Variations in serum concentrations of selected organochlorines among delivering women in Argentina. The EMASAR study†
Received
19th June 2017
, Accepted 27th September 2017
First published on 29th September 2017
Abstract
The EMASAR study is the first study to describe the body burden of OCs in Argentinian women after delivery. In total, 698 maternal serum samples from Salta (n = 498) and Ushuaia (n = 200) were collected in 2011–2012 and analyzed for a total of 7 polychlorinated biphenyls (PCBs) and 12 pesticide-related compounds. Only 11 of the compounds had detection rates above 60% in one or both places. Compared with Ushuaian women, those from Salta exhibited higher lipid-adjusted concentrations of p,p′-DDE, p,p′-DDT, β-HCH, and PCB 118 (p ≤ 0.003), with no differences in concentrations of PCB 153 and 138. After controlling for age, parity and heritage (born in the province or migrated there from other regions of Argentina), concentrations of p,p′-DDE, p,p′-DDT, β-HCH and all PCBs were significantly higher in Salta natives compared with Ushuaia natives or migrants (p ≤ 0.010). No variations between native and migrated Ushuaian women were observed other than for PCB 153 (6.1 versus 8.6 μg kg−1 lipid, p = 0.022). Age was generally associated positively with the body burden of nearly all OCs and parity negatively so, with p,p′-DDD, o,p′-DDT, and o,p′-DDD residues and α-HCH in Ushuaia being the exceptions. The regional differences in OC concentrations are explained by contrasting domestic sources, historical and current uses, industrial emissions, dietary patterns and lifestyle factors, as well as long-range-transport. The relatively high PCB 118/PCB 180 ratio observed for both Argentinian communities likely reflects the use of technical mixtures with congener-specific composition. In a comprehensive comparison with other countries, the Argentinian OC concentrations were mostly in the lower range. It is concluded that a latitude effect equivalent to that operative in the Arctic region seems unlikely.
Environmental significance
Knowledge about human exposure to organochlorine compounds (OCs) in Southern Hemisphere countries is limited. Polychlorinated biphenyls (PCBs) and pesticides in the sera of delivering women in Salta (a northwestern province) and Ushuaia (the most southern province) of Argentina reveal regional differences in concentrations and these are explained by contrasting domestic sources, historical and current uses, industrial emissions, dietary patterns, lifestyle factors and long-range-transport. A comprehensive comparison with other countries indicates that the Argentinian OC concentrations were mostly in the lower range.
|
1. Introduction
Most OCs‡ were synthesized and used in the Northern Hemisphere.1,2 Furthermore, the Arctic has become a repository for contaminants due to what is referred to as the latitude effect that involves atmospheric transportation and ocean currents.3 As a consequence, most studies of the body burdens of OCs in humans have been conducted among Northern Hemisphere populations.3 Comparable information for southern-latitude countries is limited. The Southern American continent constitutes a potential sink for OCs from global sources.4 Environmental studies of air, sediments, soil, water, wildlife and biota have indeed regarded their occurrence there as a consequence of both global transport and past local uses.5–9 DDT and HCHs have been used in agriculture, while PCBs have been linked to inputs from power plants, industrial activities, combustion and dumping.5,6,9 Not surprisingly, these pollutants have entered the domestic food web.4,6,10,11 Argentina ratified the Stockholm Convention that banned DDT, HCB and HCHs in agriculture uses since the late 1990s, as well as placing restrictions or bans on PCBs in 2001 (ref. 4) and more recently on the use of the insecticide dicofol.4,9,10
OCs are persistent, lipophilic, toxic and bioaccumulate in the food chain.3 The concentration of contaminants in maternal blood and breast milk provides important information on the exposure of the fetus and newborn, who are especially vulnerable. Endocrine disruption, reproductive effects, and impairment of immunologic development constitute worries and their adverse toxic effects may threaten human health (including trans-generational impairments).3
Knowledge about human exposure to OCs in South America is limited.12 The current study describes the body burden of OCs in Argentinian delivering women, and our findings are assessed in the context of comparable studies elsewhere. Our working hypothesis is that a phenomenon similar to the Arctic latitude-transport effect is not operative in the Southern Hemisphere.
2. Materials and methods
2.1 Description of the study area and data collection
The EMASAR study was conducted in the Argentinian cities of Ushuaia and Salta (for map see Fig. 1 in Økland et al. 2017).13 Ushuaia (54.80° S, 68.30° W) is the southernmost city in the world and the capital of the Tierra del Fuego in Antártida e Islas del Atlántico Sur Province. It has a population of some 60
000 and features electronics manufacturing, fisheries, natural gas and oil extraction, sheep farming, and tourism. Salta (24.78° S, 65.42° S) is the capital of the northwestern-highland Salta province. The city has around 620
000 citizens, with agriculture and related industrial activities being the main economic activities (for additional details see Økland et al., 2017).13
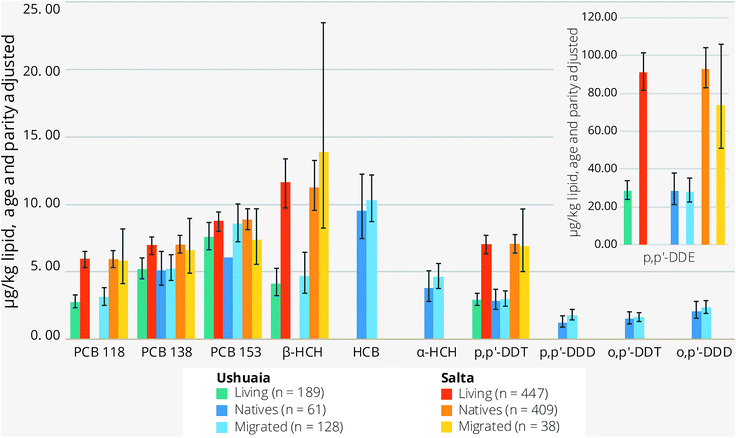 |
| Fig. 1 Age- and parity-adjusted maternal serum OC concentrations (μg kg−1 lipid, geomean) with 95% CI error bars, stratified by residence (Ushuaia and Salta) and maternal birthplace (native or migrated) for compounds with a detection frequency > 60%. City differences (Ushuaia versus Salta) at p < 0.001 except for PCB 153 (p = 0.081). The p-values for differences between native and migrated groups are provided in Table 2; for full names of the compounds, see Section 2.2. | |
The field work was conducted in the period April 2011 to March 2012. Of the total 698 delivering women enrolled, the current study component is limited to 670 subjects (199 from Ushuaia and 471 from Salta). One person from Ushuaia and 27 from Salta were excluded due to the lack of serum lipid concentrations. Through interviews, participants completed questionnaires covering personal characteristics, socioeconomic factors, obstetrical and breastfeeding history, environmental, health and lifestyle conditions, and dietary intake. Non-fasting maternal blood samples, height and weight were obtained at 36 ± 12 hours following delivery (median 1 day, range 0–3 days). Details about the study profile, population anthropometric measurements and blood sampling procedures have been provided previously.13
EMASAR is a collaborative project between UiT The Arctic University of Norway, the Stavanger University Hospital (Norway) and the two Argentinian partners, namely Clínica San Jorge in Ushuaia (a private institution co-responsible with a public hospital for the in-hospital deliveries in the city and the surrounding region), and the Hospital Público Materno Infantil in Salta (a public institution responsible for all in-hospital deliveries in the city and the region).
The study (#2010/7317) was approved by the Ethics Committee of the Salta Medical Association and the Ministries of Health in both provinces. As required by law, the Norwegian Regional Committee for Medical and Health Research Ethics (REC North) approved the study (#2011/706), and it was conducted in accordance with the Helsinki declaration. Informed consent was obtained for any experimentation with human subjects in the study.
2.2 Chemical analysis
Blood collected in BD Vacutainers® (BD SST II Plus Advance 10/8.5 ml) was sampled and centrifuged at 2000 relative centrifugal force (RCF) for 10 minutes. The maternal serum was then transferred into vials (Sarstedt CryoPure, 2.0 ml tubes) for general analyses, or into glass vials (4.0 ml, pre-rinsed with n-hexane/acetone) retained for chemical analyses of the POPs. The serum samples were transferred in a frozen state to the EMASAR Biobank at the Faculty of Health Sciences, UiT The Arctic University of Norway, Tromsø, Norway.
The analytical work was conducted at the Department of Environmental Chemistry, Institute of Environmental Assessment and Water Research (IDAEA-CSIC), Barcelona, Catalonia, Spain. Serum samples were analysed for the following organochlorines (OCs): pentachlorobenzene (PeCB); α- and β-HCB (hexachlorobenzene); α-, β- δ- and γ-HCH (hexachlorocyclohexane); 1,1,1-trichloro-2-(o-chlorophenyl)-2-(p-chlorophenyl)ethane (o,p′-DDT); 1,1,1-trichloro-2,2-bis(p-chlorophenyl)ethane (p,p′-DDT); 1,1-dichloro-2-(o-chlorophenyl)-2-(p-chlorophenyl)ethane (o,p′-DDD); 1,1-dichloro-2,2-bis(p-chlorophenyl)ethane (p,p′-DDD); 1,1-dichloro-2-(o-chlorophenyl)-2-(p-chlorophenyl)ethylene (o,p′-DDE); 1,1-dichloro-2,2-bis(p-chlorophenyl) ethylene (p,p′-DDE); and polychlorinated biphenyl (PCB) congeners 28, 52, 101, 118, 138, 153 and 180. The analytical procedures employed have been described elsewhere by Bravo et al. (2017).14 Briefly, recovery standards TBB and PCB-209, n-hexane and concentrated H2SO4 were added to the serum samples (1 ml). After vortexing and centrifugation, repeated extraction of the serum n-hexane layer followed and the combined extracts were reduced to near dryness. The remaining solution was quantitatively transferred to gas chromatograph (GC) vials using four 25 μl rinses of isooctane and the injection standard PCB-142 (20 μl) was added. Instrumental analysis was performed by gas chromatography with electron capture detection. Compound identification was confirmed by analysis with a GC coupled to a mass spectrometer. A blank was analysed for every 6 samples. The recoveries of the surrogate standards were 76 ± 13% and 82 ± 14% for PCB-30 and PCB-200, respectively. The measured concentrations of the compounds were adjusted for the recoveries of these standards. The limits of detection were calculated as three times the standard deviation of the noise measured next to the chromatographic peaks of each analyte: they ranged from 0.01 to 0.027 μg l−1 (Table 1). Moreover, the analytical methods fared well (within 20% variability of the consensus values) in the AMAP Ring Test Proficiency Program for Persistent Organic Pollutants in human serum conducted by the Centre de Toxicologie Institut National de Santé Publique du Québec.15
Table 1 Concentrations (in μg kg−1 lipid) and limits of detection of OCs in serum samples of delivering mothers from Argentina (2011–2012)
Compounda |
LODa |
Ushuaia (n = 199) |
Salta (n = 471) |
p-Valueb |
% ≥ LOD |
GM |
AM |
Median |
Min |
Max |
% ≥ LOD |
GM |
AM |
Median |
Min |
Max |
For the full names of the compounds see Section 2.2; the limit of detection (LOD) is in μg l−1.
Mann Whitney test for compounds with a detection frequency > 60%.
Detection frequency < 20%.
|
PCB28 |
0.010 |
32.7 |
1.3 |
2.1 |
0.9 |
0.3 |
14.2 |
24.0 |
1.2 |
1.7 |
0.9 |
0.4 |
17.4 |
|
PCB52 |
0.005 |
38.2 |
1.04 |
2.84 |
0.47 |
0.21 |
37.9 |
33.1 |
0.94 |
2.68 |
0.50 |
0.22 |
59.3 |
|
PCB101 |
0.001 |
56.3 |
0.70 |
2.59 |
0.72 |
0.06 |
25.2 |
16.1 |
0.18 |
0.46 |
0.13 |
0.06 |
16.1 |
|
PCB118 |
0.011 |
64.8 |
3.1 |
5.6 |
3.8 |
0.5 |
66.9 |
79.0 |
5.7 |
9.1 |
7.1 |
0.6 |
163 |
<0.001 |
PCB138 |
0.002 |
97.0 |
6.32 |
8.84 |
7.06 |
0.13 |
45.4 |
97.0 |
6.36 |
9.91 |
7.04 |
0.18 |
172 |
0.900 |
PCB153 |
0.008 |
97.0 |
9.13 |
12.19 |
9.80 |
0.48 |
51.7 |
94.3 |
8.08 |
11.60 |
8.80 |
0.48 |
144 |
0.091 |
PCB180 |
0.011 |
36.7 |
1.7 |
3.2 |
1.0 |
0.4 |
38.2 |
30.1 |
1.7 |
3.4 |
1.1 |
0.5 |
62.5 |
|
p,p′-DDT |
0.005 |
89.4 |
3.22 |
14.29 |
3.47 |
0.31 |
1950 |
97.0 |
6.83 |
12.32 |
6.32 |
0.31 |
334 |
<0.001 |
p,p′-DDE |
0.013 |
99.0 |
38.5 |
196.5 |
32.7 |
1.2 |
23 800 |
100 |
80.2 |
228.3 |
69.0 |
3.69 |
12 100 |
<0.001 |
p,p′-DDD |
0.002 |
81.4 |
1.63 |
2.89 |
2.41 |
0.13 |
71.5 |
52.0 |
0.60 |
1.17 |
0.67 |
0.09 |
23.3 |
|
o,p′-DDT |
0.005 |
66.3 |
1.61 |
2.71 |
2.22 |
0.26 |
15.3 |
15.7 |
0.55 |
0.94 |
0.43 |
0.20 |
30.5 |
|
o,p′-DDE |
0.013 |
47.7 |
2.2 |
3.4 |
1.4 |
0.5 |
22.5 |
15.3 |
1.4 |
2.2 |
1.2 |
0.6 |
57.5 |
|
o,p′-DDD |
0.007 |
68.8 |
2.31 |
4.02 |
2.97 |
0.32 |
29.9 |
24.2 |
0.95 |
1.56 |
0.66 |
0.30 |
21.3 |
|
∑DDT |
|
|
60.0 |
223.8 |
49.9 |
8.1 |
25 900 |
|
99.5 |
246.5 |
83.2 |
9.7 |
12 400 |
<0.001 |
PeCB |
0.006 |
22.6 |
0.72 |
1.18 |
0.52 |
0.20 |
11.8 |
4.20 |
—c |
—c |
0.51 |
0.26 |
9.7 |
|
HCB |
0.027 |
80.9 |
10.0 |
19.0 |
9.8 |
1.4 |
499 |
57.1 |
5.9 |
8.9 |
7.1 |
1.5 |
102 |
|
α-HCH |
0.007 |
86.9 |
4.42 |
7.64 |
4.84 |
0.37 |
59.9 |
33.5 |
1.44 |
4.77 |
0.67 |
0.32 |
47.2 |
|
β-HCH |
0.010 |
61.3 |
6.0 |
21.2 |
7.8 |
0.5 |
281 |
71.3 |
9.4 |
31.0 |
13.7 |
0.5 |
483 |
0.003 |
δ-HCH |
0.020 |
17.6 |
2.1 |
3.7 |
1.7 |
0.7 |
45.7 |
19.5 |
2.7 |
6.0 |
1.8 |
0.9 |
74.4 |
|
γ-HCH |
0.013 |
19.1 |
—c |
—c |
1.1 |
0.4 |
24.7 |
1.1 |
—c |
—c |
1.1 |
0.6 |
26.6 |
|
Chemical analyses of the serum lipid profile (Økland et al., 2017)13 were done enzymatically at the respective hospitals. Total lipid contents were calculated from the cholesterol and triglyceride concentrations: total lipids = 90 + 1.3 (cholesterol + triglyceride) mg dl−1.16
2.3 Statistical analysis
Statistical analyses were carried out using the IBM SPSS Statistics for Windows statistical package version 24 (SPSS Inc. Chicago, IL, USA). Descriptive details are reported as means, standard deviations, median and range or percentage, and relationships between variables were explored using Spearman's rank correlation analysis. Concentrations below the LOD were replaced by LOD/2. Our choice to limit the between-group statistical analyses to compounds with a prevalence > 60% is recommended by the US CDC.17 Despite log
10 transformation, the OC distributions remained skewed positively to the right and were not normally distributed according to the Kolmogorov–Smirnov test and Q–Q residual plots. We therefore employed non-parametric statistics (the Mann–Whitney U test) for comparisons of OC concentrations between the two study sites. For related compounds, Levene's test demonstrated equal variances between the two study sites. In a univariate general linear model (UGML) adjusted for the continuous variables age and parity (excluding stillbirths), acceptable case-wise diagnostics were possible even though abruptions of normality were evident based on histograms, the Kolomogorov–Smirnov test and residual plots. Parity (excluding stillbirths) and months of lactation were highly correlated (Spearman's ρ = 0.937), with the former displaying the highest correlation with OC concentrations; it was thus selected in the modelling. Nevertheless, lactation yielded similar results (not reported). To harmonize the group size, and thus the distribution, bootstrapping with 2000 estimates was selected. Between city comparisons (Ushuaia versus Salta) and maternal birthplaces involved four groups: (i) born and living in Ushuaia (natives, n = 61); (ii) living in Ushuaia, but born in another province within Argentina (migrated, n = 128); (iii) living and born in Salta (natives, n = 409); and (iv), living in Salta but born in another province within Argentina (n = 38). In the regression model, the coefficients for the OCs were estimates of the mean difference in log-transformed concentrations between each group (city or heritage) and the reference level. The regression coefficients were back-transformed (10β) to reflect the ratios of change in concentrations, and in the text are described as % change = (10β − 1) × 100. The significant levels were set at p < 0.05. Finally, the ratios within the DDT group were calculated to distinguish ongoing chronic exposure versus dietary exposure or distant past exposure.3,18
3. Results
3.1 Personal characteristics
The personal characteristics of all participating women (n = 698) are described elsewhere13 and include details about mothers (e.g., age, marital status, education, employment, smoking habits, diet, and vitamin intake) and newborns. As mentioned, 28 participants were excluded from the current study cohort. With reference to Økland et al. (2017),13 Salta women were younger, had somewhat higher parity, breastfed longer, had less education, and differed somewhat in dietary habits. Details about migration from other regions of Argentina into the provinces of Ushuaia and Salta were also available for 189 and 446 participants, respectively. Almost everyone in Salta (91%) was born in the province compared to 32% in Ushuaia. Details about age, parity, breastfeeding and years of living in current home are reported in ESI Table S1.†
3.2 Influence of area residence and maternal birth place in OC serum concentrations
3.2.1 Detection frequency of OCs.
The detection limits and frequencies are reported in Table 1. Among the 19 OCs analyzed, only six compounds had a detection rate above 60% in both places, specifically PCB 118, PCB 138 and PCB 153, p,p′-DDT, p,p′-DDE and β-HCH. Five additional compounds exceeded this threshold in Ushuaia, namely p,p′-DDD, o,p′-DDT, o,p′-DDD, HCB, and α-HCH. We selected p,p′-DDE, PCB 138, PCB 153 and p,p′-DDT which exhibited detection frequencies (>89%) for detailed evaluations, and a somewhat more inclusive group when considering maternal birthplaces (Table S2†).
3.2.2 Serum lipid-adjusted OC concentrations by city of residence.
Of the OCs, lipid-adjusted serum p,p′-DDE (μg kg−1 lipid) was the dominant compound at both sites (Table 1) followed by PCB 153 (respectively, lower by factors of 4.3 in Ushuaia and 9.9 in Salta based on GMs). Compared to Ushuaia, serum concentrations of PCB 118, β-HCH, p,p′-DDT, p,p′-DDE and ∑DDT were all higher (p < 0.003) in Salta, while there were no city differences for PCB 153 and 138. Wet-weight serum OC concentrations are summarized in Table S3.†
3.2.3 Impact of residence and place of birth on age- and parity-adjusted OC concentrations.
The age- and parity-adjusted concentrations of the dominant OCs (ng per lipids) by area of residence are shown in Fig. 1 and are further stratified whether born in the two study areas or migration into them from other regions of Argentina (n = 636). Through bootstrapping, differences in OC concentrations between the Ushuaia and Salta women were shown to be distinct (p < 0.001), with the exception of PCB 153 (p = 0.081).
Several significant statistical differences (p ≤ 0.010) were observed when considering residence history in terms of being native born or migrants to the two regions of Argentina (Table 2). Relative to Salta natives, those of Ushuaia had 27% lower concentrations of PCB 138 and 32% of PCB 153; and migrants into Ushuaia also exhibited reduced concentrations of PCB 118 and PCB 138 (respectively, by 47% and 25%), but not so for PCB 153. In addition, Ushuaian migrants had 46% less serum PCB 118 than those in Salta. The p,p′-DDE and p,p′-DDT concentrations for Ushuaian natives and migrants were less substantive by 60% and 70% when compared to the respective groups in Salta (Table 3). Ushuaia migrants had 58% lower concentrations of β-HCH compared to Saltanean natives (post hoc analysis). Intra-city differences in OC concentrations between native and migrated women in Salta did not reach statistical significance; nor for Ushuaians, with the exception that PCB concentrations in native citizens were on average 29% lower (Table 2; also see Table S4†). Age had a positive influence on OC concentrations and parity a negative one (p < 0.05). The statistical significance for these variables was lost for Ushuaia residents when examining each community separately (e.g., Table S5†), but persisted for all OCs in Salta (data not shown).
Table 2 Fractional change in serum concentrations (in μg kg−1 lipid) of OCs by place of maternal birth, adjusted for age and paritya,b,c
Groups |
n
|
PCB 153 |
p-Value |
CI 95% |
PCB 138 |
p-Value |
CI 95% |
PCB 118 |
p-Value |
CI 95% |
GM |
Ratio |
GM |
Ratio |
GM |
Ratio |
Univariate analyses of variance models based on detection frequencies above 60% in each population group and bootstrap with p-values and 95% CI based on 2000 samples.
Blanks indicate detection frequencies below 60%, and the corresponding data were excluded from the analyses.
Bonferroni post hoc pairwise comparisons: for PCB 153, the Ushuaia natives/Ushuaia migrated concentration ratio was 0.71, p = 0.022; for PCB 118, the Ushuaia migrated/Salta migrated ratio was 0.54, p = 0.004; and for both p,p′-DDT and p,p′-DDE, the Ushuaia natives and Ushuaia migrated/Salta migrated ratios were ∼0.40, p < 0.001.
|
Ushuaia natives |
61 |
6.07 |
0.68 |
0.008
|
0.52 |
0.91 |
5.14 |
0.73 |
0.010
|
0.57 |
0.93 |
|
|
|
|
|
Ushuaia migrated |
128 |
8.55 |
0.96 |
0.679 |
0.81 |
1.14 |
5.25 |
0.75 |
0.008
|
0.61 |
0.91 |
3.13 |
0.53 |
<0.001
|
0.42 |
0.67 |
Salta natives |
409 |
8.87 |
Ref. |
|
|
|
7.01 |
Ref. |
|
|
|
5.93 |
Ref. |
|
|
|
Salta migrated |
38 |
7.36 |
0.83 |
0.200 |
0.61 |
1.13 |
6.62 |
0.94 |
0.625 |
0.77 |
1.19 |
5.83 |
0.98 |
0.929 |
0.68 |
1.40 |
Age, year |
|
|
1.05 |
<0.001
|
1.03 |
1.06 |
|
1.06 |
<0.001
|
1.04 |
1.07 |
|
1.02 |
0.013
|
1.01 |
1.04 |
Parity |
|
|
0.82 |
<0.001
|
0.76 |
0.87 |
|
0.78 |
<0.001
|
0.72 |
0.84 |
|
0.88 |
0.001
|
0.81 |
0.95 |
Groups |
n
|
p,p′-DDT |
p-Value |
CI 95% |
p,p′-DDE |
p-Value |
CI 95% |
β-HCH |
p-Value |
CI 95% |
GM |
Ratio |
GM |
Ratio |
GM |
Ratio |
Ushuaia natives |
61 |
2.88 |
0.41 |
<0.001
|
0.30 |
0.53 |
28.4 |
0.31 |
<0.001
|
0.22 |
0.43 |
|
|
|
|
|
Ushuaia migrated |
128 |
3.00 |
0.42 |
<0.001
|
0.35 |
0.51 |
28.2 |
0.30 |
<0.001
|
0.24 |
0.38 |
4.7 |
0.42 |
<0.001
|
0.28 |
0.62 |
Salta natives |
409 |
7.06 |
Ref. |
|
|
|
92.9 |
Ref. |
|
|
|
11.2 |
Ref. |
|
|
|
Salta migrated |
38 |
6.93 |
0.98 |
0.864 |
0.78 |
1.24 |
73.5 |
0.79 |
0.206 |
0.56 |
1.14 |
13.9 |
1.23 |
0.424 |
0.71 |
2.11 |
Age, year |
|
|
1.03 |
0.001
|
1.01 |
1.04 |
|
1.08 |
<0.001
|
1.06 |
1.10 |
|
1.09 |
<0.001
|
1.06 |
1.12 |
Parity |
![[thin space (1/6-em)]](https://www.rsc.org/images/entities/char_2009.gif) |
![[thin space (1/6-em)]](https://www.rsc.org/images/entities/char_2009.gif) |
0.92 |
0.012
|
0.86 |
0.97 |
![[thin space (1/6-em)]](https://www.rsc.org/images/entities/char_2009.gif) |
0.65 |
<0.001
|
0.60 |
0.70 |
|
0.56 |
<0.001
|
0.50 |
0.64 |
Table 3 Spearman's rho (ρ) for interrelationships between serum concentrations of OCs by city of residence (n = 636)a
|
|
PCB 118 |
PCB 138 |
PCB 153 |
p,p′-DDT |
p,p′-DDE |
p,p′-DDD |
o,p′-DDD |
o,p′-DDT |
HCB |
α-HCH |
Results are presented for compounds with a detection frequency > 60%. ** Significant correlation at the 0.01 or * 0.05 level (2-tailed).
|
Ushuaia (n = 199) |
PCB 138 |
0.632**
|
|
|
|
|
|
|
|
|
|
PCB 153 |
0.595**
|
0.758**
|
|
|
|
|
|
|
|
|
p,p′-DDT |
0.222**
|
0.339**
|
0.301**
|
|
|
|
|
|
|
|
p,p′-DDE |
0.259**
|
0.510**
|
0.465**
|
0.381**
|
|
|
|
|
|
|
p,p′-DDD |
0.073 |
−0.106 |
0.118 |
0.172*
|
−0.046 |
|
|
|
|
|
o,p′-DDD |
0.417**
|
0.180*
|
0.148*
|
0.046 |
0.084 |
0.177*
|
|
|
|
|
o,p′-DDT |
0.103 |
−0.008 |
0.142 |
0.197**
|
0.021 |
0.646**
|
0.119 |
|
|
|
HCB |
0.192**
|
0.348**
|
0.233**
|
0.249**
|
0.464**
|
−0.002 |
−0.070 |
0.011 |
|
|
α-HCH |
0.099 |
−0.060 |
0.114 |
−0.115 |
−0.064 |
0.534**
|
0.305**
|
0.401**
|
−0.046 |
|
β-HCH |
0.292**
|
0.388**
|
0.445**
|
0.151*
|
0.474**
|
−0.073 |
0.029 |
0.050 |
0.321**
|
−0.068 |
Salta (n = 471) |
PCB 138 |
0.589**
|
|
|
|
|
|
|
|
|
|
PCB 153 |
0.592**
|
0.899**
|
|
|
|
|
|
|
|
|
p,p′-DDT |
0.223**
|
0.420**
|
0.343**
|
|
|
|
|
|
|
|
p,p′-DDE |
0.124**
|
0.414**
|
0.384**
|
0.564**
|
|
|
|
|
|
|
β-HCH |
0.288**
|
0.411**
|
0.379**
|
0.312**
|
0.553**
|
0.205**
|
|
|
|
|
3.3 Ratios of DDT-isomers
The p,p′-DDE/p,p′-DDT ratio was rather similar in Ushuaia (mean 22.8, median 11.4) and Salta (mean 19.2, median 11.6) women (p > 0.05, Table S4†). In both places, around 18% of the women had a ratio below 5.0. By comparison, the respective median ratios (0.09) of p,p′-DDT/p,p′-DDE were identical (p > 0.05); three women in Ushuaia and five in Salta exceeded a ratio of 1.00. In addition, the mean o,p′-DDT/p,p′-DDT ratio was calculated only for samples with concentrations above the LOD (n = 183) and had values of 1.09 (Ushuaia) and 0.29 (Salta) with p < 0.001.
3.4 Correlations of OC concentrations
Based on Spearman's rho tests, significant inter-OC correlations might be designated as weak (ρ < 0.4) to moderate (ρ 0.4–0.9) for the 5 OCs compared in Salta (Table 3). The most robust relationships occurred between PCB congeners (ρ = 0.59–0.90). For Ushuaia, the intra-PCB relationships were again the most robust (ρ = 0.60–0.76); others were mostly weak or absent.
3.5 Global comparisons
Our OC concentration data are summarized and compared to those reported for other regions of the world in Fig. 2–4; the corresponding world-wide data are compiled in Tables S6a–e and in Tables S6f & g† for PCB 138 and 118. This overview roughly covers a 10 year period (2004–2014). It is clear that the observed concentrations for the Ushuaia and Salta study participants are in the middle to lower range.
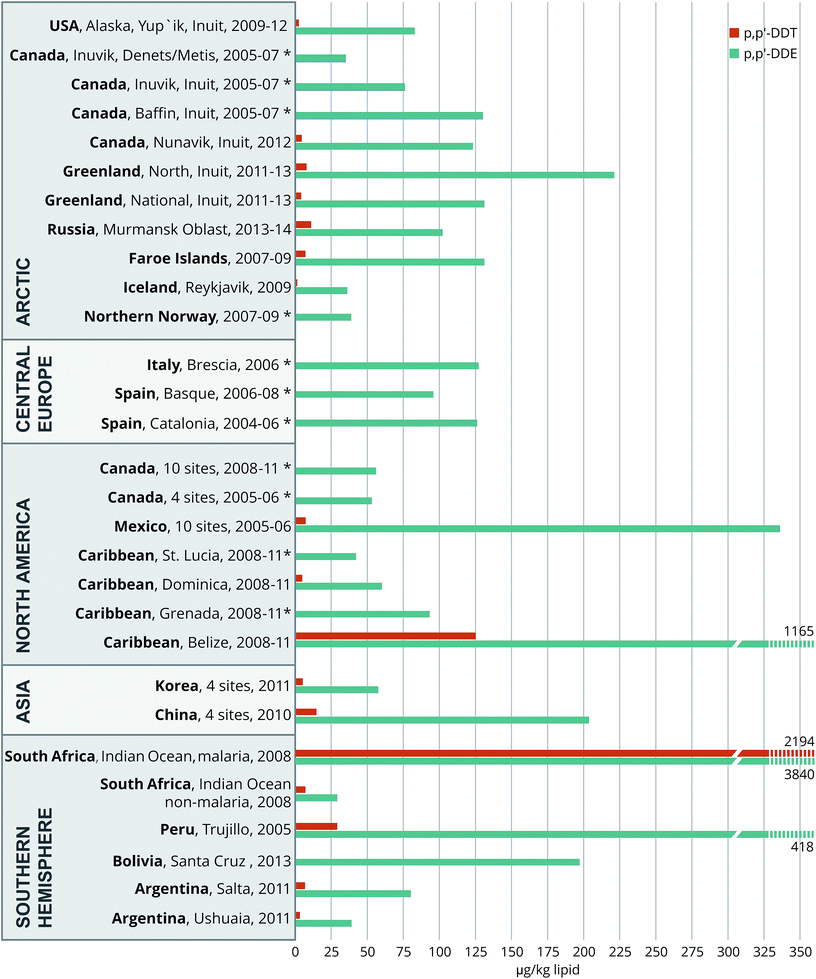 |
| Fig. 2 Worldwide comparisons of serum or plasma p,p′-DDT and p,p′-DDE (μg kg−1 lipid, geomean) of pregnant or delivering women for the 2004–2014 period. *p,p′-DDT, not reported due to high numbers of non-detections. For details and references, see the text and ESI S6 a and b.† | |
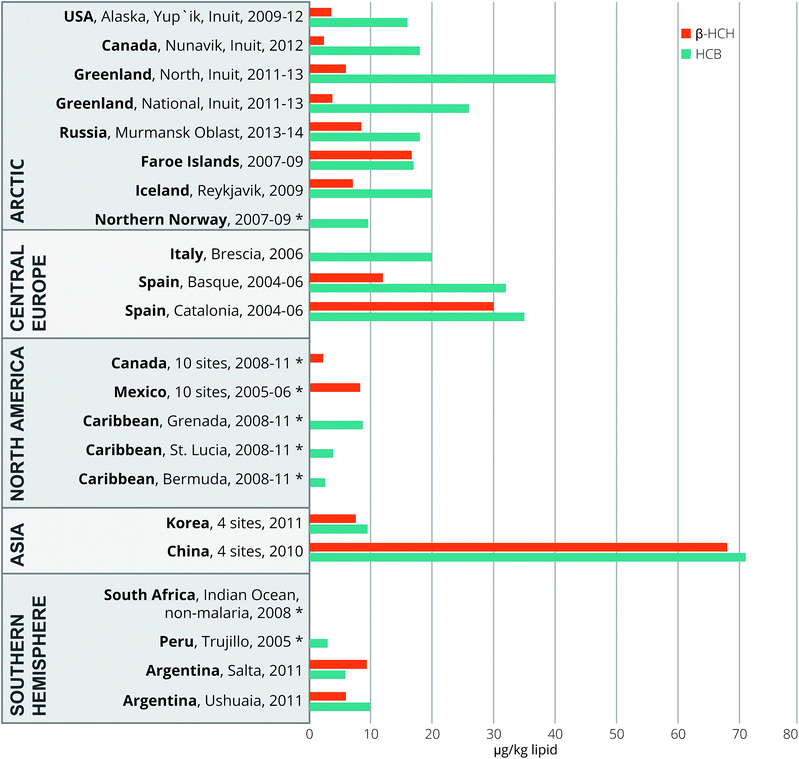 |
| Fig. 3 Worldwide comparisons of serum or plasma β-HCH and HCB (μg kg−1 lipid, geomean) of pregnant or delivering women for the 2004–2014 period. *Not reported due to high numbers of non-detections. For details and references, see the text and ESI S6c and d.† | |
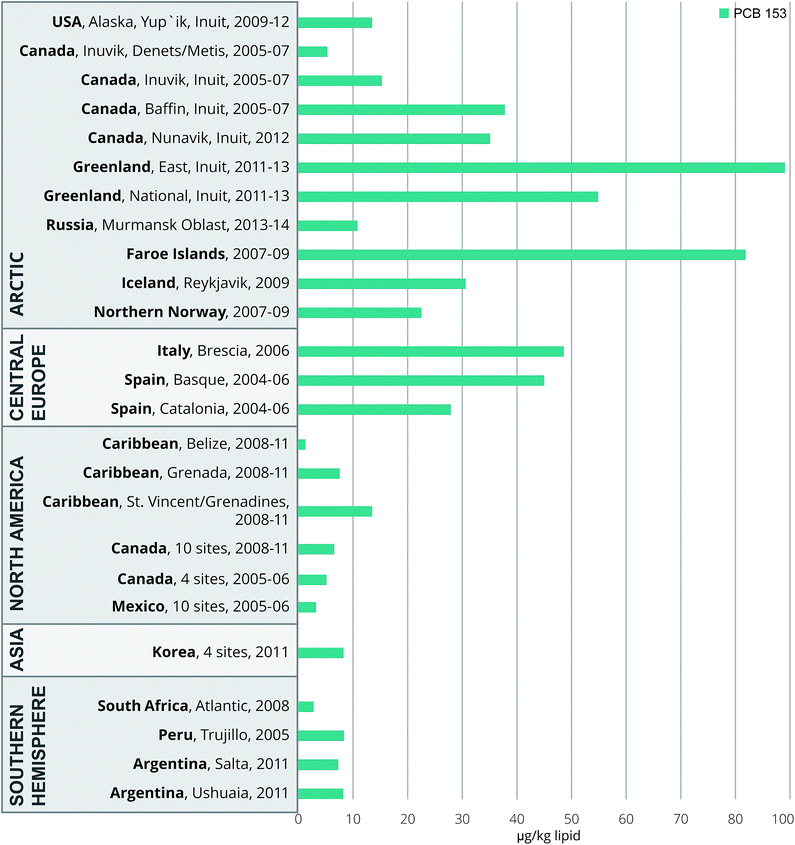 |
| Fig. 4 Worldwide comparisons of serum or plasma PCB 153 (μg kg−1 lipid, geomean) of pregnant or delivering women for the 2004–2014 period. For details and references, see the text and ESI S6e.† | |
4. Discussion
4.1 Preamble
In addition to comparing our serum OC concentrations with those reported for other regions of the world, the influences of the following predictor variables are discussed below: maternal age, parity, breastfeeding history, province lived in and of birth (specifically, within the provinces of Ushuaia and Salta or migration into them from other Argentinian provinces), as well as dietary and environmental predictor variables.
4.2 The signature of the OCs in a global and regional comparison
4.2.1 DDT-group.
In general, the observed maternal lipid-adjusted serum concentrations of p,p′-DDE in Salta may be designated as moderate when compared with those reported for other world areas (Fig. 2). By comparison, concentrations for Ushuaian mothers were in the lower range and comparable to those reported for Arctic populations not consuming marine mammals,3 although somewhat higher than the lowest observed such as for the residents of the coast of non-malaria South Africa.19 The concentrations for Saltanean mothers were comparable to those reported for Alaska Yup'ik and for Baffin Island and Canadian Inuits living in Inuvik,3 although higher than in Korea20 and central Canadian cities21 – and both above and below those reported for the Caribbean islands.22 The Argentinian maternal levels were moderately lower than in Western Australia (wet weight, wwt),23 central Europe like Spain24 and Italy,25 and considerably lower than in Bolivia,26 China,27 and Arctic women with a traditional diet of marine mammals.3 By contrast, the observed concentrations were considerably lower than in countries with historical use of DDT and subsequent prohibition such as Mexico28 and Peru,17 Vietnam (wwt)29 and Belize.22 Malaria-endemic areas of South Africa with active DDT treatments have reported the highest concentrations in contrast to the low levels in the neighboring non-malaria Indian Ocean region.19
The Argentinian p,p′-DDT concentrations observed (Fig. 2) were lower than in countries with a previous or current history of DDT usage.17,19,22,27,29 Comparable p,p′-DDT levels to those in Salta have been observed in Mexico,28 Northern Greenland and Faroe Island3 and the non-malaria Indian Ocean area in South Africa.19 The Ushuaian levels were slightly higher than those found in Alaska and Iceland.3 Finally, our observed concentrations of DDT-related compounds were higher (p,p′-DDE), comparable (p,p′-DDT) or lower (p,p′-DDD) than those reported for an adult population of Buenos Aires in 2006.12
Relative to Ushuaia, the higher concentrations of p,p′-DDT (and thus also p,p′-DDE) in Salta are in agreement with the nearly ten-fold higher use of insecticides in the home and perhaps in agriculture.13 Several decades ago this compound was used for malaria vector control in the northwestern regions.4 The current presence of low levels p,p′-DDT and the relatively high concentrations of its derivative p,p′-DDE point to past comprehensive use and ongoing entry into the local food web.4,6,7,9 The observed p,p′-DDE/p,p′-DDT ratio of around 20 in the two communities (Table S4†) is consistent with historical use of the latter.18 Nevertheless, chronic or more recent exposure to DDT cannot be excluded as one in five women in both communities had ratios below 5. The o,p′-DDT/p,p′-DDT ratio is a useful index in regions with active use of dicofol.30 In Ushuaia, the relatively high o,p′-DDT/p,p′-DDT ratio of 1.09 compared to 0.29 in Salta (Table S4†) suggests some recent DDT input,18 and is likely related to dicofol use.30–32 Atmospheric release of dicofol in the province of Mendoza in western-central Argentina has also been reported,9 and this insecticide still appears to be in use.10 Furthermore, long-range transport of the relatively volatile Dicofol from neighboring regions and countries, or other southern continents, might also have some influence.33,34
4.2.2 HCB and the HCH-group.
The observed concentrations of HCB and β-HCH (Table 1) were mostly in the low range compared to other jurisdictions (Fig. 3). In Ushuaia, the concentrations of both compounds were comparable to those for the women residents of Korea20 and HCB concentrations were comparable to those reported in Northern Norway.35 Serum HCB concentrations for mothers in Italy,25 Spain24 and remote Arctic regions3 were up to two-fold higher, and up to six-fold in China.27
Before the use of pure lindane (γ-HCH) technical mixtures were used, which encompassed isomeric compositions of 60–70% α-HCH, 5–12% β-HCH and 10–15% γ-HCH.36 Generally speaking, data on human maternal body burdens of α-HCH are scarce and are low or below the detection limit for mothers in China,27 Australia,23 Canada and Mexico.28 Our Argentinian levels (Table 1), particularly those in Ushuaia, are comparable in magnitude to those reported in South Africa (2 μg kg−1 lipid)37 and China (5.2 μg kg−1 lipid).38 As depicted in Fig. 3, the observed β-HCH concentrations were higher than those from Canada, Greenland and Alaska,3 comparable to those from Mexico,28 Korea20 and South Africa,39 but lower than those reported for the Faroe Island3 and Spain.24 The high concentrations observed in China are consistent with its extensive organochlorine pesticide production and use.27 The generally low β-HCH concentrations among Arctic populations contrast those of other OCs, and perhaps reflect decades of worldwide restrictions and prohibitions.
The observed HCB, α-HCH and β-HCH concentrations likely reflect regional diversities in past emissions. HCB is still released into the Argentinian environment as a by-product of pesticide use, various industrial activities, open burning processes, waste disposal and landfills.9 The 3-fold higher α-HCH levels in Ushuaia, and its lack of a relationship with HCB, PCBs, p,p′-DDT and p,p′-DDE (Table 3) may reflect source-specific pathways. It is known that α-HCH is retained less in nature and humans than β-HCH.36 Their comparable concentrations in Ushuaia mothers remain unexplained, as is the observation in China that α-HCH levels exceeded those of β-HCH.38 Atmospheric long-range transportation of this semi-volatile HCH to colder climates may be relevant. Furthermore, the relatively higher exposure to the water-insoluble β-HCH in Salta suggests greater historical local usage of HCH pesticides compared with more central and southern remote areas. The positive correlations of β-HCH at both sites with both PCBs and p,p′-DDE (see Table 3) suggest common sources of exposure, while the scarcity of γ-HCH is consistent with its lower bioaccumulation potential and more rapid degradation in nature.36 By contrast, a study in South Africa showed almost non-detectable maternal concentrations of HCB, α-HCH and β-HCH and a complete predominance of γ-HCH – this was explained by on-going use of lindane.37
4.2.3 PCB-congeners.
PCB congeners 153 and 138 were the dominant PCBs, and this is like the footprint observed elsewhere. Furthermore, the observed PCB 118/PCB 180 ratio of 2–3 contrasts those observed elsewhere, such as in the Arctic (though with some Russian observations exempted),3 but is in line with previous studies within Argentina.6 Presumably this ratio reflects local use of technical mixtures with congener-specific composition.6,11
Despite prohibitions, Argentinian electric power-generation equipment, aged industrial plants, stockpiles and the other sources already mentioned continue to be sources of PCBs and continue to impact the environment and thus the food chain.9,11 However from a global perspective, the Argentinian production and use of PCBs have been low. It is estimated that only 3% of the total global historical use of PCB occurred in the Southern Hemisphere, with Argentina contributing 0.1%.40 This is consistent with the data for PCB 153 in Fig. 4.
4.3 Factors influencing OC concentrations
Generally speaking, we conclude that the exposures in Salta for the dominant OCs exceeded those in Ushuaia, with PCB 153 exempted. Taken together, the relatively robust inter-correlation patterns for PCBs, p,p′-DDE and β-HCH in both communities (Table 3) suggest more or less a common exposure pathway. However, in Ushuaia they are somewhat more limited for α-HCH, p,p′-DDD and o,p′-residues and suggest additional sources. The impact of inter-country migration was not strong, but nevertheless measurable. Ushuaian migrants had considerably lower serum PCB 118, p,p′-DDE and p,p′-DDT than those who had moved into Salta. Interestingly, PCB-153 appears to have contributed considerably to the overall population body burden of PCBs in Ushuaia. Geographic differences in OC levels as observed likely also reflect history, socioeconomic, culture, physiological factors, lifestyle and dietary differences.22,24,41–43
Age as a positive predictor of serum OC concentrations and parity and previous breastfeeding as negative ones are well understood.35,44 Because of the relatively long half-lives (in years) of OCs (typically > 7 years),45 body burdens increase over time. OC storage in lipid tissues and loss via breastfeeding explain the observed negative impact of parity.
In Argentina, dietary foodstuffs are typically produced within the nation, but with regional differences. Extensive importation of foods into Ushuaia suggests an additional contrasting factor. OCs are often associated with animal lipid-rich aquatic and terrestrial food web sources, including dairy products and eggs, or even fruit, vegetables and grains.35,41,46 As indicated by others6 and confirmed in our recent publication,13 fish contributed only 10–15% of the Argentinian diet. Argentinian studies have also suggested that freshwater species contain OCs due to the pollution of rivers.6,11 Consumption of fatty meats and freshwater fish has been identified as a dietary predictor variable of OCs in Argentinian women.47 Such sources would likely affect the inland residents of Salta more.
Salta province in the north has a subtropical climate, while Tierra del Fuego is at a lower latitude and has a cooler climate. Its prevailing winds are from the south-west, and thus a latitude effect equivalent to that operative in the Arctic region seems unlikely. However, Ushuaia's expanding industry, economy, population and tourism constitute environmental challenges that include heavily polluted waterfronts and limited waste-disposal systems.8
4.4 Strengths and limitations
The present study of OCs in two distinct provinces of Argentina uses the methodological and analytical approaches employed in AMAP studies and our laboratory participated successfully in the most recent AMAP Analytical Ring Test.15 Nearly all invited women participated, and the sample sizes for the two regions are comparable to those for other population monitoring studies. We consider the representativeness of our sample set as acceptable. Our findings would facilitate the planning of other surveys of other regions of Argentina, as its generalizability might best be limited to the investigated regions.
Low detection frequencies for some compounds might have introduced some bias into the statistical analyses. A few cases of extreme values of DDTs were also found, but sensitivity analyses supported their inclusion. We acknowledge that our comparisons of our data with those of multiple studies are subject to uncertainties related to varying methodological and analytical variances. However, most of the publications referred to employed the AMAP study approach, and the respective laboratories have participated in the mentioned Inter-laboratory Ring test.3 Our use of lipid-adjusted concentrations in our comparisons might have helped to minimize bias such as that associated with different pregnancy sampling periods.18,44
5. Conclusions
To the best of our knowledge, the EMASAR study is the first conducted in Argentina on OC concentrations in the sera of women related to pregnancy. Overall, our results indicate that the serum OC concentrations in Argentinian delivering women were in the lower range. Even so, the geographical distributions of the DDTs, HCB, HCHs and PCBs for the Argentinian southernmost and northwest mothers are inferred to reflect differences in domestic sources, regional diversity in historical and current uses, prior and current industrial emissions, and potential contributions of disposal sites, stockpiles and long-range transfer. Due to the wide range of pesticide use, some individuals are deemed to be at higher risk. Compared to other countries, the natives of Ushuaia in the Tierra del Fuego province are considered to be subject to lower levels of POPs than in many other countries. By comparison, the exposure of the Salta group is considered to be moderately elevated. The relatively high PCB 118/PCB 180 ratio observed for both Argentinian communities contrasts that seen elsewhere and likely reflects the use of technical mixtures with congener-specific composition.
Funding
We acknowledge The Norwegian Department of Foreign Affairs and the Arctic Monitoring and Assessment Programme (AMAP) (2011/706-13) for generously funding the study.
Conflicts of interest
There are no conflicts to declare.
Acknowledgements
The authors acknowledge the Health Ministries of the provinces Salta and Tierra del Fuego, Argentina for their administrative support and facilitation. We acknowledge Dr Martin de la Arena for his assistance in obtaining the ethics approval and initiating the study in Argentina, Bente A. Augdal for designing the biological sampling procedures and managing the study biobank at the UiT, Dra. Silvia dib Ashur for implementing the biological sampling routines, and Lic. Maria José Aleman (Salta) and Maria Florence Bressan (Ushuaia) for their key roles in the local administration of the study. Finally, we acknowledge the participants from Ushuaia and Salta.
References
- K. Breivik, A. Sweetman, J. M. Pacyna and K. C. Jones, Towards a global historical emission inventory for selected PCB congeners – a mass balance approach. 1. Global production and consumption, Sci. Total Environ., 2002, 290, 181–198 CrossRef CAS PubMed.
- K. Breivik, R. Alcock, Y. F. Li, R. E. Bailey, H. Fiedler and J. M. Pacyna, Primary sources of selected POPs: regional and global scale emission inventories, Environ. Pollut., 2004, 128, 3–16 CrossRef CAS PubMed.
-
AMAP, AMAP Assessment 2015: Human Health in the Arctic, Arctic Monitoring and Assessment Programme (AMAP), Oslo, Norway, 2015, pp. 1–165, http://www.amap.no/documents/doc/AMAP-Assessment-2015-Human-Health-in-the-Arctic/1346 Search PubMed.
-
UNEP, Regionally Based Assessment of Persistent Toxic Substances, Eastern and Western South America Regional Report, Unites Nations, Switzerland, 2002, pp. 1–91, http://www.oas.org/dsd/Quimicos/South%20American%20Report%202.pdf Search PubMed.
- K. S. Miglioranza, M. Gonzalez, P. M. Ondarza, V. M. Shimabukuro, F. I. Isla, G. Fillmann, J. E. Aizpun and V. J. Moreno, Assessment of Argentinean Patagonia pollution: PBDEs, OCPs and PCBs in different matrices from the Rio Negro basin, Sci. Total Environ., 2013, 452–453, 275–285 CrossRef CAS PubMed.
- P. M. Ondarza, M. Gonzalez, G. Fillmann and K. S. Miglioranza, PBDEs, PCBs and organochlorine pesticides distribution in edible fish from Negro River basin, Argentinean Patagonia, Chemosphere, 2014, 94, 135–142 CrossRef CAS PubMed.
- P. Torres, K. S. Miglioranza, M. M. Uhart, M. Gonzalez and M. Commendatore, Organochlorine pesticides and PCBs in Southern Right Whales (Eubalaena australis) breeding at Peninsula Valdes, Argentina, Sci. Total Environ., 2015, 518–519, 605–615 CrossRef CAS PubMed.
- O. A. Amin, L. I. Comoglio and J. L. Sericano, Polynuclear aromatic and chlorinated hydrocarbons in mussels from the coastal zone of Ushuaia, Tierra del Fuego, Argentina, Environ. Toxicol. Chem., 2011, 30, 521–529 CrossRef CAS PubMed.
- D. Allende, M. Ruggeri, B. Lana, K. Garro, J. Altamirano and E. Puliafito, Inventory of primary emissions of selected persistent organic pollutants to the atmosphere in the area of Great Mendoza, Emerging Contaminants, 2016, 2, 14–25 CrossRef.
- M. Gonzalez, K. S. Miglioranza, J. E. Aizpun de Moreno and V. J. Moreno, Evaluation of conventionally and organically produced vegetables for high lipophilic organochlorine pesticide (OCP) residues, Food Chem. Toxicol., 2005, 43, 261–269 CrossRef CAS PubMed.
- P. M. Ondarza, M. Gonzalez, G. Fillmann and K. S. Miglioranza, Increasing levels of persistent organic pollutants in rainbow trout (Oncorhynchus mykiss) following a mega-flooding episode in the Negro River basin, Argentinean Patagonia, Sci. Total Environ., 2012, 419, 233–239 CrossRef CAS PubMed.
-
A. S. Ridolfi, G. B. Alvarez and M. E. Rodriuez Girault, in Bioremediation in latin America: Current Research and Perspectives, ed. A. Alvarez and M. A. Polti, Springer International Publishing, Switzerland, 2014, ch. 2, pp. 17–40, DOI:10.1007/978-3-319-05738-5_2.
- I. Økland, J. O. Odland, S. Matiocevich, M. V. Alvarez, T. Aarsland, E. Nieboer and S. Hansen, The Argentinian mother-and-child contaminant study: a cross-sectional study among delivering women in the cities of Ushuaia and Salta, Int. J. Circumpolar Health., 2017, 76, 1364598 CrossRef PubMed.
- N. Bravo, S. Hansen, I. Okland, M. Gari, M. V. Alvarez, S. Matiocevich, J. O. Odland and J. O. Grimalt, Influence of maternal and sociodemographic characteristics on the accumulation of organohalogen compounds in Argentinian women. The EMASAR study, Environ. Res., 2017, 158, 759–767 CrossRef CAS PubMed.
- INSPQ, https://www.inspq.qc.ca/node/1729, accessed Aug, 2017.
- L. Rylander, P. Nilsson-Ehle and L. Hagmar, A simplified precise method for adjusting serum levels of persistent organohalogen pollutants to total serum lipids, Chemosphere, 2006, 62, 333–336 CrossRef CAS PubMed.
- O. Adetona, K. Horton, A. Sjodin, R. Jones, D. B. Hall, M. Aguillar-Villalobos, B. E. Cassidy, J. E. Vena, L. L. Needham and L. P. Naeher, Concentrations of select persistent organic pollutants across pregnancy trimesters in maternal and in cord serum in Trujillo, Peru, Chemosphere, 2013, 91, 1426–1433 CrossRef CAS PubMed.
- C. R. Kirman, L. L. Aylward, S. M. Hays, K. Krishnan and A. Nong, Biomonitoring equivalents for DDT/DDE, Regul. Toxicol. Pharmacol., 2011, 60, 172–180 CrossRef CAS PubMed.
- K. Channa, H. B. Rollin, T. H. Nost, J. O. Odland and T. M. Sandanger, Prenatal exposure to DDT in malaria endemic region following indoor residual spraying and in non-malaria coastal regions of South Africa, Sci. Total Environ., 2012, 429, 183–190 CrossRef CAS PubMed.
- S. Kim, J. Park, H. J. Kim, J. J. Lee, G. Choi, S. Choi, S. Kim, S. Y. Kim, H. B. Moon, S. Kim and K. Choi, Association between several persistent organic pollutants and thyroid hormone levels in serum among the pregnant women of Korea, Environ. Int., 2013, 59, 442–448 CrossRef CAS PubMed.
- M. Fisher, T. E. Arbuckle, C. L. Liang, A. LeBlanc, E. Gaudreau, W. G. Foster, D. Haines, K. Davis and W. D. Fraser, Concentrations of persistent organic pollutants in maternal and cord blood from the maternal-infant research on environmental chemicals (MIREC) cohort study, Environ. Health, 2016, 15, 59 CrossRef PubMed.
- M. S. Forde, E. Dewailly, L. Robertson, E. A. Laouan Sidi, S. Cote, P. Dumas and P. Ayotte, Prenatal exposure to persistent organic pollutants and polybrominated diphenyl ethers in 10 Caribbean countries, Environ. Res., 2014, 133, 211–219 CrossRef CAS PubMed.
- A. Reid, A. Callan, A. Stasinska, J. Heyworth, D. T. Phi, J. O. Odland and A. Hinwood, Maternal exposure to organochlorine pesticides in Western Australia, Sci. Total Environ., 2013, 449, 208–213 CrossRef CAS PubMed.
- J. Ibarluzea, M. Alvarez-Pedrerol, M. Guxens, L. S. Marina, M. Basterrechea, A. Lertxundi, A. Etxeandia, F. Goni, J. Vioque, F. Ballester, J. Sunyer and I. Project, Sociodemographic, reproductive and dietary predictors of organochlorine compounds levels in pregnant women in Spain, Chemosphere, 2011, 82, 114–120 CrossRef CAS PubMed.
- R. Bergonzi, C. Specchia, M. Dinolfo, C. Tomasi, G. De Palma, T. Frusca and P. Apostoli, Distribution of persistent organochlorine pollutants in maternal and foetal tissues: data from an Italian polluted urban area, Chemosphere, 2009, 76, 747–754 CrossRef CAS PubMed.
- J. P. Arrebola, M. Cuellar, J. P. Bonde, B. Gonzalez-Alzaga and L. A. Mercado, Associations of maternal o,p′-DDT and p,p′-DDE levels with birth outcomes in a Bolivian cohort, Environ. Res., 2016, 151, 469–477 CrossRef CAS PubMed.
- H. Guo, Y. Jin, Y. Cheng, B. Leaderer, S. Lin, T. R. Holford, J. Qiu, Y. Zhang, K. Shi, Y. Zhu, J. Niu, B. A. Bassig, S. Xu, B. Zhang, Y. Li, X. Hu, Q. Chen and T. Zheng, Prenatal exposure to organochlorine pesticides and infant birth weight in China, Chemosphere, 2014, 110, 1–7 CrossRef CAS PubMed.
- B. Adlard, K. Davis, C. L. Liang, M. S. Curren, S. Rodriguez-Dozal, H. Riojas-Rodriguez, M. Hernandez-Avila, W. Foster, L. Needham, L. Y. Wong, J. P. Weber, L. Marro, T. Leech and J. Van Oostdam, Persistent organic pollutants (POPs) and metals in primiparous women: a comparison from Canada and Mexico, Sci. Total Environ., 2014, 500–501, 302–313 CrossRef CAS PubMed.
- S. Hansen, J. O. Odland, D. T. Phi, E. Nieboer and T. M. Sandanger, Maternal levels of organochlorines in two communities in southern Vietnam, Sci. Total Environ., 2009, 408, 225–232 CrossRef CAS PubMed.
- S. Zhou, L. Tong, Q. Tang, X. Gu, B. Xue and W. Liu, Residues, sources and tissue distributions of organochlorine pesticides in dog sharks (Mustelus griseus) from Zhoushan Fishing Ground, China, Mar. Pollut. Bull., 2013, 73, 374–380 CrossRef CAS PubMed.
- C. Turgut, C. Gokbulut and T. J. Cutright, Contents and sources of DDT impurities in dicofol formulations in Turkey, Environ. Sci. Pollut. Res. Int., 2009, 16, 214–217 CrossRef CAS PubMed.
- X. Qiu, T. Zhu, B. Yao, J. Hu and S. Hu, Contribution of dicofol to the current DDT pollution in China, Environ. Sci. Technol., 2005, 39, 4385–4390 CrossRef CAS PubMed.
- X. Qiu, T. Zhu, J. Li, H. Pan, Q. Li, G. Miao and J. Gong, Organochlorine pesticides in the air around the Taihu Lake, China, Environ. Sci. Technol., 2004, 38, 1368–1374 CrossRef CAS PubMed.
- K. S. Miglioranza, J. E. Aizpun de Moreno and V. J. Moreno, Dynamics of organochlorine pesticides in soils from a southeastern region of Argentina, Environ. Toxicol. Chem., 2003, 22, 712–717 CrossRef CAS PubMed.
- A. S. Veyhe, D. Hofoss, S. Hansen, Y. Thomassen, T. M. Sandanger, J. O. Odland and E. Nieboer, The Northern Norway Mother-and-Child Contaminant Cohort (MISA) Study: PCA analyses of environmental contaminants in maternal sera and dietary intake in early pregnancy, Int. J. Hyg. Environ. Health, 2015, 218, 254–264 CrossRef CAS PubMed.
-
ATSDR, Toxicological profile for alpha-, beta-, gamma-, and delta-hexachlorocyclohexane, Agency for Toxic Substances and Disease Registry, Atlanta, GA, 2005, pp. 1–325, https://www.atsdr.cdc.gov/toxprofiles/tp43.pdf Search PubMed.
- K. R. Channa, H. B. Rollin, K. S. Wilson, T. H. Nost, J. O. Odland, I. Naik and T. M. Sandanger, Regional variation in pesticide concentrations in plasma of delivering women residing in rural Indian Ocean coastal regions of South Africa, J. Environ. Monit., 2012, 14, 2952–2960 RSC.
- B. Wang, D. Yi, L. Jin, Z. Li, J. Liu, Y. Zhang, X. Qiu, W. Liu, S. Tao and A. Ren, Organochlorine pesticide levels in maternal serum and risk of neural tube defects in offspring in Shanxi Province, China: a case-control study, Sci. Total Environ., 2014, 490, 1037–1043 CrossRef CAS PubMed.
- H. B. Rollin, T. M. Sandanger, L. Hansen, K. Channa and J. O. Odland, Concentration of selected persistent organic pollutants in blood from delivering women in South Africa, Sci. Total Environ., 2009, 408, 146–152 CrossRef CAS PubMed.
- R. Kallenborn, K. Breivik, S. Eckhardt, C. R. Lunder, S. Mano, M. Schlabach and A. Stohl, Long-term monitoring of persistent organic pollutants (POPs) at the Norwegian Troll station in Dronning Maud Land, Antarctica, Atmos. Chem. Phys., 2013, 13, 6983–6992 Search PubMed.
- M. Long, A. K. Knudsen, H. S. Pedersen and E. C. Bonefeld-Jorgensen, Food intake and serum persistent organic pollutants in the Greenlandic pregnant women: The ACCEPT sub-study, Sci. Total Environ., 2015, 529, 198–212 CrossRef CAS PubMed.
- M. S. Curren, C. L. Liang, K. Davis, K. Kandola, J. Brewster, M. Potyrala and H. M. Chan, Assessing determinants of maternal blood concentrations for persistent organic pollutants and metals in the eastern and western Canadian Arctic, Sci. Total Environ., 2015, 527–528, 150–158 CrossRef CAS PubMed.
- M. S. Curren, K. Davis, C. L. Liang, B. Adlard, W. G. Foster, S. G. Donaldson, K. Kandola, J. Brewster, M. Potyrala and J. Van Oostdam, Comparing plasma concentrations of persistent organic pollutants and metals in primiparous women from northern and southern Canada, Sci. Total Environ., 2014, 479–480, 306–318 CrossRef CAS PubMed.
- S. Hansen, E. Nieboer, J. O. Odland, T. Wilsgaard, A. S. Veyhe and T. M. Sandanger, Levels of organochlorines and lipids across pregnancy, delivery and postpartum periods in women from Northern Norway, J. Environ. Monit., 2010, 12, 2128–2137 RSC.
- Q. Bu, M. MacLeod, F. Wong, L. M. Toms, J. F. Mueller and G. Yu, Historical intake and elimination of polychlorinated biphenyls and organochlorine pesticides by the Australian population reconstructed from biomonitoring data, Environ. Int., 2015, 74, 82–88 CrossRef CAS PubMed.
- M. Gasull, M. Bosch de Basea, E. Puigdomenech, J. Pumarega and M. Porta, Empirical analyses of the influence of diet on human concentrations of persistent organic pollutants: a systematic review of all studies conducted in Spain, Environ. Int., 2011, 37, 1226–1235 CrossRef CAS PubMed.
- M. Munoz-de-Toro, H. R. Beldomenico, S. R. Garcia, C. Stoker, J. J. De Jesus, P. M. Beldomenico, J. G. Ramos and E. H. Luque, Organochlorine levels in adipose tissue of women from a littoral region of Argentina, Environ. Res., 2006, 102, 107–112 CrossRef CAS PubMed.
Footnotes |
† Electronic supplementary information (ESI) available. See DOI: 10.1039/c7em00278e |
‡ Full names of the abbreviations used are provided in Section 2.2. |
|
This journal is © The Royal Society of Chemistry 2017 |