DOI:
10.1039/C7DT02255G
(Communication)
Dalton Trans., 2017,
46, 11529-11532
Phototoxicity of strained Ru(II) complexes: is it the metal complex or the dissociating ligand?†
Received
21st June 2017
, Accepted 12th July 2017
First published on 13th July 2017
Abstract
A photochemically dissociating ligand in Ru(bpy)2(dmphen)Cl2 [bpy = 2,2′-bipyridine; dmphen = 2,9-dimethyl-1,10-phenanthroline] was found to be more cytotoxic on the ML-2 Acute Myeloid Leukemia cell line than Ru(bpy)2(H2O)22+ and prototypical cisplatin. Our findings illustrate the potential potency of diimine ligands in photoactivatable Ru(II) complexes.
Cancer is a disease associated with high mortality rates in the entire world.1 Limited numbers of metal-based chemotherapeutic agents are clinically approved, among which is the prototypical drug cisplatin.2 This Pt(II) complex has been associated with side-effects and drug-resistance problems among patients.3,4 On the one hand, photoactivated chemotherapy (PACT) drugs5 rely on the selective, localized and stoichiometric release of cytotoxic substances following controlled light irradiation.6,7 Using this technique, tumors can be targeted with fewer side effects than conventional chemotherapy since prodrugs are ideally inert in the dark at the concentration used.5,8 When transition metal complexes are deployed, photochemical reactivity can be modulated by virtue of structural modifications.9 Photophysical tuning of the excited state can be achieved via fine design of organic ligand frameworks.10 On the other hand, photodynamic therapy (PDT) drugs act through the catalytic production of 1O2 and subsequently reactive oxygen species (ROS) that will induce cell death.11 More recently, research on the photochemical release of cytotoxic agents through ligand dissociation of PACT has been increasing.12–15 A significant tuning of photochemical reactivity was afforded by ligand functionalization around metal centers.9 In sterically congested Ru(II) polypyridyl complexes bearing alkyl or phenyl substituents on the 2,9 positions of 1,10-phenanthroline or 6,6′ positions of 2,2′-bipyridine, photochemical ligand ejection follows the population of a dissociative triplet metal-centered excited state (3*MC).16–18 The latter can be thermally populated from the triplet metal-to-ligand charge transfer state (3*MLCT).19 As the distortions from the regular octahedral geometry increased, the formation of the 3*MC state is increasingly favored.16–18 In recent studies, attention has been paid to the role of the Ru(bpy)2(H2O)22+ product formed as a result of photochemical dissociation of sterically strained diimine ligands.13 This photoproduct is believed to mimic cisplatin's mode of action by cross-linking DNA.20 However, along with the release of these species, side-products consisting of free ligands are also formed. Recently, the 2,9-dimethyl-1,10-phenanthroline (dmphen) ligand was used in examples of chiral Ru(II) complexes containing a 2,3-dihydro-1,4-dioxino[2,3-f]-1,10-phenanthroline (dop)15 ligand or hydroxyquinoline ligand.21 In addition, structurally related compounds to dmphen were used in conjunction with bipyridine such as dmdop (2,3-dihydro-1,4-dioxino[2,3-f]-2,9-dimethyl-1,10-phenanthroline)15 or dpq (dipyrido[3,2-f:2′,3′-h]-quinoxaline).13 All these examples furnished Ru(II) complexes that demonstrated potential application as PACT drugs in cancer therapy. In general, the biological role of dissociated ligands is ill-characterized and mostly assumed to be inert. While this assumption is correct for a variety of examples tested on a number of cell lines, we found that the free dmphen is significantly more toxic than the Ru(bpy)2(H2O)22+ co-product on the ML-2 cell line (Acute Myeloid Leukemia [AML], non-adherent). Despite the fact that the utilization of PACT which produce multiple potentially potent species might complicate the mechanistic studies in biological media, we believe it has a major impact on future photosensitizer design and application. In this perspective, the metal center can be merely used as a carrier for highly cytotoxic ligands. The latter would be selectively released inside or in the vicinity of cancer cells upon light activation. Notably, the photochemistry of caged Ru compounds has been utilized for the release of biologically active molecules such as serotonin,22 CO,23 NO,24etc.
Ru(bpy)2(dmphen)(PF6)2 and Ru(bpy)2(dmphen)Cl2 were synthesized and characterized using a variety of techniques, see the ESI for 1H and 13C NMR, elemental analysis, UV-vis, HPLC, and ESI-MS.†
25 The quantum yield of ligands by photoejection from Ru(bpy)2(dmphen)(PF6)2 was measured following 442 nm He/Cd laser excitation (Fig. S4†). A value of 0.32 ± 0.03% was obtained in acetonitrile which is comparable to previously reported sterically strained Ru(m-bpy)3(BF4)2 [m-bpy = 6-methyl-2,2′-bipyridine] and one order of magnitude larger than Ru(bpy)3(PF6)2.26 Note that these quantum yield values are acquired for complexes bearing non-coordinating counter-ions in acetonitrile as a coordinating solvent. NMR spectroscopy in CD3CN revealed that either bpy or dmphen ligands can dissociate from Ru(bpy)2(dmphen)(PF6)2 forming a mixture of solvent-bound Ru(II) products27 and free ligands in ∼1
:
1 (bpy
:
dmphen) ratio (Fig. S5†).
The photochemical ligand dissociation of water-soluble Ru(bpy)2(dmphen)Cl2 was studied in acetonitrile and water under versatile broadband irradiation (white LED light, see the ESI† for details). Under these experimental conditions, the half-life in acetonitrile was found to be 5 times less in water (∼5 min) than in acetonitrile (∼25 min) (Fig. 1). The rate of ligand release was slower in water than in acetonitrile which is attributed to a larger solubility of the ejected ligand in the latter as well as the preference of the Ru(II) center to acetonitrile over aquo ligands. It is worth noting that Ru(bpy)2(dmphen)Cl2 was stable in the dark as evidenced by the lack of change in the UV-vis spectra after a week of storage in aqueous solution.
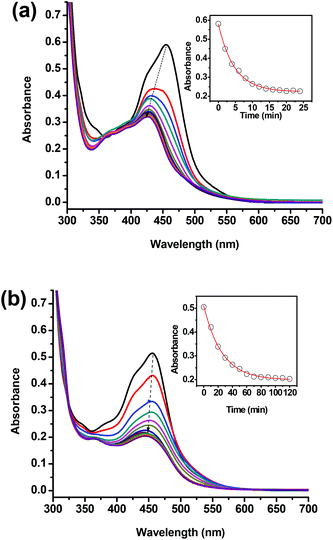 |
| Fig. 1 Absorption spectra of a stirred solution of Ru(bpy)2(dmphen)Cl2 (30 μM) irradiated with white LED light (see the ESI† for more details) placed 2 cm away from a 1 cm pathlength quartz cuvette in (a) acetonitrile recorded at 2 min intervals and (b) water at 10 min intervals. Dashed arrows indicate the progress of the charge transfer absorption peak. The inset represents the change of absorbance at 450 nm as a function of irradiation time. | |
Previously reported PACT agents bearing substituted bipyridyl ligands such as Ru(bpy)2(dmbpy)Cl2 [dmbpy = 6,6′-dimethyl-2,2′-bipyridine] displayed a quantitative photoejection of the dmbpy ligand.13 However, it is not uncommon to have a non-substituted ligand, such as bipyridine, dissociate from a sterically encumbered ruthenium complex. Sauvage and coworkers have previously reported the photochemical dissociation of bipyridine from Ru(bpy)2(dpph)(PF6)2 [dppH = 2,9-diphenyl-1,10-phenanthroline].27 Since ligand release likely occurs through a stepwise one nitrogen dissociation of the diimine moiety,28 the re-coordination of the rigid phenanthroline based moiety is more efficient than the flexible bipyridine ligands leading to competitive photo-induced ligand dissociation of dmphen and bpy ligands from Ru(bpy)2(dmphen)Cl2. In addition, steric interactions around the metal can be relieved via an asymmetrical distortion of the octahedral geometry and Ru–N bond elongation labilizing both dmphen and bpy ligands.29 The cytotoxicities of Ru(bpy)2(dmphen)Cl2, Ru(bpy)2Cl2, dmphen, bpy, an equimolar mixture of Ru(bpy)2Cl2 and dmphen, and cisplatin were measured on the ML-2 cancer cell line (Fig. 2 and Table 1).
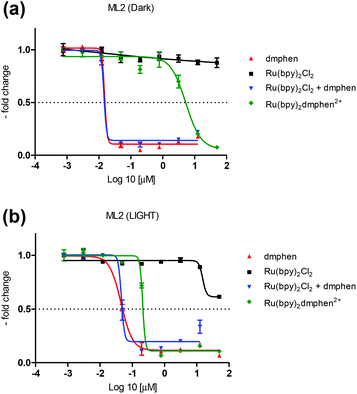 |
| Fig. 2 Cytotoxicity data acquired on the ML2 cell line of Ru(bpy)2dmphenCl2, Rubpy2Cl2, 2,9-dimethyl-1,10-phenanthroline (dmphen), and a mixture of Rubpy2Cl2 and dmphen in the dark (a) and upon blue-light (see the ESI† for more details) excitation (b). | |
Table 1 IC50 on ML2 cell lines expressed in μmol L−1 of Ru(bpy)2dmphenCl2, Rubpy2Cl2, 2,9-dimethyl-1,10-phenanthroline (dmphen), 2,2′-bipyridine (bpy), and a mixture of Rubpy2Cl2 and dmphen, in the dark and upon light activation. Cisplatin and bpy controls (Fig. S6) were acquired in the dark
Compound |
Dark |
Light |
Ru(bpy)2dmphenCl2 |
5.5 μM |
0.2 μM |
Rubpy2Cl2 |
>100 μM |
>100 μM |
dmphen |
0.02 μM |
0.04 μM |
bpy |
>100 μM |
|
Rubpy2Cl2 + dmphen |
0.02 μM |
0.04 μM |
Cisplatin |
4.0 μM |
|
The photoresponsive Ru(bpy)2(dmphen)Cl2 was tested in the dark and upon photoactivation revealing a phototoxicity index (PI = [IC50 dark]/[IC50 light]) of 27.5, with IC50 of 5.5 μM in the dark and of 0.2 μM under light (Fig. 2 and Table 1). A blue LED light source (see the ESI† for details) was used to provide metal-to-ligand charge transfer (MLCT) photoexcitation. The IC50 of the dmphen ligand was found to be ∼0.02–0.04 μM (difference between light and dark is not significant due to plate to plate variability) with and without the presence of Ru(bpy)2Cl2 indicating that the former is significantly more potent than the latter. Notably, this ligand was also more potent than the prototypical cisplatin complex which possessed an IC50 of 4.0 μM when measured under dark conditions, Fig. S6.† It is worth noting that the bpy ligand was found to have no potency at concentrations lower than 50 μM on ML-2 cells with IC50 > 100 μM, Fig. S6.† In previous studies, bpy exhibited moderate cytotoxicity (IC50 ∼ 30 μM) on the chronic myelogenous leukemia cell line (K562) whereas no potency was detected on MDA-MB-231 and MCF-7 cells.30,31 To further substantiate our findings, Ru(bpy)2Cl2, a thermal and photochemical precursor to Ru(bpy)2(H2O)22+ (Fig. S8†),20,32 exhibited marginal cytotoxicity with and without irradiation (IC50 > 100 μM in the dark and upon light activation, Fig. 2 and Table 1). These results are consistent with a previous cytotoxicity assessment on L1210 and HeLa cells whereby Ru(bpy)2(H2O)22+ was shown to lack DNA interstrand cross-linking efficiency.33 Furthermore, Ru(bpy)2(H2O)22+ was not found to be a potent cysteine protease enzyme inhibitor in a study on isolated enzymes and human cell lysates.34 The photoproducts of Ru(bpy)2(dmphen)Cl2 were assessed in water using ESI-MS experiments under identical irradiation conditions used in biological studies. In aqueous medium, it was found that both bpy and dmphen dissociate in a ratio of ∼3
:
2 (bpy
:
dmphen) to form the corresponding polypyridyl Ru(II) aquo species, Fig. S7.† In addition, the ESI-MS (Fig. S7 and S8†) results were supportive of the photochemical formation of Ru(bpy)2(H2O)22+ from Ru(bpy)2Cl2 and Ru(bpy)2(dmphen)Cl2 in water. Based on these data combined, the significant phototoxicity of Ru(bpy)2(dmphen)Cl2 on the ML-2 cancer cell line can be largely attributed to the released dmphen ligand rather than the formation of Ru(bpy)2(H2O)22+ which was found to be minimally potent under our experimental conditions. Peculiarly, dmphen was also previously found to be potent on the L1210 cell line with IC50 ∼ 0.25 μM.35 The mechanistic function of metal binding chelators, such as dmphen, was proposed to be through two plausible pathways: binding to free essential metals and to trace-metal contaminants in cell culture media.35 Both mechanisms lead to the formation of biologically active metal-chelate complexes.35 Despite the fact that our data clearly show the potential role of the dmphen ligand in dictating the photobiological activity of the Ru(bpy)2dmphenCl2 complex, we cannot rule out the contribution of other possible photoproducts such as Ru(bpy)(dmphen)(H2O)2+ which was detected by ESI-MS, Fig. S7.† In addition, besides water, there are multiple potential ligands in biological media leading to the formation of multiple species that could bind DNA or target specific organelles within the cell.20
Conclusions
Ru(bpy)2(dmphen)Cl2, a sterically congested and photochemically labile Ru(II) complex, was investigated against the ML-2 Acute Myeloid Leukemia (AML) cancer cell line. Upon visible light irradiation in water, either bpy or dmphen ligands dissociate to form polypyridyl Ru(II) aquo species. Ligand ejection was more rapid in acetonitrile than in water likely due to the better solvation of photoproducts in the former solvent. Ru(bpy)2Cl2, a thermal and photochemical precursor to Ru(bpy)2(H2O)22+,32 was found to be minimally potent relative to highly cytotoxic dmphen when tested independently on the ML-2 cancer cell line in the dark and upon photoactivation. These experiments clearly indicate that the ruthenium center can act as a carrier to a cytotoxic diimine ligand. In addition, these findings unveil the potential role of dissociating ligands in the biological mechanism of action in strained polypyridyl Ru(II) produgs. Finally, this work may aid the development and understanding of new caged PACT drugs containing cytotoxic phenanthroline or bipyridine derivatives.
Acknowledgements
RSK acknowledges financial support from the School Research and Development Council at the Lebanese American University and the Lebanese National Council for Scientific Research (Ref: 05-06-14).
References
- L. A. Torre, F. Bray, R. L. Siegel, J. Ferlay, J. Lortet-Tieulent and A. Jemal, CA-Cancer J. Clin., 2015, 65, 87–108 CrossRef PubMed.
- A. S. Abu-Surrah and M. Kettunen, Curr. Med. Chem., 2006, 13, 1337–1357 CrossRef CAS PubMed.
- V. Cepeda, M. A. Fuertes, J. Castilla, C. Alonso, C. Quevedo and J. M. Perez, Anti-Cancer Agents Med. Chem., 2007, 3–18 CrossRef CAS.
- M. A. Fuertes, C. Alonso and J. M. Perez, Chem. Rev., 2003, 103, 645–662 CrossRef CAS PubMed.
- N. J. Farrer, L. Salassa and P. J. Sadler, Dalton Trans., 2009, 10690–10701 RSC.
- R. Ackroyd, C. Kelty, N. Brown and M. Reed, Photochem. Photobiol., 2001, 74, 656–669 CrossRef CAS PubMed.
- M. R. Detty, S. L. Gibson and S. J. Wagner, J. Med. Chem., 2004, 47, 3897–3915 CrossRef CAS PubMed.
- D. E. J. G. J. Dolmans, D. Fukumura and R. K. Jain, Nat. Rev. Cancer, 2003, 3, 380 CrossRef CAS PubMed.
- C. Mari, V. Pierroz, S. Ferrari and G. Gasser, Chem. Sci., 2015, 6, 2660–2686 RSC.
- N. A. Smith and P. J. Sadler, Philos. Trans. R. Soc., A, 2013, 371, 20120519 CrossRef PubMed.
- M. C. DeRosa and R. J. Crutchley, Coord. Chem. Rev., 2002, 233–234, 351–371 CrossRef CAS.
- R. N. Garner, J. C. Gallucci, K. R. Dunbar and C. Turro, Inorg. Chem., 2011, 50, 9213–9215 CrossRef CAS PubMed.
- B. S. Howerton, D. K. Heidary and E. C. Glazer, J. Am. Chem. Soc., 2012, 134, 8324–8327 CrossRef CAS PubMed.
- J. D. Knoll, B. A. Albani and C. Turro, Acc. Chem. Res., 2015, 48, 2280–2287 CrossRef CAS PubMed.
- A. N. Hidayatullah, E. Wachter, D. K. Heidary, S. Parkin and E. C. Glazer, Inorg. Chem., 2014, 53, 10030–10032 CrossRef CAS PubMed.
- J.-P. Collin, D. Jouvenot, M. Koizumi and J.-P. Sauvage, Inorg. Chem., 2005, 44, 4693–4698 CrossRef CAS PubMed.
- P. Mobian, J.-M. Kern and J.-P. Sauvage, Angew. Chem., Int. Ed., 2004, 43, 2392–2395 CrossRef CAS PubMed.
-
V. W. Yam, E. Baranoff, F. Barigelletti, S. Bonnet, J.-P. Collin, L. Flamigni, P. Mobian and J.-P. Sauvage, in Photofunctional Transition Metal Complexes, Springer, Berlin, Heidelberg, 2007, vol. 123, pp. 41–78 Search PubMed.
- J. V. Caspar and T. J. Meyer, J. Am. Chem. Soc., 1983, 105, 5583–5590 CrossRef CAS.
- T. N. Singh and C. Turro, Inorg. Chem., 2004, 43, 7260–7262 CrossRef CAS PubMed.
- D. K. Heidary, B. S. Howerton and E. C. Glazer, J. Med. Chem., 2014, 57, 8936–8946 CrossRef CAS PubMed.
- L. Zayat, M. Salierno and R. Etchenique, Inorg. Chem., 2006, 45, 1728–1731 CrossRef CAS PubMed.
- M. A. Wright and J. A. Wright, Dalton Trans., 2016, 45, 6801–6811 RSC.
- P. C. Ford, Acc. Chem. Res., 2008, 41, 190–200 CrossRef CAS PubMed.
- E. V. Dose and L. J. Wilson, Inorg. Chem., 1978, 17, 2660–2666 CrossRef CAS.
- Q. Sun, S. Mosquera-Vazquez, L. M. Lawson Daku, L. Guénée, H. A. Goodwin, E. Vauthey and A. Hauser, J. Am. Chem. Soc., 2013, 135, 13660–13663 CrossRef CAS PubMed.
- A.-C. Laemmel, J.-P. Collin and J.-P. Sauvage, Eur. J. Inorg. Chem., 1999, 1999, 383–386 CrossRef.
- S. Tachiyashiki, H. Ikezawa and K. Mizumachi, Inorg. Chem., 1994, 33, 623–625 CrossRef CAS.
- H. Ichida, S. Tachiyashiki and Y. Sasaki, Chem. Lett., 1989, 18, 1579–1580 CrossRef.
- D. A. Paixão, I. M. Marzano, E. H. L. Jaimes, M. Pivatto, D. L. Campos, F. R. Pavan, V. M. Deflon, P. I. da S. Maia, A. M. Da Costa Ferreira, I. A. Uehara, M. J. B. Silva, F. V. Botelho, E. C. Pereira-Maia, S. Guilardi and W. Guerra, J. Inorg. Biochem., 2017, 172, 138–146 CrossRef PubMed.
- P. P. Silva, W. Guerra, G. C. dos Santos, N. G. Fernandes, J. N. Silveira, A. M. da Costa Ferreira, T. Bortolotto, H. Terenzi, A. J. Bortoluzzi, A. Neves and E. C. Pereira-Maia, J. Inorg. Biochem., 2014, 132, 67–76 CrossRef CAS PubMed.
- A. Vaidyalingam and P. K. Dutta, Anal. Chem., 2000, 72, 5219–5224 CrossRef CAS PubMed.
- O. Novakova, J. Kasparkova, O. Vrana, P. M. van Vliet, J. Reedijk and V. Brabec, Biochemistry, 1995, 34, 12369–12378 CrossRef CAS PubMed.
- T. Respondek, R. N. Garner, M. K. Herroon, I. Podgorski, C. Turro and J. J. Kodanko, J. Am. Chem. Soc., 2011, 133, 17164–17167 CrossRef CAS PubMed.
- A. Mohindru, J. M. Fisher and M. Rabinovitz, Nature, 1983, 303, 64–65 CrossRef CAS PubMed.
Footnote |
† Electronic supplementary information (ESI) available: Experimental section, NMR spectra, HPLC chromatogram, ESI-MS, UV-vis spectra and cytotoxicity data. See DOI: 10.1039/c7dt02255g |
|
This journal is © The Royal Society of Chemistry 2017 |