DOI:
10.1039/C6GC03142K
(Communication)
Green Chem., 2017,
19, 112-116
Copper-catalyzed S-methylation of sulfonyl hydrazides with TBHP for the synthesis of methyl sulfones in water†
Received
15th November 2016
, Accepted 28th November 2016
First published on 28th November 2016
Abstract
A copper-catalyzed S-methylation of sulfonyl hydrazides with TBHP was efficiently developed, providing a variety of methyl sulfones with good to excellent yields. The reaction can be carried out in water smoothly without any ligand or additive under mild conditions and this catalyst-in-water can be recycled several times.
Introduction
The formation of C–S bonds has drawn much attention over the years in chemical synthesis due to their prevalence in many biologically active compounds as well as in organic materials.1 As a type of these species, methyl sulfones have emerged as important synthetic targets in recent years due to their promising antibacterial, antifungal and antitumor activities and so on.2 For example, MK-0524 was found to be the prostaglandin D2 (DP) antagonist,3 and, additionally, rofecoxib and etoricoxib were identified as very effective and specific cyclooxygenase-2 inhibitors (Fig. 1).4 Thus, substantial attention has been paid to approaches for acquiring methyl sulfones during the past several decades.
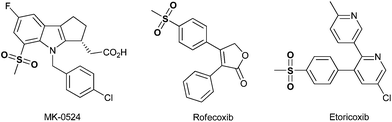 |
| Fig. 1 Structures of MK-0524, rofecoxib and etoricoxib. | |
Traditionally, known procedures to obtain methyl sulfones are mainly based on the oxidation of the corresponding sulfides,5 the electrophilic aromatic substitution of arenes,6 the coupling reaction of arylboronic acids,7 and the reaction of organomagnesium halides8 or organolithium compounds9 with sulfonate esters. However, with the desire of chemists to minimize synthetic steps and the amount of toxic waste in the formation of the C–S bond, and, moreover, to find more selective and milder transformations, the concepts of green chemistry10 have witnessed explosive developments in the past few decades. As a consequence of the often green chemical character, significant improvements in the design and optimization of new oxidation reactions have been made recently. For instance, He et al. reported an iron-catalyzed selective oxidation of sulfides to sulfoxides with the polyethylene glycol/O2 system (Fig. 2a).11 Subsequently, Yuan et al. utilized atmospheric oxygen as the oxidant, and developed a novel copper(I)-catalyzed synthesis of aryl methyl sulfones from aryl halides and easily available DMSO (Fig. 2b).12 Recently, an oxygenation reaction of thioether by using H2O2 under catalysis of a gigantic Zr24-cluster-substituted polyoxometalate was reported by Yang et al. (Fig. 2c).13 The studies mentioned above are usually focused on the employment of different catalysts or oxidants. However, methylation,14 known as a fundamental transformation in organic chemistry, has long been neglected as an important efficient strategy for the direct synthesis of methyl sulfones.
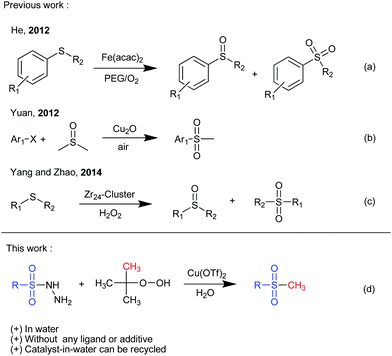 |
| Fig. 2 (a) The iron-catalyzed selective oxidation of sulfides; (b) the copper(I)-catalyzed synthesis of aryl methyl sulfones; (c) the oxygenation reaction of thioether by H2O2; (d) the copper-catalyzed S-methylation of sulfonyl hydrazides. | |
On the other hand, employing sulfonyl hydrazides as the sulfonyl precursor in the presence of tert-butyl hydroperoxide (TBHP) has been well developed in recent years.15 In this type of methodology, TBHP provides an efficient access toward forming sulfonyl radicals, which then performed with other building blocks. However, the study on the direct S-methylation of sulfonyl hydrazides with TBHP for the synthesis of methyl sulfones has rarely been reported. With our ongoing interest in various in-water and on-water green chemical methodologies in organic synthesis,16 moreover, in view of the importance of methyl sulfones and the related scaffolds, we report herein a simple copper-catalyzed S-methylation of sulfonyl hydrazides, in which organic peroxide served as a methylating reagent, thus offering a new convenient pathway for the synthesis of various methyl sulfones (Fig. 2d). In comparison with the previous reports on the construction of methyl sulfones, this new S-methylation can be carried out in water with the catalyst-in-water recycled several times without any ligand or additive, affording a much more environmentally benign and economical methodology.
Results and discussion
Initially, 4-methylbenzenesulfonyl hydrazide (1a) was treated with Cu(OAc)2 and di-tert-butyl peroxide (DTBP) in water at 120 °C for 12 h. No product was observed. First of all, different peroxides were examined in the reaction (Scheme 1, entries 2 and 3). It was found that TBHP could perform well to afford the desired product 3a with a yield of 38%. Based on the previous reports about the activation of TBHP by iodine and copper,14g,17 we investigated different iodine and copper sources as catalysts. Iodine sources showed no catalytic activity (Scheme 1, entries 4 and 5), whereas product formation in moderate to excellent yields was observed when copper sources were employed in the reaction (Scheme 1, entries 6–10).
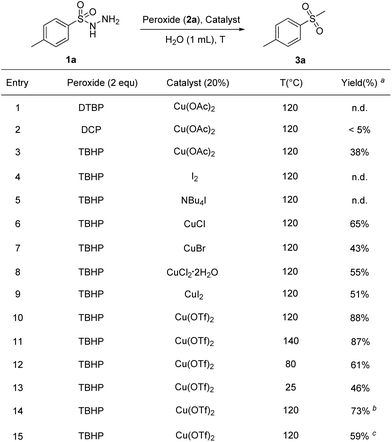 |
| Scheme 1 Optimization of the reaction conditions. Reaction conditions: 1a (0.20 mmol), 2a (0.40 mmol), catalyst (20 mol%), solvent (1 mL), under N2, 12 h. a Isolated yields based on 1a. b Under air. c Under O2. | |
Screening of the temperature indicated 120 °C to be optimal with the expected product 3a obtained in 88% yield under catalysis of Cu(OTf)2. Raising the temperature had no effect on the yield while lowering the reaction temperature decreased the yields largely (Scheme 1, entries 11–13). When the reaction was subjected to air or an oxygen atmosphere instead of nitrogen, a relatively lower yield was obtained (Scheme 1, entries 14 and 15). After investigation, the optimal reaction condition was decided as below. The reaction of 1a with 2a was carried out in water under a nitrogen atmosphere at 120 °C in the presence of Cu(OTf)2.
With the optimal conditions established, we examined the scope of this S-methylation of sulfonyl hydrazides. The examples of the reactions between TBHP and sulfonyl hydrazides are presented in Scheme 2. We found that a series of substituted sulfonyl hydrazides 1a could perform well in this reaction, affording the corresponding products with moderate to excellent yields. Electron-rich and electron-neutral aryl sulfonyl hydrazides were typically employed. In general, the substrates with electron-donating groups (R = Me, OMe) on the phenyl ring gave slightly higher yields than those bearing electron-withdrawing groups (R = I, CF3, CN, NO2). Interestingly, the reaction with some electron-deficient substituents such as phenyl halide substituted sulfonyl hydrazides (1f and 1g) could proceed smoothly. In particular, 4-fluoro-phenyl sulfonyl hydrazides worked well in the reaction to afford the desired product with a high yield, indicating that the electron-deficient nature of the aryl halide favored the reaction. Sterically encumbered aryl sulfonyl hydrazides (2k–m) were also investigated and it was found that hindrance had a great negative influence on the reaction. For instance, ortho-nitro benzene-sulfonyl hydrazides furnished a trace amount of the corresponding product 3ma. α-Naphthyl sulfonyl hydrazides (3qa) were obtained with a lower yield (59%) than that (81%) of β-naphthyl sulfonyl hydrazides (3pa) perhaps due to the hindrance of the α-hydrogen atom close to the sulfonyl in the α-naphthyl sulfonyl hydrazides. On the other hand, disubstituted benzenesulfonyl-hydrazides can also be employed as reaction substrates, affording the methyl sulfone products 3na and 3oa with good yields. It was also worth noting that when the aryl ring of the sulfonyl hydrazide was replaced by the thiophene ring, the corresponding thiolation product could also be obtained with a yield of 69%. In addition, we proved that this method was remarkably compatible with some aliphatic sulfonyl hydrazides (2s and 2t).
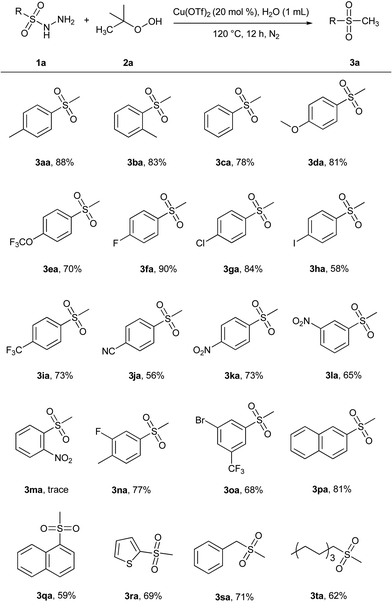 |
| Scheme 2
S-Methylation of sulfonyl hydrazides. Reaction conditions: sulfonyl hydrazides (0.20 mmol), TBHP (0.40 mmol) and Cu(OTf)2 (20 mol%) in 1 mL of H2O, 120 °C, under N2, 12 h. Isolated yields based on sulfonyl hydrazides. | |
Furthermore, the recycle of the catalyst-in-water was investigated. The Cu(OTf)2 catalyst was stable in air with good solubility in water but poor solubility in ethyl acetate. By virtue of this characteristic, catalyst-in-water could be recovered by a simple phase separation from the organic layer. The recovered catalyst-in-water could then be reused in the next round. As shown in Scheme 3, a slight loss of catalytic activity was observed after the six round, giving the corresponding methyl sulfones with a yield of 73%.
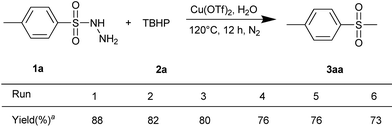 |
| Scheme 3 Recycling catalyst-in-water system. Reaction conditions: 1a (0.20 mmol), 2a (0.40 mmol), Cu(OTf)2 (20 mol%), H2O (1 mL), under N2, 12 h. a Isolated yields based on 1a. | |
Encouraged by this green and sustainable synthesis, we then conducted scale-up experiments to examine the synthetic utility. When 10 mmol of 4-methylphenyl-sulfonyl hydrazide 1a was treated with 20 mmol of TBHP 2a, the reaction also proceeded well to give the corresponding product 3aa with a satisfactory yield of 72%, although an extended reaction time was required (Scheme 4). The method could be used to prepare precursors of some important bioactive molecules, demonstrating great potential in pharmaceutical synthesis.
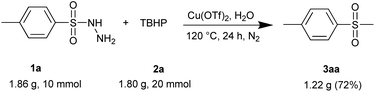 |
| Scheme 4 The scale-up reaction: 1a (10 mmol) and 2a (20 mmol) and Cu(OTf)2 (20 mol%) in 10 mL of H2O, 120 °C, under N2, 24 h. Product 3aa was isolated in 72% yield. | |
Studies on the reaction mechanism
To gain an insight into the mechanism of this S-methylation process, several control experiments were conducted. First, radical trapping experiment was conducted to elucidate whether the reaction involves radical species. When the radical scavenger 2,2,6,6-tetramethyl-1-piperidinyloxy (TEMPO; 2 equiv.) was employed under the standard conditions (Scheme 5a), the S-methylation process was completely shut off and no 3a was detected. In addition, the TEMPO–CH3 adduct 4 was detected by GC–MS, which indicates that a free radical process should be involved. On the other hand, the employment of 5 could afford 3ca in 59% yield (Scheme 5b), suggesting that benzenesulfinic acid might be an intermediate in this reaction.
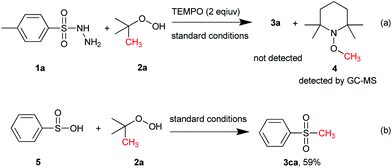 |
| Scheme 5 Mechanistic investigations of the S-methylation process. | |
On the basis of the aforementioned experimental results and the previous reports,14g,16,17 a possible reaction pathway was proposed and is depicted in Scheme 6. Initially, thermally promoted homolytic cleavage of the radical initiator TBHP produces a tert-butoxy radical tBuO˙, which converts to the methyl radical by releasing 1 equiv. of acetone in the presence of Cu.17c,18 Subsequently, sulfonyl hydrazides transform into major intermediate 6 which can isomerize to 7 to form copper intermediate 8 in the presence of Cu(II). Finally, the methyl radical reduces the Cu(II) intermediate 8 to produce S-methylation compound 3a and regenerate Cu(I), which is oxidized to Cu(II) for further reactions.
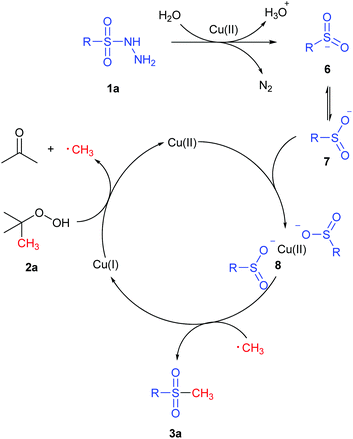 |
| Scheme 6 The proposed mechanism for the reaction. | |
Conclusions
A copper-catalyzed S-methylation of sulfonyl hydrazides with TBHP was efficiently developed, affording a variety of methyl sulfones with good to excellent yields. This new S-methylation can be carried out in water under environmentally benign conditions without any ligand or additive. And the copper catalyst-in-water can be recovered by a simple phase separation and this catalyst in water can be recycled several times without obvious loss of catalytic activity. Also, the by-products were nitrogen, acetone and water, no pollution in the environment. What's more, the reaction has a broad scope of the reaction substrates, a variety of functional groups can perform well under the reaction conditions. Ongoing research including further mechanistic details, expanding the substrate scope and applications in organic synthesis is currently underway.
Experimental
A mixture of sulfonyl hydrazides (0.20 mmol), TBHP (0.40 mmol) and Cu(OTf)2 (20 mol%) in water (1 mL) was put into a Schlenk tube at 120 °C under magnetic stirring for 12 h under nitrogen. After the reaction was complete, the mixture was extracted with EtOAc (3 × 5 mL) and then the combined organic extracts were washed with brine (10 mL), dried over sodium sulfate, and filtered. The solvent was removed under reduced pressure and the residue was purified by flash column chromatography (hexane/ethyl acetate = 3
:
1) to give compound 3.
Acknowledgements
We are grateful to the National Nature Science Foundation of China (21272222, 91213303, 21172205, J1030412).
Notes and references
-
(a) C. Zhang, C. Tang and N. Jiao, Chem. Soc. Rev., 2012, 41, 3464 RSC;
(b) S. R. Neufeldt and M. S. Sanford, Acc. Chem. Res., 2012, 45, 936 CrossRef CAS PubMed;
(c) L.-H. Zou, J. Reball, J. Mottweiler and C. Bolm, Chem. Commun., 2012, 48, 11307 RSC;
(d) C. Shen, P. Zhang, Q. Sun, S. Bai, T. S. Andy Hor and X. Liu, Chem. Soc. Rev., 2015, 44, 291 RSC;
(e) Y. T. Kondo and T. Mitsudo, Chem. Rev., 2000, 100, 3205 CrossRef PubMed;
(f) Q. Lu, J. Zhang, F. Wei, Y. Qi, H. Wang, Z. Liu and A. Lei, Angew. Chem., Int. Ed., 2013, 52, 7156 CrossRef CAS PubMed.
-
(a) M.-J. Langman, D.-M. Jensen, D.-J. Watson, S.-E. Harper, P.-L. Zhao, H. Quan, J.-A. Bolognese and T.-J. Simon, J. Am. Med. Assoc., 1999, 282, 1929 CrossRef CAS;
(b) D. Bachovchin, A. Zuhl, A. Speers, M. Wolfe, E. Weerapana, S. Brown, H. Rosen and B. Cravatt, J. Med. Chem., 2011, 54, 5229 CrossRef CAS PubMed.
-
(a) C. F. Sturino, G. O'Neill, N. Lachance, M. Boyd, C. Berthelette, M. Labelle, L. Li, B. Roy, J. Scheigetz, N. Tsou, Y. Aubin, K. P. Bateman, N. Chauret, S. H. Day, J.-F. Levesque, C. Seto, J. H. Silva, L. A. Trimble, M.-C. Carriere, D. Denis, G. Greig, S. Kargman, S. Lamontagne, M.-C. Mathieu, N. Sawyer, D. Slipetz, W. M. Abraham, T. Jones, M. McAuliffe, H. Piechuta, D. A. Nicoll-Griffith, Z. Wang, R. Zamboni, R. N. Young and K. M. Metters, J. Med. Chem., 2007, 50, 794 CrossRef CAS PubMed;
(b) A. Kar, I. A. Sayyed, W. F. Lo, H. M. Kaiser, M. Beller and M. K. Tse, Org. Lett., 2007, 17, 3405 CrossRef PubMed.
-
(a) G. Yuan, J. Zheng, X. Gao, X. Li, L. Huang, H. Chen and H. Jiang, Chem. Commun., 2012, 48, 7513 RSC;
(b) S.-P. Khanapure, D.-S. Garvey, D.-V. Young, M. Ezawa, R.-A. Earl, R.-D. Gaston, X. Fang, M. Murty, A. Martino, M. Shumway, M. Trocha, P. Marek, S.-W. Tam, D.-R. Janero and L.-G. Letts, J. Med. Chem., 2003, 46, 5484 CrossRef CAS PubMed;
(c) R.-W. Friesen, C. Brideau, C.-C. Chan, S. Charleson, D. Deschênes, D. Dubé, D. Ethier, R. Fortin, J.-Y. Gauthier, Y. Girard, R. Gordon, G.-M. Greig, D. Riendeau, C. Savoie, Z. Wang, E. Wong, D. Visco, J.-L. Xu and R.-N. Young, Bioorg. Med. Chem. Lett., 1998, 8, 2777 CrossRef CAS PubMed.
-
(a)
S. Oae, Organic Sulfur Chemistry, CRC Press, Boca
Raton, FL, 1992, ch. 6 Search PubMed;
(b) M. Gonza-Nenuz, R. Mello, J. Royo, J.-V. Rios and G. Asensio, J. Am. Chem. Soc., 2002, 124, 9154 Search PubMed;
(c) C.-A. Gamelas, T. Lourenco, D. Costa, A.-L. Simplício, B. Royo and C.-C. Romao, Tetrahedron Lett., 2008, 49, 4708 CrossRef CAS;
(d) N. Fukuda and T. Ikemoto, J. Org. Chem., 2010, 75, 4629 CrossRef CAS PubMed;
(e) B. M. Choudary, C. R. V. Reddy, B. V. Prakash, M. L. Kantam and B. Sreedhar, Chem. Commun., 2003, 754 RSC;
(f) S.-L. Jain, B.-S. Rana, B. Singh, A.-K. Sinha, A. Bhaumik, M. Nandi and B. Sain, Green Chem., 2010, 12, 374 RSC.
-
(a) B. M. Graybill, J. Org. Chem., 1967, 32, 2931 CrossRef CAS;
(b) M. Ueda, K. Uchiyama and T. Kano, Synthesis, 1984, 323 CrossRef CAS.
-
(a) F. Huang and R.-A. Batey, Tetrahedron, 2007, 63, 7667 CrossRef CAS;
(b) M.-K. Kantam, B.-N. Sreedhar and R. Chakravarti, Synlett, 2008, 1455 CrossRef CAS;
(c) B.-P. Bandgar, S.-V. Bettigeri and J. Phopase, Org. Lett., 2004, 6, 2105 CrossRef CAS PubMed;
(d) C. Beaulieu, D. Guay, Z. Wang and D.-A. Evans, Tetrahedron Lett., 2004, 45, 3233 CrossRef CAS;
(e) M.-B. Jeremy and Z. Wang, Org. Lett., 2002, 4, 4423 CrossRef PubMed;
(f) A. Kar, I.-A. Sayyed, W.-F. Lo, H.-M. Kaiser, M. Beller and M.-K. Tse, Org. Lett., 2007, 9, 3405 CrossRef CAS PubMed;
(g) S. Cacchi, G. Fabrizi, A. Goggiamani and L.-M. Parisi, Org. Lett., 2002, 4, 4719 CrossRef CAS PubMed;
(h) W. Zhu and D.-W. Ma, J. Org. Chem., 2005, 70, 2696 CrossRef CAS PubMed.
-
(a) H. Gilman, N. J. Beaver and C. H. Meyers, J. Am. Chem. Soc., 1925, 47, 2047 CrossRef CAS.
- W. H. Baarschers, Can. J. Chem., 1976, 54, 3056 CrossRef CAS.
-
(a) C.-J. Li and B. M. Trost, Proc. Natl. Acad. Sci. U. S. A., 2008, 105, 13197 CrossRef CAS PubMed;
(b) M.-O. Simon and C.-J. Li, Chem. Soc. Rev., 2012, 41, 1415 RSC;
(c) P. Anastas and N. Eghbali, Chem. Soc. Rev., 2010, 39, 301 RSC;
(d) T. P. Loh and G.-L. Chua, Chem. Commun., 2006, 2739 RSC;
(e) Y.-L. Liu, L. Liu, Y.-L. Wang, Y.-C. Han, D. Wang and Y.-J. Chen, Green Chem., 2008, 10, 635 RSC.
- B. Li, A.-H. Liu, L.-N. He, Z.-Z. Yang, J. Gao and K.-H. Chen, Green Chem., 2012, 14, 130 RSC.
- G. Yuan, J. Zheng, X. Gao, X. Li, L. Huang, H. Chen and H. Jiang, Chem. Commun., 2012, 48, 7513 RSC.
- L. Huang, S.-S. Wang, J.-W. Zhao, L. Cheng and G.-Y. Yang, J. Am. Chem. Soc., 2014, 136, 7637 CrossRef CAS PubMed.
-
(a) Y. Zhang, J. Feng and C.-J. Li, J. Am. Chem. Soc., 2008, 130, 2900 CrossRef CAS PubMed;
(b) Q. Xia, X. Liu, Y. Zhang, C. Chen and W. Chen, Org. Lett., 2013, 13, 3326 CrossRef PubMed;
(c) J.-H. Fan, M.-B. Zhou, Y. Liu, W.-T. Wei, X.-H. Ouyang, R.-J. Song and J.-H. Li, Synlett, 2014, 0657 CAS;
(d) Q. Dai, J. Yu, Y. Jiang, S. Guo, H. Yang and J. Cheng, Chem. Commun., 2014, 50, 3865 RSC;
(e) Z. Xu, C. Yan and Z.-Q. Liu, Org. Lett., 2014, 16, 5670 CrossRef CAS PubMed;
(f) S. Guo, Q. Wang, Y. Jiang and J.-T. Yu, J. Org. Chem., 2014, 79, 11285 CrossRef CAS PubMed;
(g) Y. Bao, Y. Yan, K. Xu, J. Su, Z. Zha and Z. Wang, J. Org. Chem., 2015, 80, 4736 CrossRef CAS PubMed.
-
(a) X. Li, X. Xu and C. Zhou, Chem. Commun., 2012, 48, 12240 RSC;
(b) X. Li, X. Xu and Y. Tang, Org. Biomol. Chem., 2013, 11, 1739 RSC;
(c) X. Li, X. Xu, P. Hu, X. Xiao and C. Zhou, J. Org. Chem., 2013, 78, 7343 CrossRef CAS PubMed;
(d) X. Li, X. Shi, M. Fang and X. Xu, J. Org. Chem., 2013, 78, 9499 CrossRef CAS PubMed;
(e) X. Li, X. Xu and X. Shi, Tetrahedron Lett., 2013, 54, 3071 CrossRef CAS;
(f) Y. Tang, Y. Fan, H. Gao, X. Li and X. Xu, Tetrahedron Lett., 2015, 56, 5616 CrossRef CAS;
(g) W. Yu, P. Hu, Y. Fan, C. Yu, X. Yan, X. Li and X. Xu, Org. Biomol. Chem., 2015, 13, 3308 RSC.
-
(a) Y. Yang, L. Tang, S. Zhang, X. Guo, Z. Zha and Z. Wang, Green Chem., 2014, 16, 4106 RSC;
(b) Y. Yang, S. Zhang, L. Tang, Y. Hu, Z. Zha and Z. Wang, Green Chem., 2016, 18, 2609 RSC.
-
(a) R. T. Gephart, C. L. McMullin, N. G. Sapiezynski, E. S. Jang, M. J. B. Aguila, T. R. Cundari and T. H. Warren, J. Am. Chem. Soc., 2012, 134, 17350 CrossRef CAS PubMed;
(b) Q. Xia, X. Liu, Y. Zhang, C. Chen and W. Chen, Org. Lett., 2013, 13, 3326 CrossRef PubMed;
(c) S. Guo, Q. Wang, Y. Jiang and J.-T. Yu, J. Org. Chem., 2014, 79, 11285 CrossRef CAS PubMed.
- Q. Dai, T. Yu, Y. Jiang, S. Guo, H. Yang and J. Cheng, Chem. Commun., 2014, 50, 3865 RSC.
Footnotes |
† Electronic supplementary information (ESI) available: Experimental details and characterization of products. See DOI: 10.1039/c6gc03142k |
‡ These authors contributed equally to this work. |
|
This journal is © The Royal Society of Chemistry 2017 |