DOI:
10.1039/C7RA03765A
(Paper)
RSC Adv., 2017,
7, 27974-27980
Copper-catalyzed N-alkylation of indoles by N-tosylhydrazones†
Received
1st April 2017
, Accepted 17th May 2017
First published on 26th May 2017
Abstract
An efficient method for the direct N-alkylation of indoles via copper-catalyzed reductive cross coupling between N-tosylhydrazones and indole reagents has been developed. The reaction was performed in the presence of copper iodide and potassium hydroxide by utilization of tri(p-tolyl)phosphine as a ligand. A wide variety of N-alkylated indoles were obtained in moderate to good yields. The application of the current method to the synthesis of biologically active N-alkylated indole derivatives has also been demonstrated.
Introduction
The indole core is one of the most prevalent architectures found in a myriad of biologically active natural and synthesized compounds.1 For over 100 years, substituted indoles have remained preferred structures in the creation of new potential drugs, due to their high affinity to bind with many biological targets.2 Hence, enormous efforts have been devoted to the preparation and direct functionalization of indoles.3 However, the direct N-alkylation of indoles remains challenging due to the inertness of the indole nitrogen atom toward electrophilic reagents, despite the structural novelties and attractive biological activities of N-alkylated indoles. Some biologically active N-alkylated indole derivatives are shown in Fig. 1.
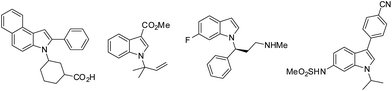 |
| Fig. 1 Representative biologically active N-alkylated indole derivatives. | |
One of the common methods for alkylation of indoles is a Mitsunobu reaction of indole derivatives with alcohol (Scheme 1).4 However, electron withdrawing substituents at position 2 or position 3 are needed to increase NH acidity in this reaction. Chen et al. reported the use of Morita–Baylis–Hillman carbonates in asymmetric N-allylic alkylation of indoles.5 Trost et al. and Hartwig et al. developed palladium-catalyzed and iridium-catalyzed N-allylic alkylation of indoles, respectively.6 Nevertheless, only N-allylation products were obtained through these methods. Therefore, it would be highly desirable to develop a new method for the mild and efficient direct introduction of alkyl substituents at the nitrogen atoms of indoles.
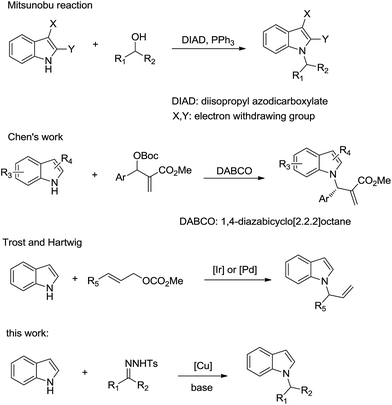 |
| Scheme 1 Synthesis of N-alkylated indoles. | |
On the other hand, N-tosylhydrazones, which are readily prepared from carbonyl compounds, have been used as a source for the safe generation of diazo compounds and carbenes.7 They have emerged as a new type of cross-coupling partner in C–C(X) bond formation, including in reactions with aryl halides,8 arylsulfonates,9 alkynes,10 H-phosphonates,11 azoles,12 arylboronic acids,13 benzylic halides,14 and amines.15
Herein, N-alkylated indoles were synthesized through the Cu-catalyzed reductive cross coupling of N-tosylhydrazones with indoles using potassium hydroxide as a base. Various N-branched alkylated and N-benzylated indoles were obtained through this method.
Results and discussion
We selected alkylation of indole with acetyl benzene-derived tosylhydrazone 1a as the model reaction for optimization (Table 1). Initial screening of Cu catalysts revealed that CuI provided the best activity, with 31% yield of 3a (Table 1, entry 7). Several phosphine ligands, including triphenylphosphine, tri(p-tolyl)phosphine, tris(4-methoxyphenyl)phosphine and tricyclohexylphosphine were examined. Tri(p-tolyl)phosphine and tris(4-methoxyphenyl)phosphine were shown to be the most efficient ligands in terms of reactivity, giving the product 3a in 30–31% yield (Table 1, entries 7 and 10). The best result was obtained with KOH as the base, giving 3a in 68% yield (Table 1, entry 12). Further screening of solvents did not improve the results (Table 1, entries 14–16). In the control experiment, 42% yield of product 3a was detected under conditions in which the phosphine ligand was absent (Table 1, entry 17). Thus, optimized reaction conditions were obtained as follows: 10 mol% of CuI, 10 mol% of tri(p-tolyl)phosphine, 2.5-equivalent of KOH, in dioxane at 100 °C under argon (Table 1, entry 12).
Table 1 Optimization of reaction conditionsa
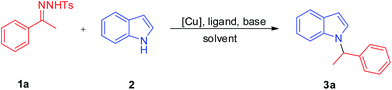
|
Entry |
Catalyst (10 mol%) |
Ligand (10 mol%) |
Solvent |
Base (2.5 equiv.) |
Yieldb (%) |
Reactions were carried out with 0.33 mmol of 1a and 0.50 mmol of 2 in the presence of 10 mol% of catalyst, 10 mol% of ligand, 2.5 equiv. of base and 2 mL solvent under an argon atmosphere for 12 h. Isolated yield. |
1 |
Cu(acac)2 |
P(p-tolyl)3 |
Dioxane |
Cs2CO3 |
Trace |
2 |
Cu(OTf)2 |
P(p-tolyl)3 |
Dioxane |
Cs2CO3 |
18 |
3 |
Cu(OAc)2 |
P(p-tolyl)3 |
Dioxane |
Cs2CO3 |
Trace |
4 |
CuBr |
P(p-tolyl)3 |
Dioxane |
Cs2CO3 |
20 |
5 |
CuCl |
P(p-tolyl)3 |
Dioxane |
Cs2CO3 |
19 |
6 |
Cu2O |
P(p-tolyl)3 |
Dioxane |
Cs2CO3 |
10 |
7 |
CuI |
P(p-tolyl)3 |
Dioxane |
Cs2CO3 |
31 |
8 |
CuI |
PPh3 |
Dioxane |
Cs2CO3 |
28 |
9 |
CuI |
P(p-OMePh)3 |
Dioxane |
Cs2CO3 |
30 |
10 |
CuI |
PCy3 |
Dioxane |
Cs2CO3 |
21 |
11 |
CuI |
P(p-tolyl)3 |
Dioxane |
LiOtBu |
25 |
12 |
CuI |
P(p-tolyl)3 |
Dioxane |
KOH |
68 |
13 |
CuI |
P(p-tolyl)3 |
Dioxane |
LiOH |
Trace |
14 |
CuI |
P(p-tolyl)3 |
Toulene |
KOH |
Trace |
15 |
CuI |
P(p-tolyl)3 |
CH3CN |
KOH |
Trace |
16 |
CuI |
P(p-tolyl)3 |
DMF |
KOH |
Trace |
17 |
CuI |
P(p-tolyl)3 |
Dioxane |
KOH |
42 |
Under the above optimized reaction conditions, we next examined the scope of this reaction (Table 2). N-Alkylation of indole proceeded with a wide range of N-tosylhydrazones in good yields. Electron-donating groups at the ortho, meta or para position on the phenyl ring of acetyl benzene-derived N-tosylhydrazones led to increased yields (3b to 3f), while strong electron withdrawing groups decreased the reaction efficiency to some extent (3j and 3k). Halogen groups and heteroaryl groups were well tolerated (3g to 3j, 3p), generating product in 58–80% yields. Benzophenone-derived N-tosylhydrazones with methyl or chloro substituents reacted with indoles to give N-alkylation products in good yield (3m to 3o). More importantly, alkyl ketone-derived N-tosylhydrazones could be effective alkylation substrates (3q to 3s). Alkylation of indoles with benzaldehyde-derived N-tosylhydrazone was examined (3t and 3u), giving products in 82–86% yield. Substituted indoles with an electron-donating group at the 5-position increased the product yield to 85% (3w) and electron withdrawing group at the 5-position caused a small decrease in production yield to 64% (3v). Finally, pyrrole and carbazole also reacted well with N-tosylhydrazones giving products (3x to 3z, 3aa) in 41–78% yield.
Table 2 Cu-catalyzed N-alkylation of indoles with tosylhydrazonesa,b
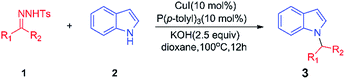
|
Reactions were carried out with 0.33 mmol of 1 and 0.50 mmol of 2 in the presence of 10 mol% of CuI, 10 mol% of P(p-tolyl)3, 0.83 mmol of KOH and 2 mL of dioxane at 100 °C under an argon atmosphere for 12 h. Isolated yield after chromatography. |
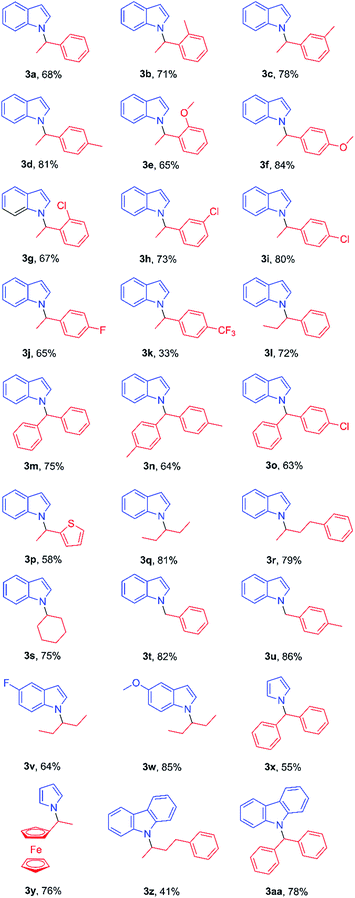 |
The synthetic value of our method was demonstrated by the synthesis of N-alkylated indole derivative 6, which has been shown to have activity in treatment of Alzheimer's disease (Scheme 2).16 The reaction of 2-phenylbenz[e]indole 4 with 3-(methoxycarbonyl)cyclohexanone-derived tosylhydrazone 5 under the current conditions proceeded smoothly to give N-alkylated product in 71% yield. Following hydrolysis of the ester group, the biologically active indole derivative 6 was obtained in 70% yield over two steps.
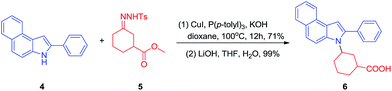 |
| Scheme 2 Application of current method to synthesis of biologically active N-alkylated indole derivative. | |
A plausible mechanism for this Cu-catalyzed reductive coupling is proposed on the basis of transition metal-catalyzed cross-coupling reactions of N-tosylhydrazones in the literature (Scheme 3).7b,17 First, the diazo compound B is formed by decomposition of the tosylhydrazone A in the presence of base. Subsequently, reaction of copper salt and diazo compound B leads to the copper-carbene species C. Then, deprotonation of indole in basic conditions followed by coordination with Cu(I) generates the intermediate D. Next, migratory insertion of the indole group gives Cu(I) species E. Finally, protonation of intermediate E affords the product and regenerates the Cu(I) catalyst.
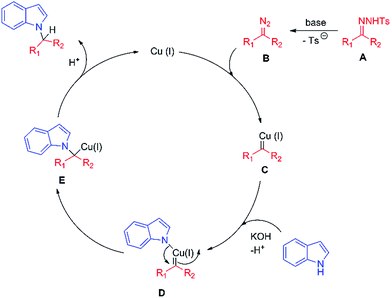 |
| Scheme 3 Proposed reaction mechanism. | |
Conclusions
In conclusion, we have described a new procedure for the direct N-alkylation of indoles by reductive coupling of N-tosylhydrazones with indoles in the presence of KOH and a catalytic amount of CuI and tri(p-tolyl)phosphine. Various N-branched alkylated and N-benzylated indoles could be obtained in moderate to good yield. Notably, the starting materials in this method are readily available, since N-tosylhydrazones are easily prepared from the corresponding aldehydes or ketones. Additionally, the reaction procedure has functional group compatibility and operational simplicity, and may have potential use in the synthesis of biologically important N-alkylated indoles.
Experimental section
General experimental methods
All reactions were carried out in oven dried glassware under argon unless otherwise specified. All NMR experiments were carried out on a Bruker AVANCE 500 MHz nuclear magnetic resonance spectrometer using CDCl3 or DMSO-d6 as the solvent with tetramethylsilane as the internal standard. Chemical shift values (δ) are given in parts per million. HRMS data were obtained on a Bruker QTOF mass spectrometer. The melting points were determined on an X-4 binocular microscope melting point apparatus and uncorrected. Dioxane, toluene, DMF and acetonitrile were dried according to literature techniques.
General procedure for preparation of N-alkylindoles
The indoles (0.50 mmol, 1.5 equiv.), N-tosylhydrazones (0.33 mmol, 1.0 equiv.), potassium hydroxide (0.83 mmol, 2.5 equiv.), copper iodide (0.03 mmol, 10 mol%), P(p-tolyl)3 (0.03 mmol, 10 mol%) and 2 mL dioxane were mixed in a dry reaction tube. The mixture was stirred at 100 °C under an argon atmosphere for 12 hours. When the reaction was completed, the crude reaction mixture was allowed to reach room temperature, and the solvent was eliminated. Then ethyl acetate and ammonia were added and the layers were separated. The aqueous phase was extracted three times with ethyl acetate. The combined organic layers were washed with water and then dried over MgSO4 and filtered. The solvents were evaporated under reduced pressure and the residue was purified by flash chromatography on silica gel.
1-(1-Phenylethyl)-1H-indole (3a)18. Yellow oil, 52.2 mg (68% yield); Rf (petroleum ether) = 0.6. 1H NMR (500 MHz, CDCl3) δ 7.71 (d, J = 7.3 Hz, 1H), 7.32 (d, J = 17.5, 11.8, 6.3 Hz, 5H), 7.25–7.13 (m, 4H), 6.65 (t, J = 4.3 Hz, 1H), 5.73 (d, J = 13.8, 6.8 Hz, 1H), 1.98 (t, J = 6.3 Hz, 3H). 13C NMR (126 MHz, CDCl3) δ 149.2, 148.5, 147.5, 139.1, 123.4, 115.8, 113.8, 69.7, 69.0, 66.9. HRMS (ESI-TOF) m/z: [M + H]+ calcd for C16H15N 222.1283; found: 222.1283.
1-(1-(o-Tolyl)ethyl)-1H-indole (3b). Yellow oil, 55.2 mg (71% yield); Rf (petroleum ether) = 0.6. 1H NMR (500 MHz, CDCl3) δ 7.62 (d, J = 7.8 Hz, 1H), 7.21–7.14 (m, 4H), 7.13–7.06 (m, 4H), 6.50 (d, J = 3.0 Hz, 1H), 5.78 (q, J = 6.9 Hz, 1H), 2.24 (s, 3H), 1.84 (d, J = 7.0 Hz, 3H). 13C NMR (126 MHz, CDCl3) δ 140.1, 136.0, 135.7, 130.8, 128.8, 127.7, 126.6, 125.4, 125.1, 121.5, 121.0, 119.6, 109.7, 101.3, 51.8, 20.5, 19.1. HRMS (ESI-TOF) m/z: [M]+ calcd for C17H17N 235.1361; found: 235.1356.
1-(1-(m-Tolyl)ethyl)-1H-indole (3c). Yellow oil, 60.5 mg (78% yield); Rf (petroleum ether) = 0.5. 1H NMR (500 MHz, CDCl3) δ 8.51 (d, J = 20.3 Hz, 2H), 8.24 (d, J = 8.2 Hz, 1H), 8.02–7.79 (m, 1H), 7.36 (d, J = 6.8, 5.2 Hz, 1H), 4.85 (s, 2H), 4.37 (s, 2H), 4.13 (s, 5H). 13C NMR (126 MHz, CDCl3) δ 142.7, 138.4, 136.2, 128.8, 128.6, 128.3, 126.7, 125.0, 123.1, 121.5, 120.9, 119.6, 110.1, 101.4, 54.8, 21.9, 21.6. HRMS (ESI-TOF) m/z: [M]+ calcd for C17H17N 235.1361; found: 235.1358.
1-(1-(p-Tolyl)ethyl)-1H-indole (3d). Yellow oil, 62.9 mg (81% yield); Rf (petroleum ether) = 0.5. 1H NMR (500 MHz, CDCl3) δ 8.51 (d, J = 20.3 Hz, 2H), 8.24 (d, J = 8.2 Hz, 1H), 8.02–7.79 (m, 1H), 7.36 (dd, J = 6.8, 5.2 Hz, 1H), 4.85 (s, 2H), 4.37 (s, 2H), 4.13 (s, 5H). 13C NMR (126 MHz, CDCl3) δ 139.7, 137.1, 136.1, 129.4, 128.8, 125.9, 124.9, 121.5, 120.9, 119.5, 110.1, 101.4, 54.6, 21.8, 21.1. HRMS (ESI-TOF) m/z: [M + H]+ calcd for C17H17N 236.1439; found: 236.1442.
1-(1-(2-Methoxyphenyl)ethyl)-1H-indole (3e). Yellow oil, 51.4 mg (65% yield); Rf (petroleum ether) = 0.4. 1H NMR (500 MHz, CDCl3) δ 7.61 (d, J = 7.7 Hz, 1H), 7.38 (d, J = 3.2 Hz, 1H), 7.22 (d, J = 8.0 Hz, 1H), 7.16 (s, 1H), 7.13–7.00 (m, 2H), 6.84 (d, J = 8.1 Hz, 1H), 6.76 (d, J = 6.6 Hz, 2H), 6.54 (d, J = 3.1 Hz, 1H), 6.03 (q, J = 7.0 Hz, 1H), 3.83 (s, 3H), 1.84 (d, J = 7.0 Hz, 3H). 13C NMR (126 MHz, CDCl3) δ 156.2, 136.1, 131.3, 128.7, 128.5, 126.0, 125.1, 121.3, 120.8, 119.4, 110.5, 110.3, 101.0, 55.5, 49.2, 20.5. HRMS (ESI-TOF) m/z: [M]+ calcd for C17H17NO 251.1310; found: 251.1305.
1-(1-(4-Methoxyphenyl)ethyl)-1H-indole (3f). Yellow oil, 64.1 mg (81% yield); Rf (petroleum ether) = 0.4. 1H NMR (500 MHz, CDCl3) δ 7.69 (d, J = 7.5 Hz, 1H), 7.30 (t, J = 6.4 Hz, 2H), 7.19 (t, J = 7.5 Hz, 1H), 7.13 (d, J = 15.2, 7.8 Hz, 3H), 6.86 (d, J = 8.7 Hz, 2H), 6.60 (d, J = 3.2 Hz, 1H), 5.68 (q, J = 7.0 Hz, 1H), 3.80 (s, 3H), 1.93 (d, J = 7.1 Hz, 3H). 13C NMR (126 MHz, CDCl3) δ 158.9, 136.0, 134.7, 128.8, 127.2, 124.9, 121.4, 120.9, 119.5, 114.0, 110.1, 101.4, 55.3, 54.3, 21.7. HRMS (ESI-TOF) m/z: [M]+ calcd for C17H17NO 251.1310; found: 251.1305.
1-(1-(2-Chlorophenyl)ethyl)-1H-indole (3g). Yellow oil, 49.8 mg (63% yield); Rf (petroleum ether) = 0.5. 1H NMR (500 MHz, CDCl3) δ 7.67–7.56 (m, 1H), 7.36 (dd, J = 18.9, 5.6 Hz, 2H), 7.16–7.05 (m, 4H), 7.02 (d, J = 14.8, 7.4 Hz, 1H), 6.74 (d, J = 7.8 Hz, 1H), 6.58 (d, J = 3.2 Hz, 1H), 6.02 (q, J = 7.0 Hz, 1H), 1.86 (d, J = 7.0 Hz, 3H). 13C NMR (126 MHz, CDCl3) δ 140.7, 136.1, 132.1, 129.7, 128.9, 128.7, 127.6, 126.5, 124.7, 121.7, 120.9, 119.8, 110.1, 101.6, 52.1, 20.5. HRMS (ESI-TOF) m/z: [M]+ calcd for C16H14NCl 255.0815; found: 255.0809.
1-(1-(3-Chlorophenyl)ethyl)-1H-indole (3h). Yellow oil, 57.7 mg (73% yield); Rf (petroleum ether) = 0.5. 1H NMR (500 MHz, CDCl3) δ 7.63 (d, J = 7.4 Hz, 1H), 7.17 (d, J = 9.1, 7.9 Hz, 3H), 7.12 (d, J = 25.6, 11.7, 4.2 Hz, 4H), 6.92 (d, J = 7.2, 1.1 Hz, 1H), 6.57 (s, 1H), 5.59 (t, J = 6.9 Hz, 1H), 1.86 (d, J = 7.1, 1.6 Hz, 3H). 13C NMR (126 MHz, CDCl3) δ 145.0, 136.0, 134.7, 130.1, 128.9, 127.7, 126.1, 124.7, 124.3, 121.1, 121.1, 120.0, 110.0, 102.0, 54.4, 21.8. HRMS (ESI-TOF) m/z: [M]+ calcd for C16H14NCl 255.0815; found: 255.0812.
1-(1-(4-Chlorophenyl)ethyl)-1H-indole (3i). Yellow oil, 65.7 mg (83% yield); Rf (petroleum ether) = 0.5. 1H NMR (500 MHz, CDCl3) δ 7.63 (d, J = 7.1 Hz, 1H), 7.23 (d, J = 15.1, 5.9 Hz, 3H), 7.19–7.04 (m, 3H), 7.01 (d, J = 8.4 Hz, 2H), 6.57 (d, J = 3.2 Hz, 1H), 5.60 (q, J = 7.1 Hz, 1H), 1.87 (d, J = 7.1 Hz, 3H). 13C NMR (126 MHz, CDCl3) δ 141.4, 136.0, 133.2, 128.9, 127.3, 124.7, 121.7, 121.1, 119.8, 110.0, 101.8, 54.3, 21.7. HRMS (ESI-TOF) m/z: [M]+ calcd for C16H14NCl 255.0815; found: 255.0809.
1-(1-(4-Fluorophenyl)ethyl)-1H-indole (3J). White solid, 61.7 mg (79% yield); mp 72–73 °C; Rf (petroleum ether) = 0.4. 1H NMR (500 MHz, CDCl3) δ 7.63 (d, J = 7.3 Hz, 1H), 7.22 (d, J = 3.0 Hz, 1H), 7.17 (d, J = 7.7 Hz, 1H), 7.14–7.06 (m, 2H), 7.03 (d, J = 8.3, 5.5 Hz, 2H), 6.95–6.88 (m, 2H), 6.55 (d, J = 3.1 Hz, 1H), 5.59 (q, J = 7.0 Hz, 1H), 1.84 (d, J = 7.1 Hz, 3H). 13C NMR (126 MHz, CDCl3) δ 163.0, 161.0, 138.5, 138.5, 136.0, 128.9, 127.6, 127.6, 124.7, 121.6, 121.0, 119.7, 115.7, 115.5, 110.0, 101.7, 54.2, 21.8. HRMS (ESI-TOF) m/z: [M]+ calcd for C16H14NF 239.1110; found: 239.1105.
1-(1-(4-(Trifluoromethyl)phenyl)ethyl)-1H-indole (3k). White oil, 26.7 mg (33% yield); Rf (petroleum ether) = 0.4. 1H NMR (500 MHz, CDCl3) δ 7.66 (d, J = 6.8, 2.0 Hz, 1H), 7.54 (d, J = 8.2 Hz, 2H), 7.32 (d, J = 3.3 Hz, 1H), 7.20 (d, J = 8.4 Hz, 2H), 7.18–7.09 (m, 3H), 6.62 (d, J = 3.2 Hz, 1H), 5.72 (q, J = 7.1 Hz, 1H), 1.96 (d, J = 7.1 Hz, 3H). 13C NMR (126 MHz, CDCl3) δ 136.0, 128.9, 126.2, 125.8, 124.6, 121.8, 121.2, 119.9, 110.0, 102.1, 100.1, 54.6, 21.8. HRMS (ESI-TOF) m/z: [M]+ calcd for C17H14NF3 239.1110; found: 239.1105.
1-(1-Phenylpropyl)-1H-indole (3l). Yellow oil, 63.7 mg (82% yield); Rf (petroleum ether) = 0.6. 1H NMR (500 MHz, CDCl3) δ 7.62 (d, J = 7.8 Hz, 1H), 7.29 (d, J = 3.1 Hz, 2H), 7.25–7.10 (m, 6H), 7.07 (t, J = 7.6 Hz, 1H), 6.56 (d, J = 3.2 Hz, 1H), 5.32 (d, J = 8.7, 6.7 Hz, 1H), 2.36–2.24 (m, 2H), 0.94 (t, J = 7.3 Hz, 3H). 13C NMR (126 MHz, CDCl3) δ 141.7, 136.5, 128.7, 128.7, 127.5, 126.5, 125.0, 121.5, 121.0, 119.5, 110.0, 101.7, 61.4, 28.5, 11.6. HRMS (ESI-TOF) m/z: [M]+ calcd for C17H17N 235.1361; found: 235.1362.
1-(Diphenylmethyl)-1H-indole (3m)19. White solid, 60.7 mg (75% yield); mp 91–92 °C; Rf (petroleum ether) = 0.5. 1H NMR (500 MHz, CDCl3) δ 7.63 (d, J = 5.8, 2.9 Hz, 1H), 7.35–7.16 (m, 7H), 7.11 (d, J = 14.5, 11.7, 7.9 Hz, 7H), 6.85–6.78 (m, 2H), 6.48 (d, J = 3.1 Hz, 1H). 13C NMR (126 MHz, CDCl3) δ 139.9, 136.6, 129.0, 128.7, 128.6, 128.5, 128.2, 128.0, 127.0, 125.9, 121.7, 121.0, 119.9, 110.3, 101.5, 63.7. HRMS (ESI-TOF) m/z: [M]+ calcd for C21H17N 283.1361; found: 283.1356.
1-(Di-p-tolylmethyl)-1H-indole (3n). White solid, 69.0 mg (84% yield); mp 111–113 °C; Rf (petroleum ether) = 0.6. 1H NMR (500 MHz, CDCl3) δ 7.72 (d, J = 6.2, 2.4 Hz, 1H), 7.31 (d, J = 7.2 Hz, 1H), 7.18 (t, J = 6.3 Hz, 6H), 7.06 (d, J = 8.0 Hz, 4H), 6.92 (d, J = 3.3 Hz, 1H), 6.84 (s, 1H), 6.56 (d, J = 3.1 Hz, 1H), 2.40 (s, 6H). 13C NMR (126 MHz, CDCl3) δ 137.6, 137.2, 136.6, 129.4, 129.0, 128.3, 127.0, 121.5, 120.9, 119.7, 110.3, 101.2, 63.2, 21.2. HRMS (ESI-TOF) m/z: [M]+ calcd for C23H21N 311.1674; found: 311.1669.
1-((4-Chlorophenyl) (phenyl)methyl)-1H-indole (3o). Yellow oil, 68.4 mg (83% yield); Rf (petroleum ether) = 0.5. 1H NMR (500 MHz, CDCl3) δ 7.77–7.70 (m, 1H), 7.40 (d, J = 6.2 Hz, 3H), 7.36 (d, J = 8.3 Hz, 2H), 7.29 (d, J = 6.4 Hz, 1H), 7.22 (d, J = 9.4, 5.7 Hz, 2H), 7.19–7.15 (m, 2H), 7.08 (d, J = 8.4 Hz, 2H), 6.91–6.84 (m, 2H), 6.60 (d, J = 3.1 Hz, 1H). 13C NMR (126 MHz, CDCl3) δ 139.3, 138.5, 136.5, 133.8, 129.7, 129.0, 128.9, 128.9, 128.5, 128.2, 126.7, 121.8, 121.1, 120.0, 110.1, 101.8, 63.0. HRMS (ESI-TOF) m/z: [M]+ calcd for C21H16NCl 317.0971; found: 317.0970.
1-(1-(Thiophen-2-yl)ethyl)-1H-indole (3p)20. Yellow oil, 44.8 mg (58% yield); Rf (petroleum ether) = 0.5. 1H NMR (500 MHz, CDCl3) δ 7.66 (d, J = 7.9 Hz, 1H), 7.40 (d, J = 8.3 Hz, 1H), 7.22 (t, J = 3.7 Hz, 3H), 7.14 (t, J = 7.4 Hz, 1H), 6.98–6.86 (m, 2H), 6.57 (d, J = 3.2 Hz, 1H), 5.93 (q, J = 7.0 Hz, 1H), 1.99 (d, J = 7.0 Hz, 3H). 13C NMR (126 MHz, CDCl3) δ 146.1, 135.8, 128.8, 126.8, 124.8, 124.5, 121.6, 121.2, 119.7, 109.7, 102.1, 50.8, 22.3. HRMS (ESI-TOF) m/z: [M]+ calcd for C14H13NS 227.0769; found: 227.0763.
1-(Pentan-3-yl)-1H-indole (3q). Yellow oil, 59.5 mg (81% yield); Rf (petroleum ether) = 0.6. 1H NMR (500 MHz, CDCl3) δ 7.63 (d, J = 7.8 Hz, 1H), 7.37 (d, J = 8.3 Hz, 1H), 7.18 (d, J = 13.7, 5.6 Hz, 1H), 7.12 (d, J = 3.2 Hz, 1H), 7.08 (t, J = 7.1 Hz, 1H), 6.54 (d, J = 3.0 Hz, 1H), 4.17–4.04 (m, 1H), 1.91–1.82 (m, 4H), 0.75 (t, J = 7.4 Hz, 6H). 13C NMR (126 MHz, CDCl3) δ 136.9, 128.4, 124.4, 121.1, 120.9, 119.1, 109.7, 101.4, 59.7, 29.8, 28.6, 11.0. HRMS (ESI-TOF) m/z: [M]+ calcd for C13H17N 187.1361; found: 187.1356.
1-(4-Phenylbutan-2-yl)-1H-indole (3r). Yellow oil, 38.5 mg (49% yield); Rf (petroleum ether) = 0.5. 1H NMR (500 MHz, CDCl3) δ 7.64 (d, J = 7.8 Hz, 1H), 7.30–7.25 (m, 2H), 7.20 (d, J = 12.6, 5.2 Hz, 3H), 7.08 (t, J = 7.0 Hz, 3H), 6.56 (d, J = 2.8 Hz, 1H), 4.48 (d, J = 14.3, 6.8 Hz, 1H), 2.50 (d, J = 8.2 Hz, 2H), 2.32–2.22 (m, 1H), 2.19–2.08 (m, 1H), 1.51 (d, J = 6.8 Hz, 3H). 13C NMR (126 MHz, CDCl3) δ 141.3, 136.0, 128.4, 126.1, 124.0, 121.3, 121.0, 119.3, 109.6, 101.8, 509, 38.7, 32.6, 21.5. HRMS (ESI-TOF) m/z: [M + H]+ calcd for C18H19N 250.1596; found: 250.1570.
1-Cyclohexyl-1H-indole (3s). Yellow oil, 27.6 mg (37% yield); Rf (petroleum ether) = 0.7. 1H NMR (500 MHz, CDCl3) δ 7.61 (t, J = 6.7 Hz, 1H), 7.36 (d, J = 8.3 Hz, 1H), 7.17 (d, J = 8.0, 5.3 Hz, 2H), 7.11–7.04 (m, 1H), 6.49 (d, J = 3.2 Hz, 1H), 4.18 (t, J = 11.9, 3.7 Hz, 1H), 2.12 (d, J = 1.7 Hz, 2H), 1.90 (d, J = 13.6 Hz, 2H), 1.70–1.61 (m, 2H), 1.47 (t, J = 13.2, 6.6 Hz, 2H), 1.35–1.16 (m, 2H). 13C NMR (126 MHz, CDCl3) δ 135.9, 135.6, 128.8, 128.5, 126.7, 124.1, 121.6, 121.0, 119.7, 119.2, 111.1, 109.5, 102.0, 101.0, 55.1, 33.6, 29.8, 28.9, 26.0, 25.7, 24.6, 23.0, 22.0. HRMS (ESI-TOF) m/z: [M]+ calcd for C14H17N 199.1361; found: 199.1356.
1-Benzyl-1H-indole (3t)21. Yellow oil, 61.9 mg (82% yield); Rf (petroleum ether) = 0.4. 1H NMR (500 MHz, CDCl3) δ 7.68 (d, J = 7.8 Hz, 1H), 7.34–7.27 (m, 4H), 7.22–7.17 (m, 1H), 7.17–7.12 (m, 4H), 6.58 (d, J = 3.1, 0.6 Hz, 1H), 5.35 (s, 2H). 13C NMR (126 MHz, CDCl3) δ 137.6, 136.4, 128.8, 128.8, 128.3, 127.7, 126.8, 121.8, 121.0, 119.6, 109.8, 101.8, 50.1. HRMS (ESI-TOF) m/z: [M]+ calcd for C15H13N 207.1048; found: 207.1043.
1-(4-Methylbenzyl)-1H-indole (3u)22. Yellow oil, 65.9 mg (86% yield); Rf (petroleum ether) = 0.4. 1H NMR (500 MHz, CDCl3) δ 7.63 (d, J = 7.8 Hz, 1H), 7.18–6.96 (m, 8H), 6.52 (d, J = 3.1 Hz, 1H), 5.23 (s, 2H), 2.28 (s, 3H). 13C NMR (126 MHz, CDCl3) δ 137.3, 136.3, 134.5, 129.5, 128.8, 128.3, 126.9, 121.7, 121.0, 119.5, 109.8, 101.6, 49.9, 21.1. HRMS(ESI-TOF) m/z: [M]+ calcd for C15H13N 221.1204; found: 221.1199.
5-Fluoro-1-(pentan-3-yl)-1H-indole (3v). Yellow oil, 43.4 mg (64% yield); Rf (petroleum ether) = 0.4. 1H NMR (500 MHz, CDCl3) δ 7.30–7.26 (m, 1H), 7.25–7.23 (m, 1H), 7.22–7.20 (m, 1H), 6.96–6.90 (m, 1H), 6.47 (d, J = 3.1 Hz, 1H), 4.59–4.32 (m, 1H), 1.93–1.70 (m, 4H), 0.88 (t, J = 7.4 Hz, 6H). 13C NMR (126 MHz, CDCl3) δ 157.8 (d, J = 233.6 Hz), 132.7, 128.6 (d, J = 10.1 Hz), 125.7, 110.1 (d, J = 9.7 Hz), 109.7 (d, J = 26.3 Hz), 105.6 (d, J = 23.1 Hz), 101.4 (d, J = 4.7 Hz), 51.8, 39.5, 14.0. HRMS (ESI-TOF) m/z: [M + Na]+ calcd for C13H16FN 228.1164; found: 228.1165.
5-Methoxy-1-(pentan-3-yl)-1H-indole (3w). Colorless oil, 61.0 mg (85% yield); Rf (petroleum ether) = 0.4. 1H NMR (500 MHz, CDCl3) δ 7.28–7.25 (m, 1H), 7.15 (d, J = 3.1 Hz, 1H), 7.09 (d, J = 2.4 Hz, 1H), 6.88–6.83 (m, 1H), 6.44 (d, J = 3.1 Hz, 1H), 4.49–4.33 (m, 1H), 3.85 (s, 3H), 2.02–1.62 (m, 4H), 0.87 (t, J = 7.4 Hz, 6H). 13C NMR (126 MHz, CDCl3) δ 153.9, 131.4, 128.8, 124.7, 111.7, 110.3, 102.6, 100.9, 56.0, 51.6, 29.9, 14.0. HRMS (ESI-TOF) m/z: [M + H]+ calcd for C14H19NO 218.1545; found: 218.1551.
1-Benzhydryl-1H-pyrrole (3x). Colorless oil, 42.3 mg (55% yield); Rf (petroleum ether) = 0.5. 1H NMR (500 MHz, CDCl3) δ 7.35–6.99 (m, 10H), 6.76 (t, J = 2.1 Hz, 2H), 6.19 (t, J = 2.1 Hz, 2H), 5.33–5.24 (m, 1H). 13C NMR (126 MHz, CDCl3) δ 143.7, 133.4, 129.6, 128.8, 128.5, 127.6, 127.5, 127.2, 126.0, 119.7, 108.2, 58.3. HRMS (ESI-TOF) m/z: [M + H]+ calcd for C17H15N 234.1283; found: 234.1281.
1-(1-Ferrocenylethyl)-1H-pyrrole (3y). Yellow oil, 70.0 mg (76% yield); Rf (petroleum ether) = 0.5. 1H NMR (500 MHz, CDCl3) δ 6.76–6.60 (m, 2H), 6.20–5.96 (m, 2H), 5.07 (d, J = 7.0 Hz, 1H), 4.20–4.18 (m, 1H), 4.17–4.10 (m, 7H), 4.10–4.06 (m, 1H), 1.78 (d, J = 7.0 Hz, 3H). 13C NMR (126 MHz, CDCl3) δ 119.1, 107.6, 90.8, 69.0, 68.3, 67.8, 67.8, 66.2, 54.5, 22.5. HRMS (ESI-TOF) m/z: [M]+ calcd for C16H17FeN 279.0710; found: 279.0723.
9-(4-Phenylbutan-2-yl)-9H-carbazole (3z). Yellow oil, 38.7 mg (41% yield); Rf (petroleum ether
:
ethyl acetate = 100
:
1) = 0.4. 1H NMR (500 MHz, CDCl3) δ 7.68 (d, J = 7.8 Hz, 1H), 7.30 (d, J = 8.0 Hz, 3H), 7.22 (d, J = 8.5 Hz, 3H), 7.12 (t, J = 7.7 Hz, 3H), 6.59 (d, J = 2.9 Hz, 1H), 4.51 (d, J = 6.8 Hz, 1H), 2.54 (d, J = 5.9 Hz, 2H), 2.30 (d, J = 6.2 Hz, 1H), 2.16 (d, J = 6.0 Hz, 1H), 1.54 (d, J = 6.8 Hz, 3H). 13C NMR (126 MHz, CDCl3) δ 141.2, 128.5, 126.0, 125.5, 120.4, 118.7, 50.6, 36.6, 33.0, 19.3. HRMS (ESI-TOF) m/z: [M]+ calcd for C22H21N 299.1674; found: 299.1670.
9-Benzhydryl-9H-carbazole (3aa). White solid, 74.1 mg (78% yield); mp 170–173 °C; Rf (petroleum ether
:
ethyl acetate = 100
:
1) = 0.6. 1H NMR (500 MHz, CDCl3) δ 8.22 (d, J = 7.6 Hz, 2H), 7.38 (d, J = 3.7 Hz, 7H), 7.36–7.27 (m, 8H), 7.18 (d, J = 8.2 Hz, 2H). 13C NMR (126 MHz, CDCl3) δ 140.7, 138.9, 128.7, 128.4, 127.9, 125.6, 123.6, 120.2, 119.3, 110.8, 62.3. HRMS (ESI-TOF) m/z: [M + Na]+ calcd for C25H19N 356.1415; found: 356.1406.
3-(2-Phenyl-3H-benzo[e]indol-3-yl)cyclohexanecarboxylic acid (6)16. White oil, 85.3 mg (70% yield); Rf (petroleum ether) = 0.7. 1H NMR (500 MHz, CDCl3) δ 8.30–8.25 (m, 1H), 8.10–8.05 (m, 2H), 7.84–7.88 (m, 2H), 7.55–7.44 (m, 6H), 7.02–6.98 (m, 1H), 4.54–4.31 (m, 1H), 2.94–1.51 (m, 9H). 13C NMR (126 MHz, CDCl3) δ 185.1, 138.9, 136.0, 133.1, 131.3, 130.9, 128.5, 128.2, 127.5, 126.5, 126.4, 126.1, 124.9, 120.5, 110.7, 95.0, 43.1, 38.0, 32.5, 25.8, 20.3. HRMS (ESI-TOF) m/z: [M + H]+ calcd for C25H23NO2 370.1729; found: 370.1739.
Acknowledgements
This work was supported by the National Natural Science Foundation of China (Grant No. 21364005), Natural Science Foundation of Inner Mongolia (2017MS0206) and Grassland Talent Innovative Teams of Inner Mongolia Autonomous Region. The project was supported by Open Research Fund of State Key Laboratory of Polymer Physics and Chemistry, Changchun Institute of Applied Chemistry, Chinese Academy of Sciences.
Notes and references
-
(a) W. Gul and M. T. Hamann, Life Sci., 2005, 78, 442–453 CrossRef CAS PubMed;
(b) M. Ishikura and K. Yamada, Nat. Prod. Rep., 2009, 26, 803–852 RSC;
(c) M. Somei and F. Yamada, Nat. Prod. Rep., 2005, 22, 73–103 RSC.
-
(a) B. Evans, K. Rittle, M. Bock, R. DiPardo, R. Freidinger, W. Whitter, G. Lundell, D. Veber, P. Anderson and R. Chang, J. Med. Chem., 1988, 31, 2235–2246 CrossRef CAS PubMed;
(b) D. A. Horton, G. T. Bourne and M. L. Smythe, Chem. Rev., 2003, 103, 893–930 CrossRef CAS PubMed;
(c) W. J. Houlihan, The Chemistry of Heterocyclic Compounds, Indoles, John Wiley & Sons, 2009 Search PubMed;
(d) R. J. Sundberg, Indoles, Academic Press, 1996 Search PubMed.
-
(a) M. Bandini and A. Eichholzer, Angew. Chem., Int. Ed., 2009, 48, 9608–9644 CrossRef CAS PubMed;
(b) S. Cacchi and G. Fabrizi, Chem. Rev., 2005, 105, 2873–2920 CrossRef CAS PubMed;
(c) G. R. Humphrey and J. T. Kuethe, Chem. Rev., 2006, 106, 2875–2911 CrossRef CAS PubMed;
(d) J. A. Joule, in Science of Synthesis (Houben-Weyl Methods of Molecular Transformations), ed. E. J. Thomas, Thieme Verlag, Stuttgart, 2000, vol. 10, ch. 10, p. 13 Search PubMed;
(e) N. T. Patil and Y. Yamamoto, Chem. Rev., 2008, 108, 3395–3442 CrossRef CAS PubMed;
(f) G. Zeni and R. C. Larock, Chem. Rev., 2004, 104, 2285–2310 CrossRef CAS PubMed.
-
(a) S. S. Bhagwat and C. Gude, Tetrahedron Lett., 1994, 35, 1847–1850 CrossRef CAS;
(b) A. Bombrun and G. Casi, Tetrahedron Lett., 2002, 43, 2187–2190 CrossRef CAS;
(c) W. Fröhner, B. Monse, T. M. Braxmeier, L. Casiraghi, H. Sahagún and P. Seneci, Org. Lett., 2005, 7, 4573–4576 CrossRef PubMed;
(d) J. K. Laha and G. D. Cuny, J. Org. Chem., 2011, 76, 8477–8482 CrossRef CAS PubMed;
(e) S. Messaoudi, F. Anizon, B. Pfeiffer and M. Prudhomme, Tetrahedron, 2005, 61, 7304–7316 CrossRef CAS;
(f) K. K. Swamy, N. B. Kumar, E. Balaraman and K. P. Kumar, Chem. Rev., 2009, 109, 2551–2651 CrossRef CAS PubMed;
(g) G. Yagil, Tetrahedron, 1967, 23, 2855–2861 CrossRef CAS PubMed.
- H. L. Cui, X. Feng, J. Peng, J. Lei, K. Jiang and Y. C. Chen, Angew. Chem., Int. Ed., 2009, 48, 5737–5740 CrossRef CAS PubMed.
-
(a) L. M. Stanley and J. F. Hartwig, Angew. Chem., 2009, 121, 7981–7984 CrossRef;
(b) B. M. Trost, M. J. Krische, V. Berl and E. M. Grenzer, Org. Lett., 2002, 4, 2005–2008 CrossRef CAS PubMed.
-
(a) J. Barluenga and C. Valdés, Angew. Chem., Int. Ed., 2011, 50, 7486–7500 CrossRef CAS PubMed;
(b) Q. Xiao, Y. Zhang and J. Wang, Acc. Chem. Res., 2012, 46, 236–247 CrossRef PubMed.
-
(a) J. Barluenga, P. Moriel, C. Valdés and F. Aznar, Angew. Chem., Int. Ed., 2007, 46, 5587–5590 CrossRef CAS PubMed;
(b) E. Brachet, A. Hamze, J.-F. Peyrat, J.-D. Brion and M. Alami, Org. Lett., 2010, 12, 4042–4045 CrossRef CAS PubMed;
(c) M. Roche, A. Hamze, J.-D. Brion and M. Alami, Org. Lett., 2012, 15, 148–151 CrossRef PubMed;
(d) M. Roche, A. Hamze, O. Provot, J.-D. Brion and M. Alami, J. Org. Chem., 2013, 78, 445–454 CrossRef CAS PubMed;
(e) Y. Xia, F. Hu, Z. Liu, P. Qu, R. Ge, C. Ma, Y. Zhang and J. Wang, Org. Lett., 2013, 15, 1784–1787 CrossRef CAS PubMed;
(f) L. Zhou, F. Ye, Y. Zhang and J. Wang, J. Am. Chem. Soc., 2010, 132, 13590–13591 CrossRef CAS PubMed.
-
(a) J. Barluenga, L. Florentino, F. Aznar and C. Valdés, Org. Lett., 2010, 13, 510–513 CrossRef PubMed;
(b) J. Hoegermeier and H. U. Reissig, Adv. Synth. Catal., 2009, 351, 2747–2763 CrossRef CAS;
(c) B. Tréguier, A. Hamze, O. Provot, J.-D. Brion and M. Alami, Tetrahedron Lett., 2009, 50, 6549–6552 CrossRef.
-
(a) Z. S. Chen, X. H. Duan, L. Y. Wu, S. Ali, K. G. Ji, P. X. Zhou, X. Y. Liu and Y. M. Liang, Chem.–Eur. J., 2011, 17, 6918–6921 CrossRef CAS PubMed;
(b) Q. Xiao, Y. Xia, H. Li, Y. Zhang and J. Wang, Angew. Chem., 2011, 123, 1146–1149 CrossRef;
(c) L. Zhou, Y. Shi, Q. Xiao, Y. Liu, F. Ye, Y. Zhang and J. Wang, Org. Lett., 2011, 13, 968–971 CrossRef CAS PubMed;
(d) L. Zhou, F. Ye, J. Ma, Y. Zhang and J. Wang, Angew. Chem., 2011, 123, 3572–3576 CrossRef.
- W. Miao, Y. Gao, X. Li, Y. Gao, G. Tang and Y. Zhao, Adv. Synth. Catal., 2012, 354, 2659–2664 CrossRef CAS.
- X. Zhao, G. Wu, Y. Zhang and J. Wang, J. Am. Chem. Soc., 2011, 133, 3296–3299 CrossRef CAS PubMed.
-
(a) J. Barluenga, M. Tomás-Gamasa, F. Aznar and C. Valdés, Nat. Chem., 2009, 1, 494–499 CrossRef CAS PubMed;
(b) X. Li, Y. Feng, L. Lin and G. Zou, J. Org. Chem., 2012, 77, 10991–10995 CrossRef CAS PubMed;
(c) M. C. Pérez-Aguilar and C. Valdés, Angew. Chem., Int. Ed., 2012, 51, 5953–5957 CrossRef PubMed;
(d) X. Zhao, J. Jing, K. Lu, Y. Zhang and J. Wang, Chem. Commun., 2010, 46, 1724–1726 RSC.
-
(a) Q. Xiao, J. Ma, Y. Yang, Y. Zhang and J. Wang, Org. Lett., 2009, 11, 4732–4735 CrossRef CAS PubMed;
(b) W.-Y. Yu, Y.-T. Tsoi, Z. Zhou and A. S. Chan, Org. Lett., 2008, 11, 469–472 CrossRef PubMed.
- A. Hamze, B. Tréguier, J.-D. Brion and M. Alami, Org. Biomol. Chem., 2011, 9, 6200–6204 CAS.
- R. Slade, Y. Klimova, R. J. Halter, A. J. Yungai, W. S. Weiner, R. J. Walton, J. A. Willardsen, M. B. Anderson and K. Zavitz, US Pat., 7,678,823, 2010.
- A. P. Jadhav, D. Ray, V. Rao and R. P. Singh, Eur. J. Org. Chem., 2016, 2016, 2369–2382 CrossRef CAS.
- A. Karchava, I. Shuleva, A. Ovcharenko and M. Yurovskaya, Chem. Heterocycl. Compd., 2010, 46, 291–301 CrossRef CAS.
- S. M. Wales, M. M. Walker and J. S. Johnson, Org. Lett., 2013, 15, 2558–2561 CrossRef CAS PubMed.
- O. Aydin, H. Kilic, S. Bayindir, E. Erdogan and N. Saracoglu, J. Heterocycl. Chem., 2015, 52, 1540–1553 CrossRef CAS.
- P. Y. Choy, C. P. Lau and F. Y. Kwong, J. Org. Chem., 2010, 76, 80–84 CrossRef PubMed.
- H. Mao, S. Wang, P. Yu, H. Lv, R. Xu and Y. Pan, J. Org. Chem., 2011, 76, 1167–1169 CrossRef CAS PubMed.
Footnote |
† Electronic supplementary information (ESI) available: Spectroscopic data for all compounds. See DOI: 10.1039/c7ra03765a |
|
This journal is © The Royal Society of Chemistry 2017 |