DOI:
10.1039/C7AY90114C
(AMC Technical Brief)
Anal. Methods, 2017,
9, 5086-5090
A ‘Periodic Table’ of mass spectrometry instrumentation and acronyms†
Received
8th August 2017
, Accepted 8th August 2017
First published on 24th August 2017
Abstract
The development and maturation of mass spectrometry (MS) technology has meant that MS users are no longer required to be experts in this field. With the increasing popularity of mass spectrometry (MS) with non-expert users, the Analytical Methods Committee (AMC) of the Royal Society of Chemistry has highlighted the need to generate a glossary of instrumentation terms that is accessible to users of this technology at all levels. In order to address a wide range of users it is also necessary to provide a context and explanation for the types of MS technology and their acronyms.
The number of acronyms is growing all the time, especially for hyphenated techniques, and overcoming their mystique was a key aim for the glossary. The ever-widening range of users has led to variations in the way terminology has been applied and in an attempt to avoid confusion the International Union of Pure and Applied Chemistry (IUPAC) has undertaken a review of MS nomenclature. This is featured in a recent extensive publication by IUPAC, which briefly describes over 500 terms used in the field and should be used as the defining resource for mass spectrometrists.1 However, for the less experienced MS user the IUPAC document may require further elaboration, as its aim, rightly so, is to define terms for the specialist and not explain their basis.
Aim
The purpose of this manuscript is to describe the structure of typical MS instrumentation (sample introduction, ionisation source, mass analyser, detector, data acquisition/processing) whereby each section will be dealt with in turn, and summarised in tabulated format. With the many different types of instrumentation there is a clear need for understanding not just the relevant acronyms of the technologies involved but when and, importantly, why they are used in combination. The AMC decided in preparing such a guide that the form of a recognisable ‘Periodic Table’ (Fig. 1) is ideal for presenting the terminology to (non-expert) users of MS instrumentation. In particular it should facilitate decoding future sets of acronyms as well as those already in use. Most of the terms in the table are cross-referenced to the IUPAC document but it is not feasible to include over 500 terms in the table format. Hence, the acronym entries have been limited to those most widely used, and categories such as the atmospheric and ambient ionisation methods are ‘illustrative’ rather than comprehensive.
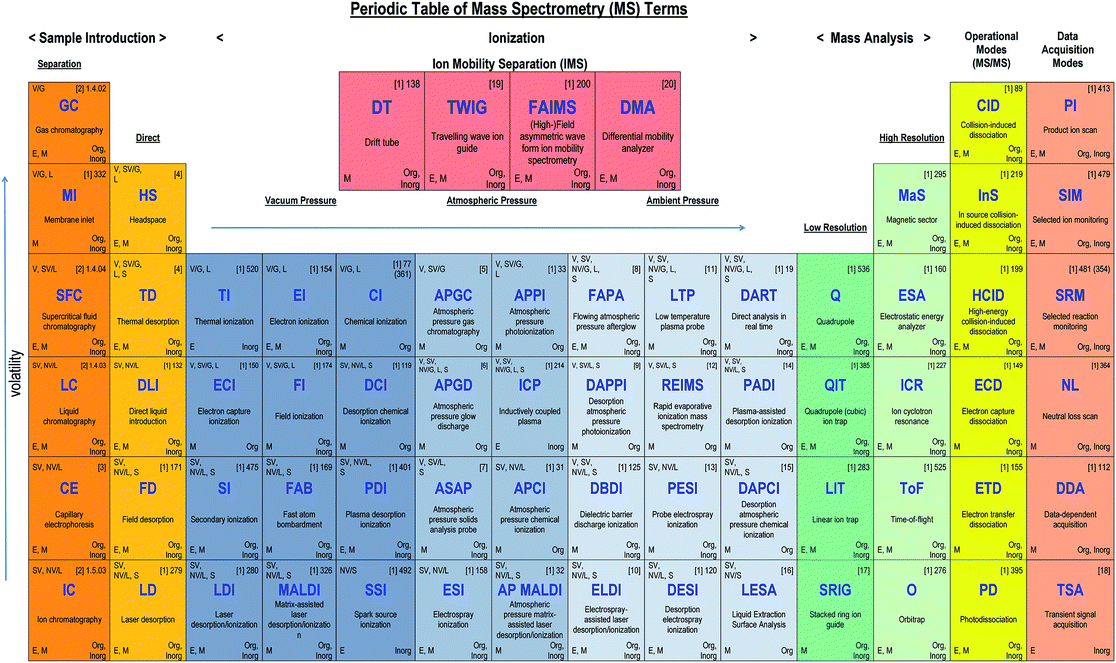 |
| Fig. 1 Periodic table of mass spectrometry (MS) terms. | |
Introduction
Mass spectrometry is the study of ions that are counted according to their mass-to-charge ratio (m/z). The field of MS and the resulting technology has expanded rapidly in the last 30 years to accommodate a range of applications for the analysis of both organic and inorganic compounds. As a result of technological developments, the number of acronyms, abbreviations and terms encountered in the literature has also increased. Mass spectrometers are available in a range of shapes and sizes, and can perform quite different tasks depending on how they are operated. However, they typically consist of the following components:
(1) Sample introduction
(2) Ionisation source
(3) Mass analyser
(4) Detector
(5) Data acquisition/processing
Samples that are analysed by MS can be in solid, liquid or gas form providing the sample can be ionised for mass analysis. The sample introduction method and ionisation sources available depend on the particular requirement. There are also a range of mass analysers available; each mass analyser will have characteristics that will lend itself to gaining information-rich data (mass spectra), for qualitative analysis or for measuring the relative abundance of a compound for quantification. Therefore, there is a broad and varied landscape of available techniques, for the analysis of both organic and inorganic materials, that may be combined to assemble a mass spectrometer designed to meet a specific purpose.
Sample introduction
The sample introduction to a mass spectrometer is chosen according to the nature of the analyte and the complexity of the test material. Analysis of simple test materials, such as ‘pure’ substances, may use straightforward sample introduction such as direct infusion of liquids or headspace sampling of a gas. More complex samples typically require a preliminary separation, either offline or online, by using techniques such as chromatography or electrophoresis to overcome the limitations of the other components of the mass spectrometer. This flexible functionality of the sample introduction enables the advantages of MS, specifically selectivity and sensitivity, to be enhanced and provide a platform capable of trace level detection and quantitation of analytes within complex mixtures.
Ionisation source
Ionisation of target components of the sample can be carried out under a range of conditions, from atmospheric pressure to near vacuum. The ionisation source is chosen to suit the analysis of certain sample chemistries and is used in conjunction with compatible sample introduction methods. Ionisation techniques include chemical modification, thermal desorption, particle bombardment, and laser ablation, with vaporisation (if necessary) into the gas phase. Typical ionisation processes include the loss or addition of an electron, or a charged reagent species and are regarded as high energy (hard ionisation) or low energy (soft ionisation) processes causing significant or minimal fragmentation of the precursor species respectively. Appropriate selection may provide an information-rich data set of chemical structure (hard) or intact elemental/molecular information (soft).
Mass analyser (ion separation)
Mass analysis involves the separation of sample ions according to their m/z. There are a number of methods available to separate ions: spatially (by application of electric or magnetic fields), by measuring an ion’s time-of-flight (ToF), or by monitoring the frequency of ion motion. (This process of separation is carried out under low pressure (typically vacuum) and is quite different to the technique known as ion mobility, which can separate species according to shape and size and can be carried out under atmospheric conditions.) The methods of mass separation may also be classified according to how the analyser operates for the ions to be detected; typically, ions are ‘scanned’, as in a quadrupole analyser, or ‘pulsed’ as in a time-of-flight analyser. These operational considerations are suited to particular applications and are chosen for achieving specific information and combined with compatible ionisation sources and methods of detection.
Detectors
Abbreviations associated with mass spectrometers typically concern sample introduction, ionisation and mass analysis but this should not lessen the importance of ion detection methods. Ion detection will typically involve either the collection of ions (e.g., the Faraday cup), the bombardment of a charged surface to generate secondary electrons (e.g., the electron multiplier), or the monitoring of the frequency of ion movement between electrodes (e.g., image current detection) after ions have exited the mass analyser. Detectors are chosen to suit particular mass analysers and according to whether the accuracy of the ion count or the sensitivity of detection is of primary importance for the analysis.
Data acquisition/processing
Signals generated from the detection of ions require processing before viewing as an output (e.g., a mass spectrum) and this can be accomplished using different methods that involve digitising an electrical signal. Typically, this is achieved by monitoring the current from the detector. That can provide a voltage or ion counting signal (time-to-digital converter, TDC, or analogue-to-digital converter, ADC) or a Fourier transform of a frequency signal derived from an ion’s motion, and converting this into a digital signal that is further processed. Through the operational software of the mass spectrometer the user is then able to observe the mass spectrum, and interrogate and process the data for the desired application.
Operational modes
Mass spectra may be obtained using different acquisition modes covering a broad selection of m/z, such as a ‘full scan’, or specified m/z in the form of single or selected ion monitoring (SIM). Spectra may also be recorded for fragment ions of the precursor species with a range of approaches used to elicit breaking of the chemical structure. These fragmentation methods may be undertaken using suitable ionisation sources or mass analysers (i.e. a ‘hard’ electron ionisation source, or ion trap, respectively), or by using a collision cell with the mass analyser, such as those used in tandem mass spectrometers (e.g. MS/MS). Again, the combination of instrumentation components is chosen to achieve certain types of data necessary for specific applications.
Using the ‘Periodic Table’
(Note: the Table in the printed version of the Brief is for illustration only with no live links. The ESI† version contains links to access references for further information on each acronym.)
Mass spectrometry instruments and techniques are commonly named by using a string of acronyms each of which refers to one of the five major components described above. The string is usually assembled from left to right, beginning with sample introduction and finishing with the operational or data acquisition mode. The table reflects this in a series of columns moving across from left to right. Each column lists the more widely used current options for that component as a vertical series of boxes for which the key entry is the acronym of that option. Additional boxes for ion mobility separation are included in a separate row from the main table as variations of this technique may be used for sample introduction or mass analysis. Within each box is the description of the acronym and a number of abbreviations providing useful information about the component, as indicated in the legend to the table shown also in Fig. 2 below. The entry at top right in each box comprises the corresponding IUPAC number (if available) and a reference for further information. This reference can be accessed directly with electronic versions of the paper by clicking the acronym. An example of using the table to decipher technique acronyms is given at the top of the table shown also in Fig. 3 below.
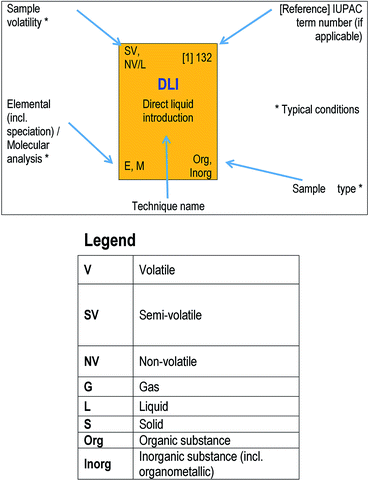 |
| Fig. 2 Legend to the table. | |
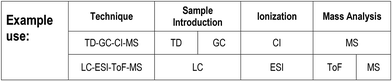 |
| Fig. 3 Example of using the table to decipher the acronym of a technique. | |
Concluding remarks
The expanded range of instrumentation types available enables MS users to carry out a more targeted approach to analysis. This allows the analyst to tailor the method development to achieve the information necessary for the test material and the desired outcome. With the wide choice of instrumentation there is also great flexibility by virtue of certain modules (i.e. the sample introduction and ionisation source) often being interchangeable. This is evident in recent instrument developments offering alternative methods of ionisation within a universal housing. However, with these developments there is perforce a need to understand the relevant acronyms and when and why the technologies are used in combination. This Periodic Table of MS terms may offer some assistance in understanding these combinations.
For techniques not covered by IUPAC/terminology guides within the reference list seminal references have been cited where possible.
A. R. Godfrey (Swansea University Medical School)
This report was prepared for the Analytical Methods Committee with contributions from members of the AMC Instrumental Analysis Sub-committee and approved by the AMC on 03/07/17.
References list
- International Union of Pure and Applied Chemistry (IUPAC), Definitions of terms relating to mass spectrometry (IUPAC Recommendations 2013), Pure Appl. Chem., 2013, 85(7), 1515–1609 Search PubMed.
- International Union of Pure and Applied Chemistry (IUPAC), Nomenclature for chromatography (IUPAC Recommendations 1993), Pure Appl. Chem., 1993, 65(4), 819–872 Search PubMed.
- International Union of Pure and Applied Chemistry (IUPAC), Terminology for analytical capillary electromigration techniques (IUPAC Recommendations 2003), Pure Appl. Chem., 2004, 76(2), 443–451 Search PubMed.
- P. Price, Standard Definitions of Terms Relating to Mass Spectrometry: A Report from the Committee on Measurements and Standards of the American Society for Mass Spectrometry, J. Am. Soc. Mass Spectrom., 1991, 2, 336–348 CrossRef CAS PubMed.
- C. N. McEwen and R. G. McKay, A Combination Atmospheric Pressure LC/MS: GC/MS Ion Source: Advantages of Dual AP-LC/MS: GC/MS Instrumentation, J. Am. Soc. Mass Spectrom., 2005, 16, 1730–1738 CrossRef CAS PubMed.
- I. Sofer, J. Zhu, H.-S. Lee, W. Antos and D. M. Lubman, An Atmospheric-Pressure Glow Discharge Ionization Source, Appl. Spectrosc., 1990, 44(8), 1391–1398 CrossRef CAS.
- C. N. McEwen, R. G. McKay and B. S. Larsen, Analysis of Solids, Liquids, and Biological Tissues Using Solids Probe Introduction at Atmospheric Pressure on Commercial LC/MS Instruments, Anal. Chem., 2005, 77, 7826–7831 CrossRef CAS PubMed.
- F. J. Andrade, J. T. Shelley, W. C. Wetzel, M. R. Webb, G. Gamez, S. J. Ray and G. M. Hieftje, Atmospheric Pressure Chemical Ionization Source.1. Ionization of Compounds in the Gas Phase, Anal. Chem., 2008, 80, 2646–2653 CrossRef CAS PubMed.
- M. Haapala, J. PoI, V. Saarela, V. Arvola, T. Kotiaho, R. A. Ketola, S. Franssila, T. J. Kauppila and R. Kostiainen, Desorption Atmospheric Pressure Photoionization, Anal. Chem., 2007, 79, 7867–7872 CrossRef CAS PubMed.
- J. Shiea, M.-Z. Huang, H.-J. HSu, C.-Y. Lee, C.-H. Yuan, I. Beech and J. Sunner, Electrospray-assisted laser desorption/ionization mass spectrometry for direct ambient analysis of solids, Rapid Commun. Mass Spectrom., 2005, 19, 3701–3704 CrossRef CAS PubMed.
- J. D. Harper, N. A. Charipar, C. C. Mulligan, X. Zhang, R. G. Cooks and Z. Ouyang, Low-Temperature Plasma Probe for Ambient Desorption Ionization, Anal. Chem., 2008, 80, 9097–9104 CrossRef CAS PubMed.
- K.-C. Schafer, J. Denes, K. Albrecht, T. Szaniszlo, J. Balog, R. Skoumal, M. Katona, M. Toth, L. Balogh and Z. Takats, In Vivo, In Situ Tissue Analysis Using Rapid Evaporative Ionization Mass Spectrometry, Angew. Chem., Int. Ed., 2009, 48, 8240–8242 CrossRef PubMed.
- K. Hiraoka, K. Nishidate, K. Mori, D. Asakawa and S. Suzuki, Development of probe electrospray using a solid needle, Rapid Commun. Mass Spectrom., 2007, 21, 3139–3144 CrossRef CAS PubMed.
- L. V. Ratcliffe, F. J. M. Rutten, D. A. Barrett, T. Whitmore, D. Seymour, C. Greenwood, Y. Aranda-Gonzalvo, S. Robinson and M. McCoustra, Surface Analysis under Ambient Conditions Using Plasma-Assisted Desorption/Ionization Mass Spectrometry, Anal. Chem., 2007, 79(16), 6094–6101 CrossRef CAS PubMed.
- Z. Takats, I. Cotte-Rodriguez, N. Talaty, H. Chen and R. G. Cooks, Direct, trace level detection of explosives on ambient surfaces by desorption electrospray ionization mass spectrometry, Chem. Commun., 2005, 1950–1952 RSC.
- V. Kertesz and G. J. Van Berkel, Fully automated liquid extraction-based surface sampling and ionization using a chip-based robotic nanoelectrospray platform, J. Mass Spectrom., 2010, 45, 252–260 CrossRef CAS PubMed.
-
D. Gerlich, in State Selected and State-to-State Ion–Molecule Reaction Dynamics. Part 1. Experiment, ed. C. Y. Ng and M. Baer, Wiley, New York, 1992, vol. LXXXII, pp. 1–176 Search PubMed.
- H. P. Longerich, S. E. Jackson and D. Gunther, Laser ablation inductively coupled plasma mass spectrometric transient signal data acquisition and analyte concentration calculation, J. Anal. At. Spectrom., 1996, 11, 899–904 RSC.
- K. Giles, S. D. Pringle, K. R. Worthington, D. Little, J. L. Wildgoose and R. H. Bateman, Applications of a travelling wave-based radio-frequency-only stacked ring ion guide, Rapid Commun. Mass Spectrom., 2004, 18, 2401–2414 CrossRef CAS PubMed.
- E. Knutson and K. Whitby, Aerosol classification by electric mobility: Apparatus, theory, and applications, J. Aerosol Sci., 1975, 6(6), 443–451 CrossRef.
Footnote |
† Electronic supplementary information (ESI) available: The ESI file contains a copy of the Table that can be zoomed for greater clarity or for display. It has links to further information and references, obtained by clicking the large acronym at the centre of each cell. See DOI: 10.1039/c7ay90114c |
|
This journal is © The Royal Society of Chemistry 2017 |