placing a small amount of the dried powder on a carbon tape attached to a copper plate, followed by sputtering a thin gold film to maintain good conductance. 13C solid-state NMR spectra were recorded using a Bruker 5000 UltraShield spectrometer (Bruker, Billerica, MA, USA). Thermogravimetric analysis (TGA) was performed using a Perkin Elmer instrument in the temperature range of 30 °C to 900 °C at a heating rate of 10 °C min−1. It should be emphasized that all the characterization measurements conducted for PANI2 were carried out on the product that was scrubbed from the C-dot coated glass surface.
Results and discussion
A series of experiments were conducted to characterize the various products. High-resolution transmission electron microscopy (HR-TEM) images of the C-dots show that they are spherical in shape with a relatively narrow size distribution (Fig. 1a). The average size of the C-dots was around ∼10 nm, which is in agreement with our previously prepared C-dots.7 The C-dots embedded in the glass slide are shown in Fig. 1b. The particles are of larger sizes than those formed in the solution. The particles on the glass range from 17 to 50 nm. This enhanced size of the C-dots may be due to the fact that a sonochemical coating bombards the already coated surface and adds the C-dots to the first existing layer, thus making the C-dots larger.
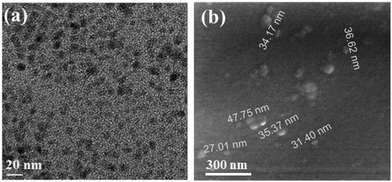 |
| Fig. 1 HRTEM images of (a) the C-dots that were separated from the PEG 400 suspension and (b) deposited on a glass side. | |
X-ray diffractograms of PANI-1 and PANI-2 are almost similar in shape (Fig. 2a). Each includes a broad band in the range of 2θ = 12 to 32 degrees, on which some sharp diffraction peaks are evident. From these diffracted patterns we observe that polyaniline is formed and the product is at least partially crystalline. The diffraction peaks are observed at 2θ = 17.8°, 18.9°, 20.9°, 23.3°, 25.6°, 27.6°, and 29.1°. The most intense diffraction peaks are observed at 21.4°, 23.3°, and 25.6°. The diffraction pattern is similar to that reported for polyaniline.12 The crystalline nature of polyaniline is a result of the presence of planes of quinoid and benzenoid rings in the polymer chain.
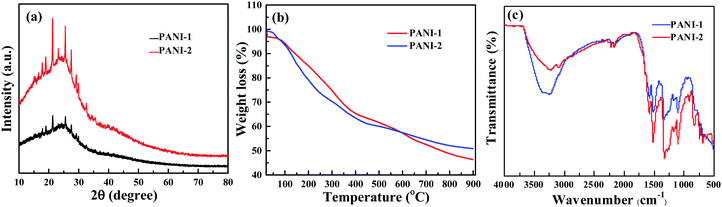 |
| Fig. 2 (a) XRD patterns, (b) TGA curves, and (c) FTIR spectra, of products (PANI-1) and (PANI-2). | |
The TGA thermograms (Fig. 2b) of polyaniline 1C-dots and polyaniline 2C-dots (which were removed from the coated glass) are similar in shape to the literature curves for PANI.15,25 Both curves PANI-1 and PANI-2 are similar to each other indicating that the products are the same.
The identification of the product as PANI is also based on the comparison of IR spectra with previous reports. Fig. 2c shows that the spectra of PANI-1 and PANI-2 are similar. The assignment of the major absorption bands is as follows: the most intense band at 3350 cm−1 corresponds to the N–H stretching vibration, and the aromatic C–H stretching band appears at 3084 cm−1. And the bands at 1582 and 1496 cm−1 are assigned to the C
C stretching mode of benzenoid and quinoid rings, respectively. The band at about 1196 cm−1 is assigned to the C–N stretching vibration and the band at 1172 cm−1 corresponds to the N
quinoid–N+ stretching vibrations. The FTIR spectrum thus confirms the formation of PANI.
The morphology of polyaniline was examined using SEM measurements. Fig. 3(a) shows that PANI-1 in solution is formed as irregular tubes, ca. 400–500 nm, whereas PANI-2 is formed as granular particles, ca. 500–600 nm in size, which is formed when the C-dots coated a glass slide (Fig. 3(b)). The reason for the larger particles on the glass is due to the sonochemical deposition that adds more CDs on the early coated particles enlarging their size.
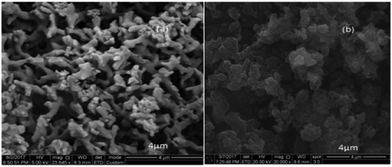 |
| Fig. 3 SEM images of (a) (PANI-1) that was formed in solution and of (b) (PANI-2) that was formed on the C-dot coated glass. | |
The nature of the surface of polyaniline products was studied using 13C solid-state NMR spectroscopy in a cross-polarization magic angle-spinning mode. The spectra of both PANI-1 and PANI-2 (Fig. 4) are similar and match well with the literature spectra for this polymer.29–31 It is composed of a broad band, centered at 128 ppm, which is composed of several peaks that appear as shoulders. The two bands marked with asterisks are the side bands. The spectral features are all assigned to the aromatic carbons of the polyaniline backbone. Both spectra exhibit six broad resonances which are observed at 116.2 ppm (shoulder), 128.2, 138.0, 146.1, 154.5, and 164.0 ppm. The peaks at 128.2 ppm and the shoulder at 116.6 ppm are assigned to carbons C-2,3 and C-6. The peaks at 138 and 164 ppm originate from C-8 protonated and C-7 non-protonated carbons of the quinoid part of the polyaniline structure, and the peaks around 146.8 ppm and 154.8 ppm are associated with C-4 and C-1 non-protonated carbons, respectively.
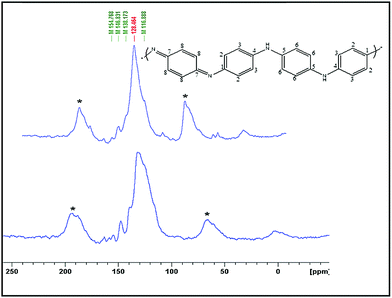 |
| Fig. 4 Solid state 13C-NMR spectra of polyaniline that was synthesized in solution (PANI-1) and on a C-dot coated glass plate (PANI-2). | |
The conductivity of a number of these polymers, dried and pressed into pellets, and was measured using a four-probe method. The conductivity of polyaniline was found to be 10 μS cm−1.
Characterization of polypyrrole
In an attempt to generalize the phenomenon of polymerization of monomers by using C-dots and UV-light, the scope of this work was extended to include more polymerization reactions. First, we tried to fabricate polypyrrole from pyrrole.
The XRD pattern of polypyrrole (Fig. 5a) shows a broad band around 2θ = 13°–45°, which is assigned to amorphous polypyrrole and is similar to the XRD pattern of polypyrrole reported in the literature.35,36 The FT-IR spectrum of the produced polymer (Fig. 5b) shows the characteristic peaks of polypyrrole: the band at 3261 cm−1 corresponds to the N–H stretching vibration, the band at 1574 cm−1 is due to the C
C in-ring stretching vibration and the band at 1130 cm−1 corresponds to the C–N stretching vibration. The bands at 1308 and 1078 cm−1 are assigned to the C–H in-plane vibration. The TGA thermogram of the polypyrrole particles (Fig. 5c) is similar in shape to the literature curves.37,38
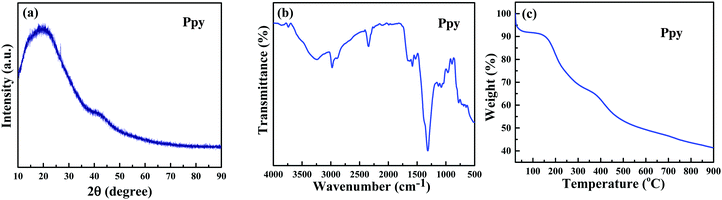 |
| Fig. 5 (a) X-ray diffractogram and (b) FT-IR of polypyrrole obtained from the polymerization of pyrrole, and (c) TGA of polypyrrole. | |
The size of the polypyrrole product was analyzed by dynamic light scattering, indicating its size as 600–900 nm. The SEM images, on the other hand, show the particles of 2–7 μm size. The disparity between SEM and DLS is explained as follows: the large micron size particles in the SEM images are aggregates of the 600 nm DLS particles. A careful look at Fig. 6b illustrates even smaller particles. The only problem is the smooth spherical shape that does not reveal any bumps on the polypyrrole surface.
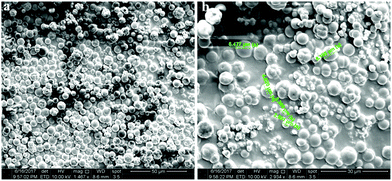 |
| Fig. 6 (a and b) SEM micrograms of polypyrrole with different magnifications. | |
13C solid state NMR spectroscopy was used for the analysis of the nature of the polypyrrole surface. The spectrum (Fig. 7) is similar to the literature spectra of this polymer,32–34 and contains four broad bands at 110, 122, 126, 129 ppm and one shoulder peak at 142. The peaks at 122 ppm and 126 are assigned to carbons C-1 and C-2, respectively, and the peak at 110 ppm originates from the protonated C-3 and non-protonated C-7 carbon of the quinoidal part of the polypyrrole structure. The peak around 129 ppm is associated with the protonated C-6 and C-4 and the non-protonated C-4 carbons, respectively.
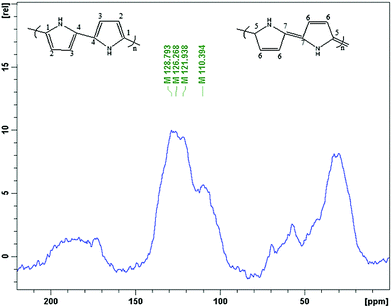 |
| Fig. 7 Solid state 13C-NMR spectrum of polypyrrole. | |
Mechanism for polymerization
The mechanism of aniline polymerization is assisted by C-dots and UV light. The dots have an unshared pair of electrons on their surface and carry negative charges.7,39 The amine group of the aniline is transformed into the protonated form by nitric acid. The reason for choosing the high concentration of nitric acid (4 M) is that it can effectively react with base, forming a phenyl ammonium ion (C6H5NH3+) at room temperature, and this ion can easily react with C-dots. Another argument is that the high concentration of the acid can accelerate both the oxidation and acidification of the amine group. Both arguments on thermodynamics and kinetics favor the high concentration of the acid.
The electronegative charges of the C-dots are adsorbed to the positively charged aniline cations through electrostatic interactions (Fig. 8). The role of UV light in this reaction is the activation of aniline, which enables more aniline molecules to form protonated species. This is well known and has been reported recently in the literature.20
 |
| Fig. 8 Schematic representation of the electrostatic interaction between the protonated aniline and the C-dots. | |
C-dots were shown to produce OH radicals in water.8 The OH radicals remove one hydrogen, forming H2O. A radical NH2+ group is left on the aromatic group. In the next stage, a proton is removed when two of these radicals interact leading to the formation of the polymer. Thus the oxidizing agent is the OH radical. Thus, UV light assists in the formation of the OH radicals. The formation of more free radicals is caused by the photoexcitation of the C-dots.
Conclusions
Polymerization of aniline and pyrrole was shown to occur in the presence of C-dots, either in solution or embedded on a glass slide, under illumination with UV light. The identity of the products and their nature were examined using XRD, FTIR spectroscopy, 13C-solid-state NMR spectroscopy, TGA and SEM, and the results were in accordance with the literature data for polyaniline and polypyrrole. The products were obtained in the shapes of microspheres. This appears to be the first demonstration of a polymerization process achieved using C-dots and UV light, without the use of additional reagents. The success of such a simple approach presenting unique opportunities in polymer chemistry was never presented before for the polymerization process.
Conflicts of interest
There are no conflicts to declare.
References
- Y. Wang and A. Hu, Carbon quantum dots: synthesis, properties and applications, J. Mater. Chem., 2014, 2(34), 6921–6939 CAS.
- M. Fu, F. Ehrat, Y. Wang, K. Z. Milowska, C. Reckmeier, A. L. Rogach and J. Feldmann, Carbon dots: a unique fluorescent cocktail of polycyclic aromatic hydrocarbons, Nano Lett., 2015, 15(9), 6030–6035 CrossRef CAS PubMed.
- P. Innocenzi, L. Malfatti and D. Carboni, Graphene and carbon nanodots in mesoporous materials: an interactive platform for functional applications, Nanoscale, 2015, 7(30), 12759–12772 RSC.
- S. Khan, A. Gupta, N. C. Verma and C. K. Nandi, Time-resolved emission reveals ensemble of emissive states as the origin of multicolor fluorescence in carbon dots, Nano Lett., 2015, 15(12), 8300–8305 CrossRef CAS PubMed.
- X. T. Zheng, A. Ananthanarayanan, K. Q. Luo and P. Chen, Glowing graphene quantum dots and carbon dots: properties, syntheses, and biological applications, Small, 2015, 11(14), 1620–1636 CrossRef CAS PubMed.
- C. López, M. Zougagh, M. Algarra, E. Rodríguez-Castellón, B. B. Campos, J. E. da Silva and A. Ríos, Microwave-assisted synthesis of carbon dots and its potential as analysis of four heterocyclic aromatic amines, Talanta, 2015, 132, 845–850 CrossRef PubMed.
- V. B. Kumar, Z. E. Porat and A. Gedanken, Facile one-step sonochemical synthesis of ultrafine and stable fluorescent C-dots, Ultrason. Sonochem., 2016, 28, 367–375 CrossRef CAS PubMed.
- V. B. Kumar, I. Perelshtein, A. Lipovsky, Z. E. Porat and A. Gedanken, The sonochemical synthesis of Ga@C-dots particles, RSC Adv., 2015, 5(32), 25533–25540 RSC.
- Q. Xu, T. Kuang, Y. Liu, L. Cai, X. Peng, T. S. Sreeprasad and N. Li, Heteroatom-doped carbon dots: synthesis, characterization, properties, photoluminescence mechanism and biological applications, J. Mater. Chem. B, 2016, 4(45), 7204–7219 RSC.
-
S. H. Yeom, Temperature-dependent Tunable Photoluminescence Properties of Carbon Nanodots Derived from Polyethylene Glycol, University of Kentucky, 2014, pp. 1–52.
- A. F. Mansour, A. Elfalaky and F. A. Maged, Synthesis, Characterization and Optical properties of PANI/PVA Blends, IOSR J. Appl. Phys., 2015, 7, 37–45 Search PubMed.
- W. Lin, K. Xu, M. Xin, J. Peng, Y. Xing and M. Chen, Hierarchical porous polyaniline–silsesquioxane conjugated hybrids with enhanced electrochemical capacitance, RSC Adv., 2014, 4(74), 39508–39518 RSC.
- P. T. Patil, R. S. Anwane and S. B. Kondawar, Development of electrospun polyaniline/ZnO composite nanofibers for LPG sensing, Procedia Mater. Sci., 2015, 10, 195–204 CrossRef CAS.
- H. Kawashima and H. Goto, Preparation and properties of polyaniline in the presence of trehalose, Soft Nanosci. Lett., 2011, 1(3), 71–75 CrossRef CAS.
- S. Ahmad, A. Sultan, W. Raza, M. Muneer and F. Mohammad, Boron nitride based polyaniline nanocomposite: Preparation, property, and application, J. Appl. Polym. Sci., 2016, 133(39), 43989 CrossRef.
- R. Abdelkader, H. Amine and B. Mohammed, Structure and Physicochemical Properties of Polyaniline Synthesized in Presence of Maghnite clay Catalyst layered (Algerian Montmorillonite), Res. J. Med. Med. Sci., 2013, 1(3), 1–6 Search PubMed.
- M. Khadra, S. Taha and Y. Fellag Bouteflika, Polyaniline/maghnite clay synthesized by aniline in sulfuric acid using potassium persulfate (PPS), ISJ African J. Chem., 2016, 3(2), 169–175 Search PubMed.
- R. Ullah, G. A. Bowmaker, J. Travas-Sejdic, K. Ali and A. U. H. A. Shah, Synthesis and Characterization of Polyaniline by Using Weak Oxidizing Agent, Macromol. Symp., 2014, 339(1), 84–90 CrossRef CAS.
- R. Ullah, G. A. Bowmaker, C. Laslau, G. I. Waterhouse, Z. D. Zujovic, K. Ali and J. Travas-Sejdic, Synthesis of polyaniline by using CuCl 2 as oxidizing agent, Synth. Met., 2014, 198, 203–211 CrossRef CAS.
- S. M. Reda and S. M. Al-Ghannam, Synthesis and electrical properties of polyaniline composite with silver nanoparticles, Adv. Mater. Phys. Chem., 2012, 2(02), 75 CrossRef CAS.
- P. Bober, M. Trchová, J. Prokeš, M. Varga and J. Stejskal, Polyaniline–silver composites prepared by the oxidation of aniline with silver nitrate in solutions of sulfonic acids, Electrochim. Acta, 2011, 56(10), 3580–3585 CrossRef CAS.
- N. V. Blinova, J. Stejskal, M. Trchová, I. Sapurina and G. Ćirić-Marjanović, The oxidation of aniline with silver nitrate to polyaniline–silver composites, Polymer, 2009, 50(1), 50–56 CrossRef CAS.
- New Jersey Department of Health, Hazardous substance fact sheet, May 2009, available: http://nj.gov/health/eoh/rtkweb/documents/fs/1672.pdf, accessed 28 May 2017.
- G. K. Veerasubramani, K. Krishnamoorthy, S. Radhakrishnan, N. J. Kim and S. J. Kim,
In situ chemical oxidative polymerization of aniline monomer in the presence of cobalt molybdate for supercapacitor applications, J. Ind. Eng. Chem., 2016, 36, 163–168 CrossRef CAS.
- V. Gautam, A. Srivastava, K. P. Singh and V. L. Yadav, Preparation and characterization of polyaniline, multiwall carbon nanotubes, and starch bionanocomposite material for potential bioanalytical applications, Polym. Compos., 2015, 38, 3 Search PubMed.
- R. Kumar, S. Singh and B. C. Yadav, Conducting Polymers: Synthesis, Properties and Applications, International Advanced Research Journal in Science, Engineering and Technology, 2015, 2, 11 Search PubMed.
- S. Pandey, Highly sensitive and selective chemiresistor gas/vapor sensors based on polyaniline nanocomposite: a comprehensive review, Journal of Science: Advanced Materials and Devices, 2016, 1(4), 431–453 CrossRef.
- X. Shi, Y. Hu, K. Tu, L. Zhang, H. Wang, J. Xu and M. Xu, Electromechanical polyaniline–cellulose hydrogels with high compressive strength, Soft Matter, 2013, 9(42), 10129–10134 RSC.
- Z. D. Zujovic, G. A. Bowmaker, H. D. Tran and R. B. Kaner, Solid-state, NMR of polyaniline nanofibers, Synth. Met., 2009, 159(7), 710–714 CrossRef CAS.
- S. K. Sahoo, R. Nagarajan, S. Roy, L. A. Samuelson, J. Kumar and A. L. Cholli, An enzymatically synthesized polyaniline: a solid-state NMR study, Macromolecule, 2004, 37(11), 4130–4138 CrossRef CAS.
- Z. D. Zujovic, M. Gizdavic-Nikolaidis and G. A. Bowmaker, Solid-state NMR of polyanilines with different morphologies, Chemistry, 2013, 87–91 CAS.
- F. Devreux, G. Bidan, A. A. Syed and C. Tsintavis, Solid state 13C NMR in conducting polymers, J. Phys., 1985, 46(9), 1595–1601 CAS.
- M. Forsyth, V. T. Truong and M. E. Smith, Structural characterization of conducting polypyrrole using 13C cross-polarization/magic-angle spinning solid-state nuclear magnetic resonance spectroscopy, Polymer, 1994, 35(8), 1593–1601 CrossRef CAS.
- J. M. Pringle, D. R. MacFarlane and M. Forsyth, Solid state NMR analysis of polypyrrole grown in a phosphonium ionic liquid. Synthetic metals, Synth. Met., 2005, 155(3), 684–689 CrossRef CAS.
- X. Chen, L. Liu, Z. Yan, Z. Huang, Q. Zhou, G. Guo and X. Wang, The excellent cycling stability and superior rate capability of polypyrrole as the anode material for rechargeable sodium ion batteries, RSC Adv., 2016, 6(3), 2345–2351 RSC.
- J. G. Wang, B. Wei and F. Kang, Facile synthesis of hierarchical conducting polypyrrole nanostructures via a reactive template of MnO2 and their application in supercapacitors, RSC Adv., 2014, 4(1), 199–202 RSC.
- X. Fan, Z. Yang and N. He, Hierarchical nanostructured polypyrrole/graphene composites as super capacitor electrode, RSC Adv., 2015, 5(20), 15096–15102 RSC.
- T. Dai and Y. Lu, Polypyrrole hydro-sponges built up from mesoscopic scales, J. Mater. Chem., 2007, 17(45), 4797–4802 RSC.
- C. Yu, G. Cuicui, F. Ge Fang, T. Xin, M. Xiaochuan, W. Tao and G. Wayne, Crossover between Anti- and Pro-oxidant Activities of Graphene Quantum Dots in the Absence or Presence of Light, ACS Nano, 2016, 10, 8690–8699 CrossRef PubMed.
|
This journal is © The Royal Society of Chemistry and the Centre National de la Recherche Scientifique 2018 |