DOI:
10.1039/C7OB02795H
(Communication)
Org. Biomol. Chem., 2017,
15, 10164-10166
Hydrogen-free reductive amination using iron pentacarbonyl as a reducing agent†
Received
15th November 2017
, Accepted 23rd November 2017
First published on 23rd November 2017
Abstract
We developed solvent-free reductive amination without an external hydrogen source using iron pentacarbonyl as a reducing agent. Neither a catalyst nor any other additives were employed. Various types of substrates are suitable for the reaction, including those with low reactivity, e.g. benzophenone. Among others, the protocol tolerates bromo-, cyano-, benzyloxy-, pyrimidyl and styryl moieties.
Selective and step-economical syntheses of complex molecules from starting materials easily available in bulk quantities are highly important from economic and environmental points of view. Such protocols should naturally tolerate a variety of functional groups, so that extra steps of introduction and removal of protective groups can be avoided. Recently, we have developed a series of methods for hydrogen-free reductive addition using carbon monoxide1 as a reducing agent.2 Starting from aldehydes or ketones, this approach can lead to various classes of compounds, including amines,2b amides, esters,2e,f pyrrolidines,2a and nitriles.2f These transformations are usually highly selective and tolerate the presence of aromatic and aliphatic nitrile groups,2f esters,2c phenols,2b carboxybenzyl (Cbz),2b trifluoroacetamido,2bN-benzyl,2aO-benzyl,2d aromatic fluoro-,2f chloro-2d and bromo-moieties,2a cyclopropanes, and even aromatic nitro-groups.2b Selectivities of these reductive aminations usually appreciably exceed those of standard reductive agents, such as sodium cyanoborohydride.2b The natural limitation for the employment of these methods in a laboratory is the necessity to use a carbon monoxide cylinder. In this context, we sought to develop a homogeneous alternative with CO in a chemically bound form, e.g. using a metal carbonyl complex as a reductant.3 From the points of view of both safety and economy, iron pentacarbonyl seemed to be the best choice since elemental impurities of iron in pharmaceutical products are much less stringently regulated by the corresponding authorities (e.g. FDA) than most other d-metals due to lower intrinsic toxicity of iron.5 Moreover production of iron pentacarbonyl exceeds thousands of tons per year and it is the least expensive metal carbonyl available.6 Iron tetracarbonyl dihydride is a well-known reducing agent; however, it rapidly decomposes at temperatures above −20 °C.7 The sodium salt is more stable, but is not commercially available and requires highly basic conditions for the synthesis, which can lead to a narrower substrate scope.4 Herein, we describe the protocol for reductive amination which employs nothing but starting materials and iron pentacarbonyl. No solvent or external hydrogen source is needed.
We chose morpholine and p-tolylaldehyde as model substrates. In light of the fact that iron pentacarbonyl is a liquid, improvement of the environmental profile of the reaction by the use of solvent-free conditions seemed very interesting. Thus, we heated the reaction components at 90 °C for 4 hours and did detect formation of product 1a (Table 1, entry 1). The temperature influence was found to be important in the range up to 130 °C (Table 1, entries 1–3); at higher temperatures no significant yield variability was found (Table 1, entries 3–6). When the amount of the carbonyl was decreased from three to two equivalents, no significant changes were observed (Table 1, entry 3 vs. 8). If the amount of the carbonyl was further decreased, the yield significantly dropped (Table 1, entry 1 vs. 9–11). When the amount of the starting amine (see ESI†) was increased to three equivalents, the yield increased up to 86% with reaction time of four hours. Based on the works of Hieber the reaction between amines and iron pentacarbonyl gives complicated clusters,8 so the mechanism reductive amination with iron pentacarbonyl seems to be complicated. The molybdenum hexacarbonyl showed the potential in the reductive amination as well (Table 1, entry 3 vs. 12).
Table 1 Investigation of the effects of the amount of iron pentacarbonyl and temperature on reductive amination

|
Entrya |
Fe(CO)5, eq. |
T, °C |
Yieldb % |
0.2 mmol scale. 1.5 eq. of morpholine were used.
Yields were determined by GC. Tol = p-methylphenyl.
Mo(CO)6 was used instead of Fe(CO)5.
|
1 |
3 |
90 |
14 |
2 |
3 |
110 |
26 |
3 |
3 |
130 |
41 |
4 |
3 |
150 |
40 |
5 |
3 |
160 |
41 |
6 |
3 |
180 |
44 |
7 |
5 |
130 |
51 |
8 |
2 |
130 |
44 |
9 |
1 |
130 |
24 |
10 |
0.5 |
130 |
15 |
11 |
0.2 |
130 |
11 |
12c |
3 |
130 |
25 |
With these results in hand, we proceeded to investigation of the substrate scope of the developed methodology under the optimized reaction conditions (Fig. 1).
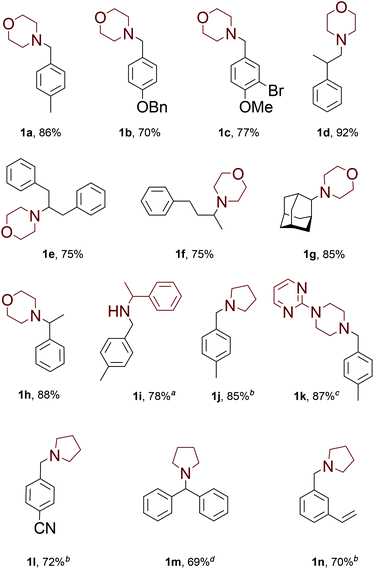 |
| Fig. 1 Studies on the substrate scope. Solvent-free conditions. 3 equivalents of Fe(CO)5 and amine were employed. 4 h at 130 °C. a 2 equivalents of amine were employed. 140 °C. b Room temperature. 12 hours. c 140 °C. d 90 °C. 12 hours. | |
A wide range of aldehydes could be successfully employed, including those containing various functional groups prone to reduction by dihydrogen. For example, benzyloxy moiety (1b), aromatic bromides (1c) and aromatic nitriles (1l) can be tolerated. Aliphatic aldehydes are known to undergo self-aldol reaction catalyzed by amines (especially secondary aliphatic amines);9 however, under our reaction conditions reductive amination product 1d was isolated in excellent yield. Whereas ketones are usually much less reactive in reductive amination, our methodology worked well with aliphatic ketones 1e–f. Acetophenone could be converted into product 1h with isolated yield of 88%. Moreover, even as unreactive ketone as 2-adamantanone 1g successfully furnished the product in 85% yield. Besides morpholine, other type of tested amines underwent the desired transformation 1i–k in high yields. The optimum reaction temperature was clearly dependent on the nucleophilicity of the starting amine. For pyrrolidine the reaction proceed well even at room temperature (1j, 1l, 1n). Other type of amines also could react at room temperature, albeit with lower rate. We were surprised to found that even benzophenone could be successfully employed: after 12 hours at 90 °C product 1m was isolated in 69% yield. The protocol is so mild that we isolated compound 1n without any side reactions on the styryl moiety.
The scalability and preparative utility of the developed methodology was exemplified on the synthesis of N-adamantylpyrrolidine. Despite the much lower intrinsic reactivity of 2-adamantanone with respect to less sterically challenged substrates, a gram-scale reaction with pyrrolidine proceeded well and furnished product 1n in 89% yield without the need for any chromatographic isolation (Scheme 1).
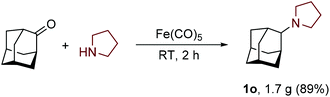 |
| Scheme 1 Scaled-up synthesis of N-adamantyl-pyrrolidine at room temperature. | |
In conclusion, we have shown that the concept of hydrogen-free reductive amination can be expanded to metal carbonyls as reducing agents, which can lead to the more selective approaches in organic synthesis. The synthetic value of the developed methodology was demonstrated by efficient preparation of a representative range of amines including clean gram-scale synthesis not requiring chromatographic purification. The reaction proceeds well even in case of poorly reactive ketones such as benzophenone.
Conflicts of interest
There are no conflicts of interest to declare.
Acknowledgements
We gratefully thank Professor Terent'ev A. O. and Dr Vil’ V. for the inspiring us to work with iron carbonyl. This work was supported by the Russian Foundation for Basic Research (15-03-02548). D. C. thanks the Ministry of Education and Science of the Russian Federation (Agreement number 02.a03.21.0008). The contribution of Center for molecule composition studies of INEOS RAS is gratefully acknowledged.
Notes and references
- For the recent examples of the state-of-the art applications of carbon monoxide as a reducing agent see:
(a) S. E. Denmark, M. Y. S. Ibrahim and A. Ambrosi, ACS Catal., 2017, 7(1), 613 CrossRef CAS;
(b) M. A. El-Atawy, F. Ferretti and F. Ragaini, Eur. J. Org. Chem., 2017,(14), 1902 CrossRef CAS;
(c) M.-M. Zhu, L. Tao, Q. Zhang, J. Dong, Y.-M. Liu, H.-Y. He and Y. Cao, Green Chem., 2017, 19(16), 3880 RSC;
(d) A. Ambrosi and S. E. Denmark, Angew. Chem., Int. Ed., 2016, 55(40), 12164 CrossRef CAS PubMed;
(e) A. Cimino, F. Moscatelli, F. Ferretti, F. Ragaini, S. Germain, J. Hannedouche, E. Schulz, L. Luconi, A. Rossin and G. Giambastiani, New J. Chem., 2016, 40(12), 10285 RSC;
(f) H.-Q. Li, X. Liu, Q. Zhang, S.-S. Li, Y.-M. Liu, H.-Y. He and Y. Cao, Chem. Commun., 2015, 51, 11217 RSC;
(g) J. W. Park and Y. K. Chung, ACS Catal., 2015, 5, 4846 CrossRef CAS;
(h) F. Ferretti, M. A. EL-Atawy, S. Muto, M. Hagar, E. Gallo and F. Ragaini, Eur. J. Org. Chem., 2015, 5712 CrossRef CAS;
(i) S. E. Denmark and Z. D. Matesich, J. Org. Chem., 2014, 79(13), 5970 CrossRef CAS PubMed;
(j) F. Ferretti, F. Ragaini, R. Lariccia, E. Gallo and S. Cenini, Organometallics, 2010, 29(6), 1465 CrossRef CAS;
(k) S. E. Denmark and S. T. Nguyen, Org. Lett., 2009, 11(3), 781 CrossRef CAS PubMed;
(l) F. Ragaini, F. Ventriglia, M. Hagar, S. Fantauzzi and S. Cenini, Eur. J. Org. Chem., 2009, 2185 CrossRef CAS;
(m) F. Ragaini, M. Gasperini, S. Cenini, L. Arnera, A. Caselli, P. Macchi and N. Casati, Chem. – Eur. J., 2009, 15(32), 8064 CrossRef CAS PubMed;
(n) F. Ragaini, Dalton Trans., 2009, 32, 6251 RSC;
(o) F. Ragaini, S. Cenini, S. Tollari, G. Tummolillo and R. Beltrami, Organometallics, 1999, 18(5), 928 CrossRef CAS;
(p) S. Cenini, F. Ragaini, S. Tollari and D. Paone, J. Am. Chem. Soc., 1996, 118(47), 11964 CrossRef CAS.
-
(a) O. I. Afanasyev, A. A. Tsygankov, D. L. Usanov and D. Chusov, Org. Lett., 2016, 18, 5968 CrossRef CAS PubMed;
(b) O. I. Afanasyev, A. A. Tsygankov, D. L. Usanov, D. S. Perekalin, N. V. Shvydkiy, V. I. Maleev, A. R. Kudinov and D. Chusov, ACS Catal., 2016, 6(3), 2043 CrossRef CAS;
(c) N. Z. Yagafarov, P. N. Kolesnikov, D. L. Usanov, V. V. Novikov, Y. V. Nelyubina and D. Chusov, Chem. Commun., 2016, 52(7), 1397 RSC;
(d) P. N. Kolesnikov, N. Z. Yagafarov, D. L. Usanov, V. I. Maleev and D. Chusov, Org. Lett., 2015, 17(2), 173 CrossRef CAS PubMed;
(e) P. N. Kolesnikov, D. L. Usanov, E. A. Barablina, V. I. Maleev and D. Chusov, Org. Lett., 2014, 16, 5068 CrossRef CAS PubMed;
(f) N. Z. Yagafarov, D. L. Usanov, A. P. Moskovets, N. D. Kagramanov, V. I. Maleev and D. Chusov, ChemCatChem, 2015, 7(17), 2590 CrossRef CAS;
(g) D. Chusov and B. List, Angew. Chem., Int. Ed., 2014, 53, 5199 CAS.
- For the state-of-the art applications of metal carbonyls as a reducing agent see:
(a) F. Zhou, D.-S. Wang, X. Guan and T. G. Driver, Angew. Chem., Int. Ed., 2017, 56(16), 4530 CrossRef CAS PubMed;
(b) N. Jana, F. Zhou and T. G. Driver, J. Am. Chem. Soc., 2015, 137(21), 6738 CrossRef CAS PubMed;
(c) F. Zhou, D.-S. Wang and T. G. Driver, Adv. Synth. Catal., 2015, 357(16–17), 3463 CrossRef CAS;
(d) B. J. Stokes and T. G. Driver, Eur. J. Org. Chem., 2011,(22), 4071 CrossRef CAS.
-
(a) S. C. Shim, K. T. Huh and W. H. Park, Tetrahedron, 1986, 42(1), 259 CrossRef CAS;
(b) S. C. Shim and Y. Watanabe, Bull. Korean Chem. Soc., 1982, 3(2), 76 CAS;
(c) Y. Watanabe, S. C. Shim, T. Mitsudo, M. Yamashita and Y. Takegami, Bull. Chem. Soc. Jpn., 1976, 49(8), 2302 CrossRef CAS;
(d) Y. Watanabe, S. C. Shim, T. Mitsudo, M. Yamashita and Y. Takegami, Bull. Chem. Soc. Jpn., 1976, 49(5), 1378 CrossRef CAS.
-
http://www.fda.gov
. US Pharmacopeia general chapter <231>.
- The carbonyls can be purchased from Sigma-aldrich. Fe(CO)5 231 USD per kg (0.23 USD per g); Mn2(CO)10 109 USD per 50 g (2.18 USD per g); Mo(CO)6 1322 USD per 500 g (2.64 USD per g); Co2(CO)8 1532 USD per 500 g (3.06 USD per g); W(CO)6 456 USD per 100 g (4.56 USD per g); Cr(CO)6 498 USD per 100 g (4.98 USD per g).
-
(a) P. Krumholz and H. M. A. Stettiner, J. Am. Chem. Soc., 1949, 71(9), 3035 CrossRef CAS;
(b)
P. Rittmeyer and U. Wietelmann, in Ullmann's Encyclopedia of Industrial Chemistry, Wiley-VCH Verlag GmbH & Co. KGaA, 2000 Search PubMed.
- W. Hieber and N. Kahlen, Chem. Ber., 1958, 91, 2223 CrossRef CAS.
- S. Mukherjee, J. W. Yang, S. Hoffmann and B. List, Chem. Rev., 2007, 107(12), 5471 CrossRef CAS PubMed.
Footnotes |
† Electronic supplementary information (ESI) available: General procedure, experimental details, IR, HRMS, and NMR spectra. See DOI: 10.1039/c7ob02795h |
‡ Current address: Department of Chemistry and Chemical Biology, Harvard University, 12 Oxford Street, Cambridge, MA 02138 (USA). |
|
This journal is © The Royal Society of Chemistry 2017 |