DOI:
10.1039/C7DT02107K
(Paper)
Dalton Trans., 2017,
46, 10847-10858
Synthesis and evaluation of biological properties of ferrocenyl–podophyllotoxin conjugates†
Received
9th June 2017
, Accepted 13th July 2017
First published on 14th July 2017
Abstract
Three types, esters, amides and 1,2,3-triazoles, of ferrocenyl–podophyllotoxin conjugates were synthesised, and their anticancer activity was evaluated. We observed that the most potent ferrocenyl derivatives were esters. Esters 15, 16 and 17 acted in a similar way to podophyllotoxin, i.e. reduced the number of G1 phase cells and induced G2/M blockage, while esters 14 and 18 and amide 19 blocked cells in S phase in a similar manner to etoposide.
Introduction
Native Americans used mandrake (Podophyllum peltatum) as an emetic, cathartic and anthelmintic herb. It was also used to treat warts and other proliferative skin conditions. In 1946, King and Sullivan demonstrated that an alcoholic extract of mandrake roots and rhizomes (called podophyllin) has colchicine-like properties.1 The active substance, podophyllotoxin (PPT) (Fig. 1), was further shown to compete for the colchicine-binding site of microtubules2 and thus lead to G2/M blockage of the cell cycle. But the high systemic toxicity of PPT prevents the using of this compound in cancer medicine. Therefore, a series of its semisynthetic derivatives, including etoposide and teniposide (Fig. 1), were developed, and these are currently used as chemotherapeutics. The mode of action of the aforementioned compounds differs from that of PPT – they are known to induce single- and double-strand DNA breaks leading to the accumulation of cells in S and G2 phases.3 The cellular target of etoposide and teniposide is topoisomerase II4 – an enzyme responsible for the relief of torsional stress that occurs during DNA transcription and replication.
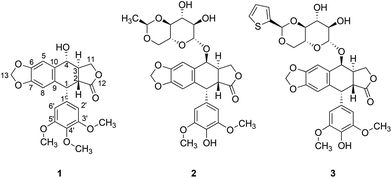 |
| Fig. 1 Structures of podophyllotoxin 1, etoposide 2, and teniposide 3. | |
The success of podophyllotoxin-based anticancer drugs in chemotherapy encouraged scientists to search for new derivatives of PPT as anticancer drug candidates. Its structure–activity relationship (SAR) indicated that lactone and benzo[d]1,3-dioxolane moieties play a crucial role in the anticancer activity of PPT. Therefore, most of the current modifications of the PPT structure focus on 4-hydroxy and 4′-methoxy moieties.5 Simple esterification of a 4-hydroxy moiety with acrylic acids6 leads to potent anti-multidrug resistance (MDR) agents. Other ester conjugates of podophyllotoxin exhibit promising anticancer properties, e.g. conjugates with norcantharidin,6a 5-fluorouracil,7 and alkanoic acids.8 Some amide derivatives of PPT, e.g. the spermidine derivative,9,10 are currently under testing in phase 1 clinical trials. 1,2,3-Triazolyl11 and 1,2,3,4-tetrazolyl12 linkers have also been successfully used in the construction of promising new podophyllotoxin-based active anti-cancer compounds.
The success of cisplatin in the treatment of cancers initiated an intensive search for new metal-based compounds for use as potential antibiotic or cytostatic agents.13 To date, many chemically diverse ferrocenyl compounds with promising anti-cancer,14 anti-bacterial15 and anti-malarial16 activities have been synthesised. Very recently, Jaouen et al. described the synthesis of O-ferrocenoylpodophyllotoxin; however, they only studied the cytotoxic properties of this compound towards two breast cancer cell lines.17
Ferrocenyl derivatives and analogues of antimitotic drugs are able to show novel and unexpected properties.14c,18 We have demonstrated that a ferrocenyl analogue of paclitaxel is more potent in terms of its antiproliferative activity than the mother compound.18 In addition, a ferrocenyl analogue of another mitotic spindle toxin (plinabulin) is not only highly active in multidrug resistant cells but directly inhibits MDR proteins.14c The latter results encouraged us to study the effect of the ferrocenyl moiety on the antiproliferative activity of podophyllotoxin. Herein we describe the synthesis and study of the cytostatic and cytotoxic properties of a series of ferrocenyl derivatives of podophyllotoxin (Fig. 2) towards a set of human cell lines, including multidrug resistant cells obtained via a stepwise selection with widely used chemotherapeutics. In addition, we also studied the effects of the metallocene moiety on cell cycle progression and tubulin polymerization.
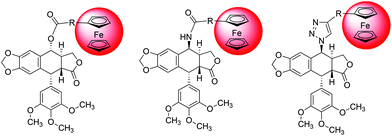 |
| Fig. 2 General structures of ester (left), amide (middle), and 1,2,3-triazole (right) ferrocenyl–podophyllotoxin conjugates. | |
Results and discussion
Synthesis
In order to investigate the influence of the ferrocenyl moiety on the cytotoxic properties of 1, three types of ferrocene–podophyllotoxin conjugates were synthesised starting from 1, azido 4 and amino 5 derivatives of podophyllotoxin (Fig. 2). First, the reaction of 1 with sodium azide in trifluoroacetic acid at RT led to 4-azido-4-deoxy-4-epipodophyllotoxin 4 in 90% yield.19 Further reduction of the azido group by hydrogen in the presence of 10% of palladium on carbon in ethyl acetate at RT gave the corresponding 4-amino-4-deoxy-4-epipodophyllotoxin 5 in 85% yield (Scheme 1).20
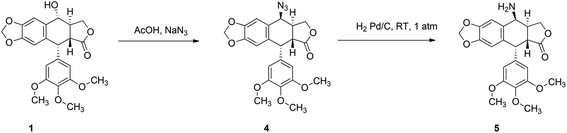 |
| Scheme 1 Synthesis of 4-azido- 4 and 4-amino-4-deoxy-4-epipodophyllotoxin 5. | |
Ferrocenylalkanoic acids required for the synthesis of ester and amide conjugates were synthesised according to Scheme 2. 3-Ferrocenoylpropionic acid 721 and 4-ferrocenoylbutyric acid 822 were prepared in a Friedel–Crafts acylation of ferrocene with succinic and glutaric anhydride, respectively, while 5-ferrocenoylpentanoic acid 10 was synthesised in two steps. First, ferrocene reacts with mono-methyl adipate and trifluoroacetic anhydride in the presence of triflic acid to give methyl 5-ferrocenoylpentanoate 9. Hydrolysis of the ester 9 with potassium hydroxide in aqueous solution led to the corresponding ketoacid 10. Finally, reduction of the ketone group in 7, 8 and 10 in a reaction with zinc amalgamate and hydrochloric acid in a toluene solution led to ω-ferrocenylalkanoic acids 11,231222 and 13,18b respectively.
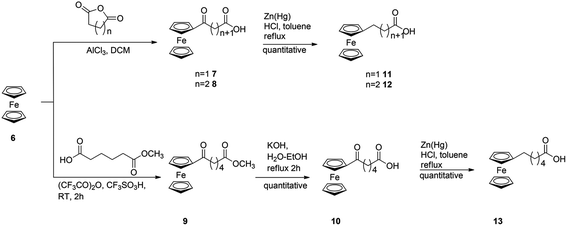 |
| Scheme 2 Synthesis of ϖ-ferrocenylalkanoic acids. | |
O-Acylation of 1 with ϖ-ferrocenylalkanoic acids and N,N′-diizopropylcarbodiimide (DIC) and 4-dimethylaminopyridine (DMAP) carried out in dichloromethane at RT for 24 h gave the corresponding esters 14–18 in 80–85% yields (Scheme 3).
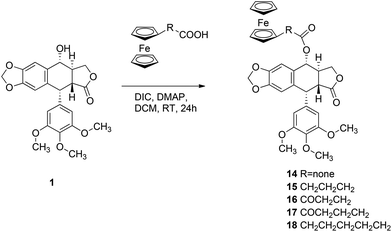 |
| Scheme 3 Synthesis of podophyllotoxin esters 14–18. | |
Amide conjugates of podophyllotoxin were prepared in good yield in a reaction of 5 with ferrocenecarboxylic acid, 3-ferrocenoylpropionic acid 7 and 4-ferrocenylbutyric acid 11 using BOP and DIPEA as coupling reagents. Reactions were carried out at RT in acetonitrile for 20 min and the corresponding amides 19–21 were isolated in 70%, 52%, and 72% yields, respectively (Scheme 4).
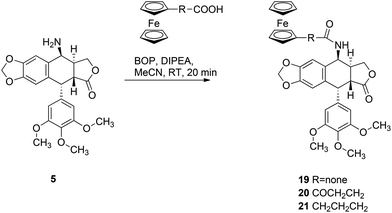 |
| Scheme 4 Synthesis of podophyllotoxin amides 19–21. | |
In order to synthesise 1,2,3-triazole conjugates of podophyllotoxin, first we prepared ϖ-alkynoylferrocenes 26–28via the Friedel–Crafts acylation of ferrocene with ϖ-alkynoic acids 22–24 in the presence of trifluoroacetic anhydride and triflic acid (Scheme 5).24 Then, ethynylferrocene or 26–28 reacts with azide 4 in the presence of TTTA, copper(II) sulphate and sodium ascorbate in a mixture of methanol and water to give the corresponding 1,2,3-triazoles 29–32 in 86%, 75%, 32%, and 17% yields, respectively (Scheme 6).
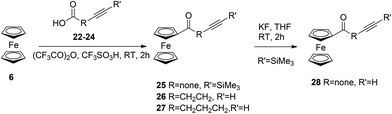 |
| Scheme 5 Synthesis of ϖ-alkynoylferrocenes 26–28. | |
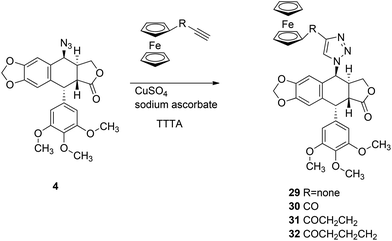 |
| Scheme 6 Synthesis of 1,2,3-triazoles 29–32. | |
The structure of compound 29 was confirmed by X-ray analysis. Crystals suitable for X-ray analysis were obtained by slow diffusion of n-pentane into a dichloromethane solution of 29. Compound 29 crystallised in the chiral P1 space group in a triclinic system. The cell unit comprises two independent molecules of compound 29 and two molecules of the solvent – dichloromethane. The crystal structure is chirally pure, with the following absolute configurations at the appropriate asymmetric carbon atoms: C13 (S), C14 (S), C17 (R), C18 (R), and analogously C53 (S), C54 (S), C57 (R), and C58 (R). The ORTEP representations of both molecules, with appropriate numbering, are presented in Fig. 3. As can be seen, X-ray analysis confirmed the inversion of the configuration of C-4 (here denoted as C13 and C53 in molecules A and B, accordingly) with respect to 1.
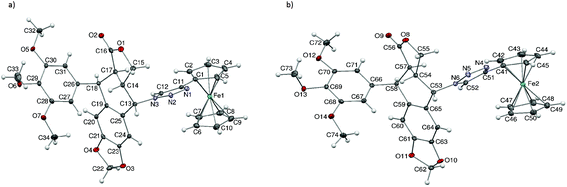 |
| Fig. 3 ORTEP representations of molecules A (a) and B (b) of 29. Atomic displacement parameters are presented at the 50% probability level. Hydrogen atom labels are omitted for clarity. Atomic numbers for molecule B are those from molecule A + 40. Most relevant bond lengths in Å and angles in °: Molecule A, C1–C11 1.463(3), C11–C12 1.375(2), C12–N3 1.354(2), N3–C13 1.475(2), C25–C13 1.520(3), average C–C distance in Fc: 1.424(6), average Fe–C distance in Fc: 2.044(4); C2–C1–C11–N1 −161.0(2), N2–N3–C13–C14 82.4(2); molecule B, C41–C51 1.460(3), C51–C52 1.382(3), C52–N6 1.351(3), N6–C53 1.478(3), C65–C53 1.519(3), C42–C41–C51–N4 −148.7(2), N5–N6–C53–C54 79.3(2). | |
The two independent molecules of 29 share several structural features. In both molecules, the 1,2,3-triazole moiety is inclined approximately 20–30 degrees with respect to the cyclopentadienyl ring, with the C–H group pointing below the plane of the cyclopentadienyl ring. The planes of the 1,2,3-triazole ring, podophyllotoxin core and 3,4,5-trimethoxyphenyl moiety are almost perpendicular to one another. Structural differences between the two independent molecules are significant even if small, and manifest mostly in the following molecular fragments: (1) the terminal methoxy groups in the two molecules are rotated slightly differently with respect to the benzene ring they are connected to; and (2) the ferrocenyl moieties in the two molecules are slightly differently tilted with respect to the triazole ring; the effect is best visible in Fig. 4 (overlay of the two independent molecules): C2–C1–C11–N1: −160.98(18)°, C42–C41–C51–N4: −148.7(2)°; the ferrocenyl moiety in molecule B represents a conformation close to eclipsed, while in the case of molecule A the two cyclopentadienyl rings are significantly rotated with respect to each other: C41–Ct3–Ct4–C42: −7.1(5)°, C1–Ct1–Ct2–C6: −21.4(5)°; the C22 methylene group in molecule A is almost co-planar with the adjacent aromatic ring; the C22 atom shows a relatively elongated atomic displacement ellipsoid, which suggests possible slight disorder of the methylene, with alternative positions pointing either towards the ferrocenyl moiety or the 3,4,5-trimethoxyphenyl moiety. In contrast, the analogous C62 methylene group in molecule B is decidedly puckered towards the ferrocenyl moiety.
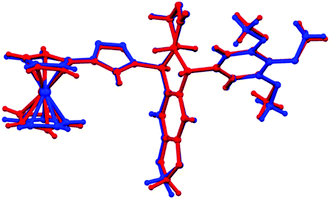 |
| Fig. 4 An overlay of the two molecules from the crystal structure of 29 (RMSD of mol. A and mol. B: 0.2556), molecule A is presented in red, and molecule B in blue. | |
Cytotoxic activity
The cytotoxic activity of the investigated podophyllotoxin analogues was studied in a set of cell lines derived from human tumours of different tissue origin: colorectal adenocarcinomas Colo 205, HCT 116 and SW620, alveolar basal epithelial cell adenocarcinoma A549, hepatocellular carcinoma Hep G2 and breast adenocarcinoma MCF7. Additionally, we employed a panel of five MDR cell lines derived from SW620 which overexpress various ABC proteins, namely ABCG2 (SW620C line), ABCC1 (SW620M and SW620E lines) and ABCB1 (SW620D, SW620E, and SW620 V lines).25
None of the analysed substances were more active than podophyllotoxin (IC50 values of PPT were always below 10 nM; except for Colo 205, where cell survival dropped to 60% at 10 nM and remained constant up to 30 μM, results not shown); however, they were more toxic than etoposide. The most active of the newly synthesised compounds, however, were the esters 16 and 17 with a ferrocenoyl moiety (IC50 in the range between 0.11 and 0.68 μM; Table 1), followed by 15, 18 and 14. Amides 19–21 were poorly toxic, while of the four 1,2,3-triazoles only 31 and 32 exerted any biological effects in the micromolar range. None of the basic cell lines studied were specifically prone to the action of the compounds tested.
Table 1 Cytotoxicity of ferrocenyl–podophyllotoxin conjugates towards a set of different cell lines, as determined by the neutral red uptake assay. 95% confidence intervals are given below (please note that due to the log-transformation of the data required to perform IC50 calculations, these are asymmetrical). IC50 values (expressed in μM) were calculated based on three independent experiments. N.D. denotes a situation in which IC50 values could not be determined (<50% viability was not achieved in the concentration range used)
Compound |
Colo 205 |
HCT 116 |
SW620 |
A549 |
Hep G2 |
MCF7 |
1
|
N.D. |
<0.001 |
0.002 |
0.005 |
0.002 |
<0.001 |
|
— |
0.000–0.008 |
0.003–0.008 |
0.001–0.004 |
— |
2
|
2.6 |
9 |
0.45 |
6 |
1.0 |
0.49 |
0.76–9.1 |
2–41 |
0.33–0.62 |
1–57 |
0.58–1.9 |
0.35–0.68 |
14
|
3.4 |
0.98 |
1.1 |
2.8 |
2.0 |
0.79 |
0.77–15.4 |
0.74–1.3 |
0.96–1.2 |
1.2–6.4 |
1.7–2.3 |
0.63–0.99 |
15
|
1.4 |
0.21 |
0.35 |
0.62 |
0.16 |
0.60 |
0.92–2.2 |
0.15–0.28 |
0.31–0.40 |
0.57–0.68 |
0.14–0.18 |
0.40–0.91 |
16
|
0.21 |
0.11 |
0.27 |
0.12 |
0.56 |
0.53 |
0.16–0.28 |
0.09–0.15 |
0.24–0.30 |
0.11–0.13 |
0.44–0.71 |
0.43–0.66 |
17
|
0.14 |
0.13 |
0.22 |
0.11 |
0.44 |
0.68 |
0.12–0.15 |
0.10–0.16 |
0.19–0.26 |
0.09–0.12 |
0.34–0.58 |
0.56–0.82 |
18
|
0.27 |
0.50 |
1.3 |
0.44 |
6.7 |
N.D. |
0.06–1.2 |
0.40–0.63 |
1.1–1.6 |
0.23–0.65 |
3.9–11.3 |
|
19
|
58 |
N.D. |
32 |
11.4 |
N.D. |
0.08 |
6–588 |
|
3–394 |
8.0–16.1 |
|
0.01–0.63 |
20
|
7.4 |
N.D. |
N.D. |
20.4 |
17.1 |
12.4 |
4.3–12.8 |
|
|
16.0–26.1 |
14.4–20.4 |
9.4–16.3 |
21
|
N.D. |
N.D. |
N.D. |
2.8 |
N.D. |
N.D. |
|
|
|
1.6–4.7 |
|
|
29
|
N.D. |
N.D. |
N.D. |
N.D. |
N.D. |
N.D. |
|
|
|
|
|
|
30
|
N.D. |
N.D. |
N.D. |
9.2 |
N.D. |
N.D. |
|
|
|
2.6–31.8 |
|
|
31
|
6.3 |
5.7 |
5.2 |
5.3 |
6.0 |
5 |
4.6–8.7 |
4.4–7.4 |
2.1–12.6 |
2.9–9.8 |
3.9–9.2 |
1–43 |
32
|
3.8 |
5.8 |
5.9 |
3.4 |
5.7 |
4.0 |
3.1–4.6 |
4.4–7.4 |
4.8–7.2 |
2.1–5.6 |
4.6–7.2 |
3.0–5.3 |
MDR cells seemed not to be significantly more resistant than the parental cell line to the investigated substances (Table 2). The SW620E cell line was originally obtained via a stepwise selection with etoposide and was roughly 9 times more resistant to 2 than the parental cell line (IC50 of 140 μM vs. 16 μM, assayed by MTT-reduction assay).25 In the current experimental setup, the resistance ratio of SW620E towards etoposide is slightly lower (the resistance factor value is approx. 7), but we employed a different viability assay here (neutral red uptake). Both parental and etoposide-resistant cells seem to be comparably sensitive to 14, 17, 18, 31 and 32, while the resistance ratio to 15 and 16 is between 2 and 3. All other MDR cell lines are as susceptible to ferrocenyl–podophyllotoxins conjugates as the parental cell line.
Table 2 Cytotoxicity of ferrocenyl–podophyllotoxin conjugates towards a panel of multidrug resistant cell lines, as determined by the neutral red uptake assay. 95% confidence intervals are given below (please note that due to the log-transformation of the data required to perform IC50 calculations, these are asymmetrical). IC50 values (expressed in μM) were calculated based on three independent experiments. N.D. denotes a situation in which IC50 values could not be determined (<50% viability was not achieved in the concentration range used)
Compound |
SW620 |
SW620C |
SW620D |
SW620E |
SW620M |
SW620V |
1
|
0.002 |
0.005 |
0.003 |
0.009 |
<0.001 |
0.007 |
0.000–0.008 |
— |
0.001–0.007 |
0.009–0.010 |
— |
0.005–0.009 |
2
|
0.45 |
0.38 |
1.5 |
3.1 |
6.0 |
1.7 |
0.33–0.62 |
0.25–0.58 |
1.3–1.8 |
1.9–5.0 |
4.1–8.8 |
0.88–3.3 |
14
|
1.1 |
1.4 |
3.4 |
1.4 |
0.60 |
1.9 |
0.96–1.2 |
1.2–1.8 |
1.8–6.6 |
1.2–1.6 |
0.32–1.1 |
1.5–2.4 |
15
|
0.35 |
0.41 |
0.47 |
0.68 |
0.45 |
0.56 |
0.31–0.40 |
0.25–0.68 |
0.40–0.56 |
0.63–0.72 |
0.34–0.59 |
0.49–0.63 |
16
|
0.27 |
0.42 |
0.64 |
0.84 |
0.51 |
0.69 |
0.24–0.30 |
0.32–0.54 |
0.55–0.75 |
0.72–0.98 |
0.43–0.61 |
0.61–0.79 |
17
|
0.22 |
0.41 |
0.15 |
0.19 |
0.46 |
0.19 |
0.19–0.26 |
0.36–0.48 |
0.12–0.18 |
0.16–0.24 |
0.38–0.57 |
0.16–0.23 |
18
|
1.3 |
1.4 |
0.54 |
1.2 |
0.84 |
1.1 |
1.1–1.6 |
1.1–1.7 |
0.42–0.69 |
0.88–1.7 |
0.54–1.3 |
0.86–1.4 |
19
|
32 |
N.D. |
N.D. |
33 |
N.D. |
N.D. |
3–394 |
|
|
4–296 |
|
|
20
|
N.D. |
N.D. |
N.D. |
N.D. |
N.D. |
N.D. |
|
|
|
|
|
|
21
|
N.D. |
N.D. |
N.D. |
N.D. |
N.D. |
N.D. |
|
|
|
|
|
|
29
|
N.D. |
N.D. |
N.D. |
N.D. |
5.6 |
16 |
|
|
|
|
2.7–11.6 |
1–949 |
30
|
N.D. |
N.D. |
N.D. |
N.D. |
5.2 |
8.2 |
|
|
|
|
3.8–7.1 |
6.5–10.4 |
31
|
5.2 |
8.6 |
4.8 |
9 |
4.6 |
25 |
2.1–12.6 |
7.2–10.1 |
2.6–8.9 |
2–33 |
2.1–10.1 |
2–284 |
32
|
5.9 |
9.5 |
4.1 |
6.1 |
5.3 |
10.9 |
4.8–7.2 |
7.9–11.6 |
2.4–7.0 |
5.3–7.1 |
4.5–6.3 |
7.1–16.7 |
Cell cycle effects
Podophyllotoxin is a potent disruptor of microtubules and thus it prevents cells from completing cell division. Therefore, we studied the effects exerted by ferrocenyl–podophyllotoxins conjugates on SW620 and SW620E cells (Fig. 5). Esters 15, 16 and 17 act in a way similar to podophyllotoxin, reducing the number of G1 phase cells and inducing G2/M blockage. On the other hand, esters 14, 18, and amide 19 tend to block cells in the S phase in a similar manner to etoposide 2 (although this effect is not pronounced). Such an outcome is clearly manifested in parental cells; however, the rate of G2/M blocked cells is also slightly increased in the SW620E line exposed to 15, 16 and 17.
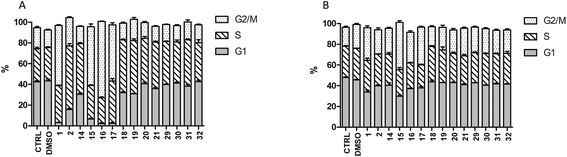 |
| Fig. 5 Cell cycle phase distribution in SW620 (panel A) and SW620E (panel B) cells exposed to 10 nM of a given ferrocenylpodophyllotoxin for 48 h. Average data ± SEM from 3 independent experiments. CTRL – cells incubated in a complete medium alone, DMSO – cells incubated in a complete medium with the addition of 0.1% dimethyl sulfoxide (solvent control). | |
Tubulin polymerization
In order to test whether the observed cell cycle disturbance results from direct interactions between ferrocenyl–podophyllotoxin conjugates and microtubules, we decided to investigate the tubulin polymerization rate. Tubulin subunits spontaneously polymerize at 37 °C and this process can be either augmented by taxol or attenuated by vincristine.26 Therefore, we decided to employ both compounds as controls and studied the ability of newly synthesised organometallic podophyllotoxins to interfere with tubulin polymerization (Fig. 6).
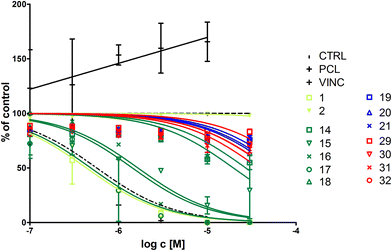 |
| Fig. 6 Effects of ferrocenyl–podophyllotoxin conjugates on the tubulin polymerization rate. CTRL – solvent control, PCL – paclitaxel, VINC – vincristine. Average data ± SEM from 3 independent experiments. | |
We observed that only esters exerted notable inhibitory effects on tubulin polymerization (Table 3). The most active compounds were 15, 16 and 17, the latter one being as efficient as podophyllotoxin. These results are fully compliant with the cell cycle data.
Table 3 IC50 of active ferrocenylpodophyllotoxins towards tubulin polymerization (the mean and 95%-confidence intervals calculated for the data presented in Fig. 6)
Compound |
IC50 |
Vincristine |
0.57 (0.29–1.1) |
1
|
0.43 (0.28–0.67) |
2
|
No effect in the concentration range studied |
14
|
19.7 (10.0–38.8) |
15
|
1.6 (0.70–3.9) |
16
|
1.4 (0.90–2.3) |
17
|
0.49 (0.25–0.94) |
18
|
27.1 (14.5–50.8) |
Stability towards ester bond cleavage exhibited by esterases
Esterases are ubiquitous in the cell. Thus, one could argue that the biological effects observed for ferrocenyl esters of podophyllotoxin actually result from the action of podophyllotoxin which is released after the digestion of the ester bond. To verify this hypothesis, we incubated the esters with crude cell extract obtained by sonication of Hep G2 cells at pH 7.4. Sonication releases esterases from native cellular compartments resulting in the formation of a highly reactive enzymatic mixture. No protease inhibitors were added to avoid non-specific blockage of hydrolytic enzymes. None of the esters used in this study were cleaved to release PPT during a 2 hour incubation at 37 °C (Fig. S1†) while Oregon Green diacetate used as a positive control was completely hydrolysed within minutes. This result strongly suggests that the investigated podophyllotoxin esters are stable under biological conditions. Therefore, it may be implied that the ester form is actually their active form in the cells. Similar results are reported for other PPT esters, e.g. podophyllotoxin acetate, which are themselves believed to exert cytotoxic effects.8b
Conclusions
We synthesised three series of ferrocenyl–podophyllotoxin conjugates: esters, amides and 1,2,3-triazoles. We found that the cytotoxic activity strongly depends on the type of linker between the metallocenyl and podophyllotoxin moieties. Of the compounds tested, only podophyllotoxin esters exerted significant biological effects on a number of cell lines, while amides and triazoles were significantly less cytotoxic. Their mechanism of action was similar to the action of the parental compound, as they efficiently disturbed tubulin polymerization leading to cell cycle inhibition. The synthesised compounds were also active towards multidrug resistant cells, including the etoposide-resistant SW620E cancer cell line, which makes them promising candidates for future research.
Experimental section
All reactions were conducted using standard Schlenk techniques under an argon atmosphere. Chemicals and solvents (HPLC grade) were purchased from Sigma-Aldrich and used as received. Dichloromethane (DCM) was distilled from calcium hydride, acetonitrile (ACN) was distilled from phosphorus pentoxide and stored over activated molecular sieves 4A (8–12 mesh). Melting points were determined on a capillary point DigiMelt MPA161 apparatus equipped with a digital thermometer and are uncorrected. UV-Vis spectra were recorded in a 1,2-dichloroethane solution using a Lambda 45 UV-Vis spectrometer (PerkinElmer) at 294 K. FT-IR spectra were recorded in KBr using a Nexus FT-IR spectrometer (Thermo Nicolet). ESI-MS spectra were recorded in positive mode on a Varian 500-MS LC ion trap spectrometer (samples were dissolved in methanol). Elemental analysis was performed for all new compounds. Unfortunately, we were not able to obtain correct results for all of the new compounds; however, inclusion of additional molecules of water for the calculation gave satisfying results. 1D (1H, 13C{1H} and 13C DEPT 135) and 2D (1H–1H COSY, 1H–13C HSQC or HMQC, 1H–13C HMBC) NMR spectra were recorded on a Bruker ARX 600 MHz (spectrometer frequency 600.3 MHz for 1H and 150.9 MHz for 13C). Chemical shifts of the 1H NMR spectra are referenced relative to residual protons in the deuterated solvent (CDCl3δ = 7.26 ppm for 1H and δ = 77.00 ppm for 13C{1H}; DMSO-d6δ = 2.50 ppm for 1H and δ = 39.51 ppm for 13C{1H}). Spectra were recorded at room temperature (301 K), chemical shifts are in ppm and coupling constants are in Hz. Thin-layer chromatography (TLC) was performed on aluminium sheets precoated with Merck 5735 Kieselgel 60F254. Column chromatography was carried out on FLUKA silica gel 60 for flash chromatography (0.040–0.063 mm, 230–400 mesh). The purity of all the compounds studied in biological assays was higher than 95%, as demonstrated by HPLC. HPLC analyses (for purity tests and the investigation of cellular esterase effects on ferrocenyl esters) were performed with a Shimadzu Prominence system equipped with a PDA detector, using a Phenomenex Kinetex 5μ PFP 100 Å column (150 × 4.6 mm). A gradient was applied using eluents A (0.1% TFA in ACN) and B (0.1% TFA in H2O), starting from A
:
B = 30
:
70 to A
:
B = 90
:
10 within 20 min, then A
:
B = 90
:
10 for 10 min, and A
:
B = 30
:
70 for 10 min. Detection was recorded at wavelengths of 220 nm, 254 nm and 280 nm and a flow rate of 1 mL min−1 was used.
Compounds 4,195,207,218,2211,2312,2213,18b and 25–2824 were prepared according to the literature reported procedures. Compound 928 was prepared according to the same procedure as that described for the synthesis of acetylferrocene27 starting from mono-ethyl adipate. Compound 9 was hydrolysed with potassium hydroxide according to a literature reported procedure28 to give the corresponding acid 10.
General procedure A – synthesis of the esters 14–18
O-Ferrocenoylpodophyllotoxin 14.
To a solution of 100 mg (0.241 mmol) of podophyllotoxin 1, 14.7 mg (0.121 mmol) of DMAP and 83 mg (0.362 mmol) of ferrocenecarboxylic acid in 4 cm3 of anhydrous DCM was added 60.9 mg (75 μl, 0.483 mmol) of DIC and the resulting solution was stirred at RT for 24 h. Then, the solvent was evaporated to dryness and the pure product was obtained by column chromatography on silica (60 cm3) using DCM/EtOAc (5/1) as an eluent. The pure product was obtained as orange crystals in 85% yield (129.6 mg). Mp 132.0–135.0 °C (dec.); elemental analysis found: C, 63.0; H, 5.1. C33H30FeO9 requires C, 63.3; H, 4.8%; λmax (ClCH2CH2Cl)/nm 448, 350 and 313 (ε/dm3 mol−1 cm−1 318, 690 and 1436); IR (KBr) νmax/cm−1: 2933m, 2837m, 1780vs, 1709vs, 1588s, 1506vs, 1484vs, 1457vs, 1420s, 1376m, 1331m, 1267vs, 1239vs, 1171m, 1127vs, 1037s, 1001s, 932s, 870m, 826m, 775m, 485m; ESI-MS calculated for C33H30FeO9m/z = 626.1; found m/z 626.1 (M+, 100%), 627.1 (M + H+, 41), 649.1 (M + Na+, 70); Rf (HPLC) τ = 18.5 min. 1H NMR (600 MHz, CDCl3) δ 6.89 (1 H, s, H-5 or H-8), 6.58 (1 H, s, H-5 or H-8), 6.44 (2 H, s, H-2′ and H-6′), 6.01 (1 H, d, J = 1.2 Hz, H-13) overlapped with 6.01 (1 H, d, J = 8.9 Hz, H-4), 5.99 (1 H, d, J = 1.2 Hz, H-13), 4.85 (1 H, br s, Cp), 4.82 (1 H, br s, Cp), 4.64 (1 H, d, J = 4.3 Hz, H-1), 4.49 (1 H, br s, Cp), 4.48 (1 H, br s, Cp), 4.46 (1 H, dd, J = 9.2, 7.0 Hz, H-11), 4.29 (1 H, t, J = 9.8 Hz, H-11), 4.26 (5 H, s, Cp), 3.81 (3 H, s, 4′-OCH3), 3.80 (6 H, s, 2′ and 5′-OCH3), 2.97 (1 H, dt, J = 14.5, 4.4 Hz, H-2), 2.88–2.93 (1 H, m, H-3); 13C{1H} NMR (150 MHz, CDCl3) δ 173.7 (4-OCO); 172.4 (C-12); 152.7 (CPh); 148.2 (CPh); 147.7 (CPh); 137.4 (CPh); 134.9 (CPh); 132.4 (CPh); 128.8 (CPh), 109.8 (C-5 or C-8); 108.3 (C-2′ and C-6′); 107.0 (C-5 or C-8); 101.6 (C-13); 90.1 (Cpipso), 73.4 (C-4); 71.9 (2 × Cp); 71.6 (C-11); 70.3 (Cp); 70.2 (Cp); 69.8 (Cp); 60.8 (4′-OCH3); 56.2 (3′-OCH3 and 5′-OCH3); 45.7 (C-2); 43.8 (C-1); 38.9 (C-3).
O-(4-Ferrocenylbutyroyl)podophyllotoxin 15.
This compound was synthesized in 86% yield (139 mg) according to general procedure A starting from 99 mg (0.362 mmol) of 4-ferrocenylbutyric acid. Mp 91.2–92.0 °C; elemental analysis found: C, 64.7; H, 5.5. C36H36FeO9 requires C, 64.7; H, 5.4%; λmax (ClCH2CH2Cl)/nm 438, 363, 320 and 288 (ε/dm3 mol−1 cm−1 130, 159, 261 and 5769); IR (KBr) νmax/cm−1: 2928m, 2837m, 1779s, 1732s, 1588m, 1506s, 1484s, 1457m, 1419m, 1379m, 1332m, 1291w, 1240vs, 1172m, 1127vs, 1076m, 1037s, 999s, 932m, 862m, 800m, 766m, 484m; ESI-MS calculated for C36H36FeO9m/z = 668.2; found m/z 668.2 (M+, 100%), 669.2 (M + H+, 49), 691.2 (M + Na+, 41); Rf (HPLC) τ = 20.5 min; 1H NMR (600 MHz, CDCl3) δ 6.75 (1 H, s, H-5 or H-8), 6.54 (1 H, s, H-5 or H-8), 6.39 (2 H, s, H-2′ and H-6′), 5.99 (1 H, d, J = 1.1 Hz, H-13), 5.98 (1 H, d, J = 1.1 Hz, H-13), 5.88 (1 H, d, J = 9.2 Hz, H-4), 4.60 (1 H, d, J = 4.4 Hz, H-1), 4.36 (1 H, dd, J = 8.9, 7.3 Hz, H-11), 4.21–4.13 (10 H, m, Cp and H-11), 3.81 (3 H, s, 4′-OCH3), 3.74 (6 H, s, 3′ and 5′-OCH3), 2.92 (1 H, dd, J = 14.5, 4.4 Hz, H-2), 2.84–2.78 (1 H, m, H-3), 2.48–2.39 (2 H, m, COCH2CH2CH2), 2.36 (2 H, br s, COCH2CH2CH2), 1.85 (2 H, br s, COCH2CH2CH2); 13C{1H} NMR (150 MHz, CDCl3) δ 173.9 (4-OCO), 173.6 (C-12), 152.7 (CPh), 148.1 (CPh), 147.6 (CPh), 137.3 (CPh), 134.8 (CPh), 132.4 (CPh), 128.4 (CPh), 109.7 (C-5 or C-8), 108.2 (C-2′ and C-6′), 107.0 (C-5 or C-8), 101.6 (C-13), 73.5 (C-4), 71.4 (C-11), 69.4 (Cp), 68.9 (Cp), 68.1 (Cp), 60.7 (4′-OCH3), 56.2 (3′ and 5′-OCH3), 45.6 (C-2), 43.8 (C-1), 38.8 (C-3), 33.9 (COCH2CH2CH2), 29.1 (COCH2CH2CH2), 26.3 (COCH2CH2CH2).
O-(3-Ferrocenoylpropionyl)podophyllotoxin 16.
This compound was synthesized in 82% yield (135 mg) according to general procedure A starting from 104 mg (0.362 mmol) of 3-ferrocenoylpropionic acid. Mp 139.5–142.0 °C; elemental analysis found: C, 61.3; H, 5.4. C36H34FeO10·H2O requires C, 61.7; H, 5.2%; λmax (ClCH2CH2Cl)/nm 455, 364, 335 and 272 (ε/dm3 mol−1 cm−1 439, 707, 1346 and 9197); IR (KBr) νmax/cm−1: 3102w, 2922s, 2850m, 1779s, 1732s, 1667s, 1588m, 1506s, 1484s, 1457s, 1420m, 1379m, 1332m, 1240vs, 1156s, 1127vs, 1079m, 1037m, 998m, 930m, 867m, 766m, 481m; ESI-MS calculated for C36H34FeO10m/z = 682.1; found m/z 682.1 (M+, 54%), 683.1 (M + H+, 26), 705.1 (M + Na+, 100); Rf (HPLC) τ = 18.2 min; 1H NMR (CDCl3) δ = 6.91 (1 H, s, H-5 or H-8), 6.54 (1 H, s, H-5 or H-8), 6.41 (2 H, s, H-2′ and H-6′), 6.00 (1 H, d, J = 1.3 Hz, H-13), 5.98 (1 H, d, J = 1.3 Hz, H-13), 5.97 (1 H, d, J = 8.7 Hz, H-4), 4.82 (2 H, br s, Cp), 4.61 (1 H, d, J = 2.9 Hz, H-1), 4.53 (2 H, br s, Cp), 4.46 (1 H, dd, J = 9.2, 6.4 Hz, H-11), 4.26 (5 H, s, Cp), 4.21–4.18 (1 H, m, H-11), 3.82 (3 H, s, 4′-OCH3), 3.76 (6 H, s, 3′ and 5′-OCH3), 3.20–3.09 (2 H, m, COCH2CH2CO), 2.94–2.90 (2 H, m, H-2 and H-3), 2.80–2.69 (2 H, m, COCH2CH2CO); 13C{1H} NMR (CDCl3) δ 201.8 (Fc
O), 173.8 (4-OCO and C-12), 152.7 (CPh), 148.1 (CPh), 147.6 (CPh), 137.3 (CPh), 134.9 (CPh), 132.3 (CPh), 128.5 (CPh), 109.7 (C-5 or C-8), 108.3 (C-2′ and C-6′), 107.2 (C-5 or C-8), 101.5 (C-13), 78.2 (Cpipso), 73.7 (C-4), 72.4 (Cp), 71.5 (C-11), 70.0 (Cp), 69.2 (Cp), 60.7 (4′-OCH3), 56.2 (3′ and 5′-OCH3), 45.6 (C-2), 43.8 (C-1), 38.7 (C-3), 34.3 (COCH2CH2CO), 28.1 (COCH2CH2CO).
O-(4-Ferrocenoylbutyroyl)podophyllotoxin 17.
This compound was synthesized in 80% yield (134 mg) according to general procedure A starting from 109 mg (0.362 mmol) of 4-ferrocenoylbutyric acid. Mp 102–103 °C; elemental analysis found: C, 63.5; H, 5.4. C37H36FeO10 requires C, 63.8; H, 5.2%; λmax (ClCH2CH2Cl)/nm 455, 360 and 329 (ε/dm3 mol−1 cm−1 473, 896 and 1626); IR (KBr) νmax/cm−1: 3096w, 2932m, 2838m, 1779s, 1732s, 1665s, 1588s, 1506s, 1484s, 1456s, 1420s, 1379m, 1332m, 1240vs, 1127vs, 1037s, 1000s, 932m, 867m, 766m, 531m, 482m; ESI-MS calculated for C37H36FeO10m/z = 696.2; found m/z 696.2 (M+, 55%), 697.2 (M + H+, 28), 719.1 (M + Na+, 100); Rf (HPLC) τ = 18.2 min. 1H NMR (CDCl3) δ = 6.77 (1 H, s, H-5 or H-8), 6.53 (1 H, s, H-5 or H-8), 6.39 (2 H, s, H-2′ and H-6′), 5.98 (1 H, d, J = 1.3 Hz, H-13), 5.97 (1 H, d, J = 1.3 Hz, H-13), 5.90 (1 H, d, J = 9.2 Hz, H-4), 4.78–4.77 (2 H, m, Cp), 4.60 (1 H, d, J = 4.4 Hz, H-1), 4.50–4.48 (2 H, m, Cp), 4.38 (1 H, dd, J = 9.2, 7.1 Hz, H-11), 4.20 (1 H, dd, J = 9.4, 1.0 Hz, H-11), 4.19 (5 H, s, Cp), 3.81 (3 H, s, 4′-OCH3), 3.76 (6 H, s, 3′ and 5′-OCH3), 2.92 (1 H, dd, J = 14.5, 4.5 Hz, H-2), 2.87–2.80 (1 H, m, H-3), 2.77–2.75 (2 H, m, 4-OCOCH2CH2CH2CH2), 2.54–2.44 (2 H, m, 4-OCOCH2CH2CH2CH2), 1.79–1.78 (4 H, m, 4-OCOCH2CH2CH2CH2); 13C{1H} NMR (CDCl3) δ 203.6 (
OFc), 173.8 (4-OCO), 173.6 (C-12), 152.7 (CPh), 148.1 (CPh), 147.6 (CPh), 137.3 (CPh), 134.8 (CPh), 132.4 (CPh), 128.4 (CPh), 109.7 (C-5 or C-8), 108.2 (C-2′ and C-6′), 107.0 (C-5 or C-8), 101.6 (C-13), 79.0 (Cpipso), 73.6 (C-4), 72.2 (Cp), 71.4 (C-11), 69.8 (Cp), 69.3 (Cp), 60.7 (4′-OCH3), 56.2 (3′ and 5′-OCH3), 45.6 (C-2), 43.8 (C-1), 39.0 (4-OCOCH2CH2CH2CH2), 38.8 (C-3), 34.3 (4-OCOCH2CH2CH2CH2), 24.8 (4-OCOCH2CH2CH2CH2), 23.7 (4-OCOCH2CH2CH2CH2).
O-(6-Ferrocenylhexanoyl)podophyllotoxin 18.
This compound was synthesized in 81% yield (136 mg) according to general procedure A starting from 109 mg (0.362 mmol) of 6-ferrocenylhexanoic acid. Mp 156–157 °C; elemental analysis found: C, 63.5; H, 6.3. C38H40FeO9·H2O requires C, 63.9; H, 5.9%; λmax (ClCH2CH2Cl)/nm 435, 363 and 288 (ε/dm3 mol−1 cm−1 109, 210 and 5043); IR (KBr) νmax/cm−1: 3091w, 2922m, 2852m, 1766s, 1732s, 1587m, 1507m, 1484s, 1419m, 1375m, 1332m, 1240s, 1225s, 1191m, 1171m, 1128vs, 1031s, 992s, 926m; ESI-MS calculated for C38H40FeO9m/z = 696.2; found m/z 696.1 (M+); Rf (HPLC) τ = 21.6 min. 1H NMR (CDCl3) δ = 6.74 (1 H, s, H-5 or H-8), 6.54 (1 H, s, H-5 or H-8), 6.39 (2 H, s, H-2′ and H-6′), 5.99 (1 H, d, J = 1.3 Hz, H-13), 5.97 (1 H, d, J = 1.2 Hz, H-13), 5.88 (1 H, d, J = 9.2 Hz, H-4), 4.60 (1 H, d, J = 4.4 Hz, H-1), 4.33 (1 H, dd, J = 9.1, 7.1 Hz, H-11), 4.20–4.08 (10 H, m, Cp and H-11), 3.82 (3 H, s, 4′-OCH3), 3.76 (6 H, s, 3′ and 5′-OCH3), 2.91 (1 H, dd, J = 14.5, 4.5 Hz, H-2), 2.83–2.76 (1 H, m, H-3), 2.47–2.37 (2 H, m, (CH2)5), 2.28 (2 H, br s, (CH2)5), 1.71–1.66 (2 H, m, (CH2)5), 1.52–1.50 (2 H, m, (CH2)5), 1.42–1.35 (2 H, m, (CH2)5); 13C{1H} NMR (CDCl3) δ 174.1 (4-OCO), 173.6 (C-12), 152.7 (CPh), 148.1 (CPh), 147.6 (CPh), 137.3 (CPh), 134.8 (CPh), 132.4 (CPh), 128.4 (CPh), 109.7 (C-5 or C-8), 108.2 (C-2′ and C-6′), 107.0 (C-5 or C-8), 101.6 (C-13), 73.4 (C-4), 71.4 (C-11), 69.1 (Cp), 68.7 (Cp), 67.7 (Cp), 60.7 (4′-OCH3), 56.2 (3′ and 5′-OCH3), 45.6 (C-2), 43.8 (C-1), 38.8 (C-3), 34.3 (4-OCO(CH2)5), 30.7 (4-OCO(CH2)5), 29.4 (4-OCO(CH2)5), 28.9 (4-OCO(CH2)5), 24.9 (4-OCO(CH2)5).
General procedure B – synthesis of amides 19–21
4-Amino-4-deoxy-N-ferrocenoyl-4-epipodophyllotoxin 19.
To a mixture of 50 mg (0.121 mmol) of 4-amio-4-deoxy-4-epipodophyllotoxin 5, 30 mg (0.131 mmol) of ferrocenecarboxylic acid, 59 mg (0.133 mmol) of BOP in 5 ml of anhydrous acetonitrile was added 31 mg (42 μl, 0.240 mmol) of DIPEA and the resulting solution was stirred at RT for 40 min. Then the solvent was evaporated to dryness and the crude product was purified by column chromatography on silica (50 ml) using a gradient of dichloromethane–methanol starting from 0 to 3% of methanol. Pure product was obtained as a yellow powder in 70% yield (53 mg). Mp 254–256 °C (dec.); elemental analysis found: C, 61.1; H, 5.6; N, 2.5. C33H31FeNO8·1.5H2O requires C, 60.8; H, 5.2; N, 2.2%; λmax (ClCH2CH2Cl)/nm 444, 345, 294 and 270 (ε/dm3 mol−1 cm−1 263, 521, 5826 and 8627); IR (KBr) νmax/cm−1: 3409s, 2919m, 1769s, 1648s, 1587m, 1503vs, 1482s, 1417m, 1391m, 1329m, 1290m, 1236s, 1188m, 1177m, 1127s, 1105m, 1033m, 999s, 926m, 862m, 826m, 481m; ESI-MS calculated for C33H31FeNO8m/z = 625.1; found m/z 626.1 (M + H+, 100%); Rf (HPLC) τ = 15.5 min. 1H NMR (CDCl3) δ = 6.79 (1 H, s, H-5 or H-8), 6.56 (1 H, s, H-5 or H-8), 6.31 (2 H, s, H-2′ and H-6′), 5.99 (1 H, s, H-13), 5.98 (1 H, s, H-13), 5.74 (1 H, br s, CONH), 5.36 (1 H, br s, H-4), 4.75 (1 H, br s, Cp), 4.69 (1 H, br s, Cp), 4.62 (1 H, br s, H-1), 4.48 (1 H, t, J = 7.9 Hz, H-11), 4.44 (1 H, br s, Cp), 4.43 (1 H, br s, Cp), 4.28 (5 H, s, Cp), 3.93 (1 H, t, J = 9.7 Hz, H-11), 3.81 (3 H, s, 4′-OCH3), 3.76 (6 H, s, 3′- and 5′-OCH3), 3.01 (1 H, br s, H-3), 2.92 (1 H, br s, H-2); 13C{1H} NMR (CDCl3) δ 174.3 (C-12), 152.7 (CPh), 148.5 (CPh), 147.9 (CPh), 137.5 (CPh), 134.7 (CPh), 132.6 (CPh), 129.2 (CPh), 110.3 (C-5 or C-8), 108.9 (C = 5 or C-8), 108.4 (C-2′ and C-6′), 101.7 (C-13), 71.4 (Cp), 70.2 (Cp), 69.2 (C-11), 68.6 (Cp), 60.7 (4′-OCH3), 56.3 (3′ and 5′-OCH3), 48.2 (C-4), 43.9 (C-1), 42.0 (C-2), 37.5 (C-3).
4-Amino-4-deoxy-N-(3-ferrocenoylpropionyl)-4-epipodophyllotoxin 20.
This compound was synthesized in 52% yield (43 mg) according to general procedure B starting from 37 mg (0.131 mmol) of 3-ferrocenoylpropionic acid. Mp > 250 °C (dec.); elemental analysis found: C, 66.2; H, 5.5; N, 2.6. C36H35FeNO9 requires C, 63.4; H, 5.2; N, 2.1%; λmax (ClCH2CH2Cl)/nm 455, 361, 335 and 273 (ε/dm3 mol−1 cm−1 196, 414, 656 and 3766); IR (KBr) νmax/cm−1: 3423vs, 2923s, 2851m, 1773s, 1671s, 1654s, 1589m, 1507s, 1482s, 1459s, 1419m, 1396m, 1329m, 1263m, 1234s, 1190m, 1172m, 1125vs, 1089m, 1030m, 992m, 867m; ESI-MS calculated for C36H35FeNO9m/z = 681.2; found m/z 682.1 (M+, 100%); Rf (HPLC) τ = 15.5 min; 1H NMR (DMSO-d6) δ 8.34 (1 H, d, J = 8.5 Hz, NH), 6.78 (1 H, s, H-5 or H-8), 6.54 (1 H, s, H-5 or H-8), 6.31 (2 H, s, H-2′ and H-6′), 6.01 (1 H, s, H-13), 6.00 (1 H, s, H-13), 5.22 (1 H, dd, J = 8.3, 4.6 Hz, H-4), 4.83 (1 H, br s, Cp), 4.82 (1 H, br s, Cp), 4.57–4.56 (3 H, m, H-1 and Cp), 4.29 (5 H, s, Cp), 4.27 (1 H, t, J = 8.1 Hz, H-11), 3.90 (1 H, dd, J = 10.5, 8.9 Hz, H-11), 3.65 (6 H, s, 3′ and 5′-OCH3), 3.62 (3 H, s, 4′-OCH3), 3.25 (1 H, dd, J = 14.7, 5.5 Hz, H-2), 3.13 (1 H, ddd, J = 17.9, 8.2, 5.3 Hz, 4-NHCOCH2CH2), 3.04–2.99 (1 H, m, 4-NHCOCH2CH2), 2.98–2.93 (1 H, m, H-3), 2.53–2.46 (1 H, m, 4-NHCOCH2CH2, overlapped with DMSO), 2.44–2.40 (1 H, m, 4-NHCOCH2CH2); 13C{1H} NMR (DMSO-d6) 202.2 (COFc), 174.4 (C-12), 171.4 (NHCO), 159.1 (CPh), 147.1 (CPh), 146.5 (CPh), 136.3 (CPh), 135.7 (CPh), 131.7 (CPh), 130.6 (CPh), 109.3 (C-5 or C-8), 109.1 (C-5 or C-8), 101.1 (C-2′ and C-6′), 78.6 (Cpipso), 71.8 (Cp), 69.5 (Cp), 68.9 (Cp), 68.4 (C-13), 59.8 (4′-OCH3), 55.7 (3′ and 5′-OCH3), 46.5 (C-4), 43.0 (C-1), 40.7 (C-2), 36.6 (C-3), 34.1 (4-NHCOCH2CH2), 28.6 (4-NHCOCH2CH2).
4-Dehydroxy-4-amino-N-(4-ferrocenylbutyroyl)-4-epipodophyllotoxin 21.
This compound was synthesized in 72% yield (58 mg) according to general procedure B starting from 36 mg (0.131 mmol) of 4-ferrocenylbutyric acid. Mp 201–202 °C (dec.); elemental analysis found: C, 64.7; H, 6.2; N, 2.8. C36H37FeNO8 requires C, 64.8; H, 5.6; N, 2.1%; λmax (ClCH2CH2Cl)/nm 443, 364 and 320 (ε/dm3 mol−1 cm−1 103, 131 and 218); IR (KBr) νmax/cm−1: 3386m, 2932m, 1776s, 1671m, 1648m, 1589m, 1506vs, 1483vs, 1418m, 1390m, 1332m, 1288m, 1231vs, 1189m, 1161m, 1126vs, 1105s, 1037s, 1000s, 931m, 865m, 802m, 485m; ESI-MS calculated for C36H37FeO8m/z = 667.2; found m/z 667.1 (M+, 100%), 668.1 (M + H+, 58); Rf (HPLC) τ = 17.8 min; 1H NMR (DMSO-d6) δ 8.23 (1 H, d, J = 8.5 Hz, NH), 6.79 (1 H, s, H-5 or H-8), 6.54 (1 H, s, H-5 or H-8), 6.30 (2 H, s, H-2′ and H-6′), 6.01 (1 H, s, H-13), 5.99 (1 H, s, H-13), 5.24 (1 H, dd, J = 8.4, 4.7 Hz, H-4), 4.55 (1 H, d, J = 5.3 Hz, H-1), 4.33 (1 H, t, J = 8.0 Hz, H-11), 4.11 (5 H, s, Cp), 4.10–4.09 (1 H, m, Cp), 4.08–4.07 (1 H, m, Cp), 4.05–4.03 (2 H, m, Cp), 3.76 (1 H, dd, J = 10.8, 8.6 Hz, H-11), 3.65 (6 H, s, 3′ and 5′-OCH3), 3.62 (3 H, s, 4′-OCH3), 3.23 (1 H, dd, J = 14.5, 5.4 Hz, H-2), 2.97–2.94 (1 H, m, H-3), 2.29 (2 H, dd, J = 8.6, 7.0 Hz, (CH2)3), 2.17 (2 H, dt, J = 7.2, 2.6 Hz, (CH2)3), 1.77–1.72 (2 H, m, (CH2)3); 13C{1H} NMR (DMSO-d6) 174.4 (C-12), 171.9 (4-NHCO), 152.0 (CPh), 147.1 (CPh), 146.6 (CPh), 136.4 (CPh), 135.7 (CPh), 131.7 (CPh), 130.8 (CPh), 109.3 (C-5 or C-8), 108.9 (C-5 or C-8), 108.2 (C-2′ and C-6′), 101.2 (C-13), 88.2 (Cpipso), 68.3 (C-11), 68.2 (Cp), 67.7 (Cp), 66.8 (Cp), 59.8 (4′-OCH3), 55.8 (3′ and 5′-OCH3), 46.4 (C-4), 43.0 (C-1), 40.7 (C-2), 36.4 (C-3), 34.9 ((CH2)3), 28.5 ((CH2)3), 26.6 ((CH2)3).
General procedure C – synthesis of 1,2,3-triazoles 29–32
4-Deoxy-4-(4-ferrocenyl-1H-1,2,3-triazol-1-yl)-4-epipodophyllotoxin 29.
100 mg (0.228 mmol) of 4-azido-4-deoxy-4-epipodophyllotoxin 4 and 46 mg (0.219) of ethynylferrocene were dissolved in 10 ml of methanol and 2 ml of water. To the resulting solution, a freshly prepared solution of 0.5 ml of 0.1 M CuSO4 and 0.5 ml of 0.1 M sodium ascorbate and 0.23 ml of 0.01 M TTTA was added. After 20 h of stirring at RT, 50 ml of water was added and the product was extracted with ethyl acetate. The extract was washed twice with water and brine, dried and evaporated to dryness. Chromatography on silica using dichloromethane–ethyl acetate 85
:
15 as the eluent gave pure product in 86% yield (122 mg). Mp > 200 °C (dec.); elemental analysis found: C, 59.4; H, 5.1; N, 6.5. C34H31FeN3O7·2H2O requires C, 59.6; H, 5.2; N, 6.1%; λmax (ClCH2CH2Cl)/nm 412, 357 and 272 (ε/dm3 mol−1 cm−1 572, 1592 and 18
011); IR (KBr) νmax/cm−1: 2911w, 2835w, 1781s, 1629m, 1588s, 1505s, 1484s, 1456m, 1418m, 1391m, 1332m, 1236vs, 1189m, 1158m, 1128vs, 1105s, 1037s, 1004s, 927m, 875m, 504m; ESI-MS calculated for C34H31FeN3O7m/z = 649.2; found m/z 649.1 (M+, 100%); 650.1 (M + H+, 73); Rf (HPLC) τ = 17.1 min 1H NMR (DMSO-d6) 8.08 (1 H, s, Htriazolyl), 6.80 (1 H, s, H-5 or H-8), 6.72 (1 H, s, H-5 or H-8), 6.35 (2 H, s, H-2′ and H-6′), 6.25 (1 H, d, J = 5.4 Hz, H-4), 6.03 (1 H, s, H-13), 6.02 (1 H, s, H-13), 4.76 (1 H, d, J = 5.2 Hz, H-1), 4.74 (1 H, s, Cp), 4.73 (1 H, s, Cp), 4.43 (1 H, t, J = 8.0 Hz, H-11), 4.31 (2 H, s, Cp), 4.01 (5 H, s, Cp), 3.69 (6 H, s, 3′ and 5′-OCH3), 3.65 (3 H, s, 4′-OCH3), 3.57 (1 H, dd, J = 14.7, 5.2 Hz, H-2), 3.32–3.25 (1 H, m, H-3), 2.99 (1 H, dd, J = 10.5, 8.9 Hz, H-11). 13C{1H} NMR (DMSO-d6) 173.5 (C-12), 152.1 (CPh), 148.1 (CPh), 147.0 (CPh), 145.5 (Ctriazolyl), 136.6 (CPh), 135.1 (CPh), 132.8 (CPh), 126.2 (CPh), 121.0 (CHtriazolyl), 109.8 (C-5 or C-8), 108.5 (C-5 or C-8), 108.2 (C-2′ and C-6′), 101.5 (C-13), 75.4 (Cpipso), 69.2 (Cp), 68.3 (Cp), 67.2 (C-11), 66.3 (Cp), 59.9 (4′-OCH3), 57.3 (C-4), 55.8 (3′ and 5′-OCH3), 43.0 (C-1), 40.8 (C-2), 36.5 (C-3).
4-Deoxy-4-(4-ferrocenoyl-1H-1,2,3-triazol-1-yl)-4-epipodophyllotoxin 30.
This compound was synthesized in 75% yield (111 mg) according to general procedure C starting from 52 mg (0.219 mmol) of propynoylferrocene. Mp > 200 °C (dec.); elemental analysis found: C, 61.8; H, 5.2; N, 6.9. C35H31FeN3O8 requires C, 62.0; H, 4.6; N, 6.2%; λmax (ClCH2CH2Cl)/nm 480 and 368 (ε/dm3 mol−1 cm−1 1158 and 2040); IR (KBr) νmax/cm−1: 3121w, 2930m, 2837w, 1782s, 1732w, 1628s, 1588m, 1525m, 1506s, 1485s, 1456m, 1418m, 1378m, 1333m, 1260s, 1238vs, 1190m, 1160m, 1126vs, 1106m, 1093m, 1037s, 1003s, 941m, 841m, 826m, 771m, 676w, 498m; ESI-MS calculated for C35H31FeN3O8m/z = 677.2; found m/z 677.1 (M+, 100%), 678.1 (M + H+, 50), 700.1 (M + Na+, 42); Rf (HPLC) τ = 17.3 min; 1H NMR (DMSO-d6) δ 8.57 (1 H, s, Htriazolyl), 6.90 (1 H, s, H-5 or H-8), 6.71 (1 H, s, H-5 or H-8), 6.36 (1 H, d, J = 5.2 Hz, H-4), 6.35 (2 H, s, H-2′ and H-6′), 6.04 (1 H, s, H-13), 6.01 (1 H, s, H-13), 5.32 (1 H, s, Cp), 5.29 (1 H, s, Cp), 4.77 (1 H, d, J = 5.0 Hz, H-1), 4.71 (2 H, br s, Cp), 4.45 (1 H, t, J = 7.8 Hz, H-11), 4.16 (5 H, s, Cp), 3.69 (6 H, s, 3′ and 5′-OCH3), 3.64 (3 H, s, 4′-OCH3), 3.62 (1 H, dd, J = 9.9, 5.1 Hz, H-2), 3.36–3.30 (1 H, m, H-3), 3.13 (1 H, t, J = 9.7 Hz, H-11); 13C{1H} NMR (DMSO-d6) 187.5 (
OFc), 173.5 (C-12), 152.1 (CPh), 148.2 (CPh), 147.2 (CPh), 147.0 (Ctriazolyl), 136.5 (CPh), 135.2 (CPh), 133.2 (CPh), 128.6 (CHtriazolyl), 125.6 (CPh), 109.8 (C-5 or C-8), 108.7 (C-5 or C-8), 108.1 (C-5 or C-8), 101.6 (C-13), 77.8 (Cpipso), 72.7 (2 × Cp), 71.0 (Cp), 70.8 (Cp), 69.9 (Cp), 67.1 (C-11), 59.9 (4′-OCH3), 57.8 (C-4), 55.8 (3′ and 5′-OCH3), 42.9 (C-1), 40.5 (C-2), 36.4 (C-3).
4-Deoxy-4-(4-(3-ferrocenyl-3-oxoprop-1-yl)-1H-1,2,3-triazol-1-yl)-4-epipodophyllotoxin 31.
This compound was synthesized in 32% yield (49 mg) according to general procedure C starting from 58 mg (0.219 mmol) of (4-pentynoyl)ferrocene. The product was purified on silica using a gradient starting from 9
:
1 to 7
:
3 of dichloromethane–ethyl acetate as the eluent. Mp > 200 °C (dec.); elemental analysis found: C, 63. 0; H, 5.5; N, 6.4. C37H35FeN3O8 requires C, 63.0; H, 5.0; N, 6.0%; λmax (ClCH2CH2Cl)/nm 453, 361 and 335 (ε/dm3 mol−1 cm−1 770, 1375 and 2406); IR (KBr) νmax/cm−1: 2926m, 1780s, 1663s, 1588s, 1506s, 1485s, 1456s, 1418m, 1382m, 1332m, 1237vs, 1189m, 1160m, 1126vs, 1092m, 1036s, 1001s, 930m; ESI-MS calculated for C37H35FeN3O8m/z 705.2; found m/z 705.1 (M+, 63%), 706.1 (M + H+, 100); Rf (HPLC) τ = 15.7 min. 1H NMR (DMSO-d6) δ 7.77 (1 H, s, Htriazolyl), 6.70 (1 H, s, H-5 or H-8), 6.67 (1 H, s, H-5 or H-8), 6.32 (2 H, s, H-2′ and H-6′), 6.21 (1 H, d, J = 5.2 Hz, H-4), 6.02 (1 H, s, H-13), 5.98 (1 H, s, H-13), 4.78 (2 H, br s, Cp), 4.73 (1 H, d, J = 5.2 Hz, H-1), 4.55 (2 H, br t, J = 1.6 Hz, Cp), 4.35 (1 H, t, J = 8.0 Hz, H-11), 4.15 (5 H, s, Cp), 3.67 (6 H, s, 3′ and 5′-OCH3), 3.63 (3 H, s, 4′-OCH3), 3.46 (1 H, dd, J = 14.7, 5.3 Hz, H-2), 3.27–3.21 (1 H, m, H-3), 3.13 (1 H, t, J = 7.4 Hz, CH2CH2COFc), 3.12 (1 H, t, J = 6.6 Hz, CH2CH2COFc), 2.96–2.91 (3 H, m, CH2CH2COFc and H-11); 13C{1H} NMR (DMSO-d6); 202.0 (CH2CH2
OFc), 173.4 (C-12), 152.0 (CPh), 148.0 (CPh), 146.8 (CPh), 146.1 (Ctriazolyl), 136.6 (CPh), 135.2 (CPh), 132.7 (CPh), 126.1 (CPh), 122.9 (CHtriazolyl), 109.7 (C-5 or C-8), 108.7 (C-5 or C-8), 108.1 (C-2′ and C-6′), 101.5 (C-13), 78.7 (Cpipso), 72.0 (Cp), 71.9 (Cp), 69.4 (Cp), 69.0 (Cp), 68.9 (Cp), 67.1 (C-11), 59.8 (4′-OCH3), 57.1 (C-4), 55.8 (3′ and 5′-OCH3), 42.9 (C-1), 40.7 (C-2), 37.9 (CH2CH2COFc), 36.4 (C-3), 19.6 (CH2CH2COFc).
4-Deoxy-4-(4-(4-ferrocenyl-4-oxobut-1-yl)-1H-1,2,3-triazol-1-yl)-4-epipodophyllotoxin 32.
This compound was synthesized in 17% yield (27 mg) according to general procedure C starting from 61 mg (0.219 mmol) of (5-hexynoyl)ferrocene. The product was purified on silica using a gradient starting from 9
:
1 to 9
:
3 of dichloromethane–ethyl acetate as the eluent. Mp 194–195 °C (dec.); elemental analysis found: C-63.1; H-6.1; N-6.1. C38H37FeN3O8 requires C, 63.4; H, 5.2; N, 5.8%; λmax (ClCH2CH2Cl)/nm 453, 358 and 333 (ε/dm3 mol−1 cm−1 901, 1786 and 2031); IR (KBr) νmax/cm−1: 2952s, 1781s, 1663s, 1588s, 1506s, 1485s, 1456s, 1418m, 1381m, 1332m, 1287m, 1238vs, 1189m, 1160m, 1126vs, 1106m, 1092m, 1037s, 1002s, 931m, 825m, 485m; ESI-MS calculated for C38H37FeN3O8m/z = 719.2; found m/z 719.2 (M+, 37%), 720.1 (M + H+, 100); Rf (HPLC) τ = 16.2 min. 1H NMR (DMSO-d6) δ 7.79 (1 H, s, Htriazolyl), 6.75 (1 H, s, H-5 or H-8), 6.68 (1 H, s, H-5 or H-8), 6.33 (2 H, s, H-2′ and H-6′), 6.22 (1 H, d, J = 5.1 Hz, H-4), 6.03 (1 H, s, H-13), 5.99 (1 H, s, H-13), 4.74 (2 H, br s, Cp), 4.72 (1 H, d, J = 5.2 Hz, H-1), 4.54 (2 H, br s, Cp), 4.38 (1 H, t, J = 7.9 Hz, H-11), 4.19 (5 H, s, Cp), 3.68 (6 H, s, 3′ and 5′-OCH3), 3.64 (3 H, s, 4′-OCH3), 3.51 (1 H, dd, J = 14.8, 5.1 Hz, H-2), 3.29–3.22 (1 H, m, H-3), 2.97 (1 H, t, J = 9.6 Hz, H-11), 2.78 (2 H, t, J = 7.2 Hz, CH2CH2CH2COFc), 2.70 (2 H, t, J = 7.3 Hz, CH2CH2CH2COFc), 1.95–1.91 (2 H, m, CH2CH2CH2COFc); 13C{1H} NMR (DMSO-d6) 203.0 (CH2CH2CH2COFc), 173.5 (C-12), 152.1 (2 × CPh), 148.0 (CPh), 146.9 (Ctriazolyl), 146.5 (Ctriazolyl), 136.5 (CPh), 135.2 (CPh), 132.8 (CPh), 126.2 (CPh), 122.7 (CHtriazolyl), 109.7 (C-5 or C-8), 108.6 (C-5 or C-8), 108.1 (C-2′ and C-6′), 101.5 (C-13), 79.0 (Cpipso), 71.9 (Cp), 69.4 (Cp), 68.9 (Cp), 67.2 (C-11), 59.9 (4′-OCH3), 57.2 (C-4), 55.8 (3′ and 5′-OCH3), 42.9 (C-1), 40.7 (C-2), 38.0 (CH2CH2CH2COFc), 36.5 (C-3), 24.5 (CH2CH2CH2COFc), 23.5 (CH2CH2CH2COFc).
Viability assay
Solutions of all the tested compounds were prepared freshly for every experiment and processed immediately. Stock solutions were prepared in DMSO and all compounds were added to cells to a final DMSO concentration of 0.2% (v/v), while controls were incubated with 0.2% DMSO alone. The chosen DMSO concentration was determined to be non-toxic to the cells.
The drug sensitivity of the cell lines was determined using the neutral red uptake assay.29 Briefly, cells were seeded on 96-well plates at a density of 10
000 per well and 24 h later were treated with either the control or the test compound at a desired concentration. After 70 h of incubation, neutral red was added to the medium to a final concentration of 1 mM. After 2 h of incubation, the cells were washed with PBS, dissolved in 200 μL solubilisation solution (1% HOAc (v/v) in 50% EtOH (v/v)) and shaken for 10 min, until the neutral red was extracted from the cells. The absorbance was measured at 540 nm with an EnVisionMultilabel Plate Reader (PerkinElmer). The results were calculated as a percentage of the controls and the IC50 values for each cell line and substance were calculated with GraphPad Prism 5.02 software (GraphPad Inc.) using four-parameter non-linear logistic regression.
Tubulin polymerization assay
The tubulin polymerization rate was determined using a fluorescence-based tubulin polymerization assay (Cytoskeleton, Inc., cat. no. BK011P) according to the manufacturer's instructions. The investigated compounds were tested over the concentration range 0.1–30 μM using freshly prepared DMSO stock solutions and the final DMSO concentration of 1% was kept constant across all samples. Paclitaxel was used as a positive control for tubulin polymerization and the results were compared to the solvent control (buffer + DMSO). The fluorescence was measured at 37 °C for 120 min at 355/40 nm excitation and 430/8 nm emission wavelengths using an EnVisionMultilabel Plate Reader (PerkinElmer).
Cell cycle analysis
Exponentially growing cells (100
000 cells per well seeded in 6-well plates 24 h before time 0) were treated with 10 nM of the test compound for 48 h. Cells were then harvested by trypsinisation, washed twice with ice-cold PBS and fixed in 70% ethanol. After storing the cells for at least 8 h at 4 °C, they were stained with a propidium iodide staining solution (75 μM propidium iodide and 50 Kunitz units per mL of RNAse A in PBS) for 30 min at 37 °C. The samples were analysed on an LSRII (Becton Dickinson) instrument and cell cycle phase distributions were determined with FlowJo 7.6.1 software (FlowJo, LLC) using a built-in cell cycle analysis module (Watson pragmatic algorithm).
Investigation of cellular esterase effects on ferrocenyl esters
Roughly 20 million Hep G2 cells were harvested via trypsinisation and centrifuged (100g for 10 min at RT). The supernatant was removed by aspiration and the cell pellets were suspended in 1 mL of ice-cold MilliQ-quality water. The cells were placed on an ice-bath and sonicated (2 min of maximum power). The cells were inspected under a microscope and no intact cells were found in the resulting crude cell homogenate. No protease inhibitors were added to the homogenate to avoid non-specific inhibition of other hydrolases. A volume of 100 μL of crude cell extract was mixed with 100 μL of DMEM for pH stabilisation and then the investigated compound was added from the DMSO stock solution to a final concentration of 1 mM. The final concentration of DMSO in the sample was 1% v/v. The mixture was incubated at 37 °C and samples were taken after 1 h and 2 h of incubation. A volume of 100 μL of sample was mixed with 100 μL of ice-cold 10% w/v solution of TCA in water and allowed to incubate for approx. 10 min on ice to precipitate proteins. The sample was centrifuged (10
000 RPM for 10 min at RT) and the supernatant was carefully aspirated, filtered through a 0.22 μm PTFE filter and processed via HPLC. The DMSO extract of the protein pellet was also prepared and analysed via HPLC. Oregon Green diacetate was used as a positive control for esterase activity. It was completely digested after 1 h of incubation with crude cell extract.
Acknowledgements
This study was financially supported by the National Science Centre Poland (NCN) based on decision DEC-2012/05/B/ST5/00300. A. M. also acknowledges the National Science Centre Poland (NCN) for financial support (decision DEC-2012/04/A/ST5/00609), which enabled the X-ray structural analysis of the compound.
References
- M. L. King and M. M. Sullivan, Science, 1946, 104, 244–245 CAS.
- J. Bryan, Biochemistry, 1972, 11, 2611–2616 CrossRef CAS PubMed.
- D. K. Kalwinsky, A. T. Look, J. Ducore and A. Fridland, Cancer Res., 1983, 43, 1592–1597 CAS.
- W. Ross, T. Rowe, B. Glisson, J. Yalowich and L. Liu, Cancer Res., 1984, 44, 5857–5860 CAS.
- Y. Q. Liu, J. Tian, K. Qian, X. B. Zhao, S. L. Morris-Natschke, L. Yang, X. Nan, X. Tian and K. H. Lee, Med. Res. Rev., 2015, 35, 1–62 CrossRef PubMed.
-
(a) L. Zhang, Z. Zhang, J. Wang, Y. Chen, F. Chen, Y. Lin and X. Zhu, RSC Adv., 2016, 6, 2895–2903 RSC;
(b) L. Zhang, Z. Zhang, F. Chen, Y. Chen, Y. Lin and J. Wang, Eur. J. Med. Chem., 2016, 123, 226–235 CrossRef CAS PubMed.
- X. W. Guan, X. H. Xu, S. L. Feng, Z. B. Tang, S. W. Chen and L. Hui, Bioorg. Med. Chem. Lett., 2016, 26, 1561–1566 CrossRef CAS PubMed.
-
(a) A. Kamal, V. L. Nayak, C. Bagul, M. V. P. S. Vishnuvardhan and A. Mallareddy, Apoptosis, 2015, 20, 1518–1529 CrossRef CAS PubMed;
(b) J. Y. Choi, W. G. Hong, J. H. Cho, E. M. Kim, J. Kim, C. H. Jung, S. G. Hwang, H. D. Um and J. K. Park, Int. J. Oncol., 2015, 47, 1257–1265 CAS.
- D. Tierny, F. Serres, Z. Segaoula, I. Bemelmans, E. Bouchaert, A. Pétain, V. Brel, S. Couffin, T. Marchal, L. Nguyen, X. Thuru, P. Ferré, N. Guilbaud and B. Gomes, Clin. Cancer Res., 2015, 21, 5314–5323 CrossRef CAS PubMed.
-
https://www.clinicaltrialsregister.eu/ctr-search/trial/2012-005241-20/IT
.
-
(a) A. Mariani, A. Bartoli, M. Atwal, K. C. Lee, C. A. Austin and R. Rodriguez, J. Med. Chem., 2015, 58, 4851–4856 CrossRef CAS PubMed;
(b) A. H. Banday, V. V. Kulkarni and V. J. Hruby, MedChemComm, 2015, 6, 94–104 RSC.
- I. Hyder, D. Yedlapudi, S. V. Kalivendi, J. Khazir, T. Ismail, N. Nalla, S. Miryala and H. M. Sampath Kumar, Bioorg. Med. Chem. Lett., 2015, 25, 2860–2863 CrossRef CAS PubMed.
-
G. Jaouen and M. Salmain, Bioorganometallic Chemistry: Applications in Drug Discovery, Biocatalysis, and Imaging, 2015 Search PubMed.
-
(a) B. Pérès, R. Nasr, M. Zarioh, F. Lecerf-Schmidt, A. Di Pietro, H. Baubichon-Cortay and A. Boumendjel, Eur. J. Med. Chem., 2017, 130, 346–353 CrossRef PubMed;
(b) J. L. Kedge, H. V. Nguyen, Z. Khan, L. Male, N. J. Hodges, S. L. Horswell, Y. Mehellou, J. H. R. Tucker and H. V. Roberts, Eur. J. Inorg. Chem., 2017, 2017, 466–476 CrossRef CAS;
(c) A. Wieczorek, A. Błauż, J. Zakrzewski, B. Rychlik and D. Plażuk, ACS Med. Chem. Lett., 2016, 7, 612–617 CrossRef CAS PubMed;
(d) A. Gross, H. Alborzinia, S. Piantavigna, L. L. Martin, S. Wölfl and N. Metzler-Nolte, Metallomics, 2015, 7, 371–384 RSC;
(e) D. Plazuk, B. Rychlik, A. Błauz and S. Domagała, J. Organomet. Chem., 2012, 715, 102–112 CrossRef CAS;
(f) J. Spencer, J. Amin, M. Wang, G. Packham, S. S. S. Alwi, G. J. Tizzard, S. J. Coles, R. M. Paranal, J. E. Bradner and T. D. Heightman, ACS Med. Chem. Lett., 2011, 2, 358–362 CrossRef CAS PubMed;
(g) E. Hillard, A. Vessières, F. Le Bideau, D. Plazuk, D. Spera, M. Huché and G. Jaouen, ChemMedChem, 2006, 1, 551–559 CrossRef CAS PubMed;
(h) A. I. Gutiérrez-Hernández, J. G. López-Cortés, M. C. Ortega-Alfaro, M. T. Ramírez-Apan, J. De Jesús Cázares-Marinero and R. A. Toscano, J. Med. Chem., 2012, 55, 4652–4663 CrossRef PubMed;
(i) S. Top, B. Dauer, J. Vaissermann and G. Jaouen, J. Organomet. Chem., 1997, 541, 355–361 CrossRef CAS.
-
(a) D. Scutaru, L. Tataru, I. Mazilu, M. Vata, T. Lixandru and C. Simionescu, Appl. Organomet. Chem., 1993, 7, 225–231 CrossRef CAS;
(b) B. Long, C. He, Y. Yang and J. Xiang, Eur. J. Med. Chem., 2010, 45, 1181–1188 CrossRef CAS PubMed;
(c) M. Görmen, M. S. I. Veitía, F. Trigui, M. El Arbi and C. Ferroud, J. Organomet. Chem., 2015, 794, 274–281 CrossRef;
(d) M. Patra, M. Wenzel, P. Prochnow, V. Pierroz, G. Gasser, J. E. Bandow and N. Metzler-Nolte, Chem. Sci., 2015, 6, 214–224 RSC.
-
(a) C. Biot, G. Glorian, L. A. Maciejewski, J. S. Brocard, O. Domarle, G. Blampain, P. Millet, A. J. Georges and J. Lebibi, J. Med. Chem., 1997, 40, 3715–3718 CrossRef CAS PubMed;
(b) C. Biot, L. Delhaes, L. A. MacIejewski, M. Mortuaire, D. Camus, D. Dive and J. S. Brocard, Eur. J. Med. Chem., 2000, 35, 707–714 CrossRef CAS PubMed;
(c) X. Wu, P. Wilairat and M. L. Go, Bioorg. Med. Chem. Lett., 2002, 12, 2299–2302 CrossRef CAS PubMed;
(d) A. Patti, S. Pedotti, T. Grassi, A. Idolo, M. Guido and A. De Donno, J. Organomet. Chem., 2012, 716, 216–221 CrossRef CAS.
- M. Beaupérin, D. Polat, F. Roudesly, S. Top, A. Vessières, J. Oble, G. Jaouen and G. Poli, J. Organomet. Chem., 2017, 839, 83–90 CrossRef.
-
(a) D. Plazuk, A. Wieczorek, A. Blauz and B. Rychlik, MedChemComm, 2012, 3, 498–501 RSC;
(b) A. Wieczorek, A. Błauż, A. Żal, H. J. Arabshahi, J. Reynisson, C. G. Hartinger, B. Rychlik and D. Plażuk, Chem. – Eur. J., 2016, 22, 11413–11421 CrossRef CAS PubMed.
- J. Ren, L. Wu, W. Q. Xin, X. Chen and K. Hu, Bioorg. Med. Chem. Lett., 2012, 22, 4778–4782 CrossRef CAS PubMed.
-
J. C. Quirion, G. L. I. C. Deliencourt-Godefroy and C. Audouard, Pat., US318675, 2009 Search PubMed.
-
S. Saaby, I. Winckelmann, K. Sondergaard, X. Liang, Y. Ke, X. Wang and J. Ye, WO2010094164 A1, 2010.
- K. J. Rinehart Jr., R. J. Curby Jr. and P. E. Sokol, J. Am. Chem. Soc., 1957, 79, 3420–3424 CrossRef.
- I. Suzuki, Q. Chen, A. Ueno and T. Osa, Bull. Chem. Soc. Jpn., 1993, 66, 1472–1481 CrossRef CAS.
- D. Plazuk and J. Zakrzewski, J. Organomet. Chem., 2009, 694, 1802–1806 CrossRef CAS.
- A. Blauz and B. Rychlik, J. Pharmacol. Toxicol. Methods, 2017, 84, 57–65 CrossRef CAS PubMed.
- M. A. Jordan and L. Wilson, Nat. Rev. Cancer, 2004, 4, 253–265 CrossRef CAS PubMed.
- D. Plazuk and J. Zakrzewski, Synth. Commun., 2004, 34, 99–107 CrossRef CAS.
- H. Zeng and H. Zhang, Eur. J. Med. Chem., 2014, 72, 10–17 CrossRef CAS PubMed.
- G. Repetto, A. Del Peso and J. L. Zurita, Nat. Protoc., 2008, 3, 1125–1131 CrossRef CAS PubMed.
Footnote |
† Electronic supplementary information (ESI) available. CCDC 1552895. For ESI and crystallographic data in CIF or other electronic format see DOI: 10.1039/c7dt02107k |
|
This journal is © The Royal Society of Chemistry 2017 |