DOI:
10.1039/C6SC03036J
(Edge Article)
Chem. Sci., 2017,
8, 627-630
Enantioselective allylic alkylation of stereodefined polysubstituted copper enolates as an entry to acyclic quaternary carbon stereocentres†
Received
10th July 2016
, Accepted 25th August 2016
First published on 15th September 2016
Abstract
A sequence of regio- and stereoselective carbometalation followed by oxidation of ynamides leads to stereodefined fully substituted enolates that subsequently react with various functionalized allyl bromide reagents to provide the expected products possessing an enantiomerically pure quaternary carbon stereocentre in the α-position to the carbonyl group in excellent yields and enantiomeric ratios after cleavage of the oxazolidinone moiety. Three new bonds are formed in a single-pot operation.
Introduction
The enantioselective synthesis of a quaternary carbon stereocentre α to a carbonyl group in acyclic systems is an ongoing challenge in the field of stereoselective synthesis, given the prevalence of these centres in a wide variety of natural products with important structural and biological properties.1 Even more challenging would be the preparation of these stereocentres from common starting materials with the concomitant creation of several new bonds in a single-pot operation.2 Additionally, any development of a new strategy that would answer this synthetic goal should be flexible enough to allow the direct preparation of functionalized quaternary stereocentres to avoid protection-deprotection steps.3 One of the main issues that has limited the formation of these stereocentres has been the lack of practical approaches to generate stereodefined acyclic β,β-disubstituted enolates.4 In this context, we have previously reported a simple, efficient and reliable stereoselective approach to polysubstituted stereodefined enolate species based on a carbometalation reaction of α-heterosubstituted alkynes followed by a selective in situ oxidation reaction.5 This approach was proven to be synthetically useful as it was successfully applied to the preparation of aldol and Mannich-type products in good overall yields and excellent diastereoselectivities (Scheme 1, path a).6 Alternatively, the aldol reaction with aliphatic aldehydes could be subsequently achieved by using stereodefined disubstituted silyl ketene aminals through the Mukaiyama aldol reaction (Scheme 1, path b).7 More recent studies have also shown that the preparation of stereochemically defined acyclic fully substituted enolates of ketones is now also possible from simple vinyl carbamates, and this has been successfully used in aldol and Mannich-type transformations (Scheme 1, path c).8
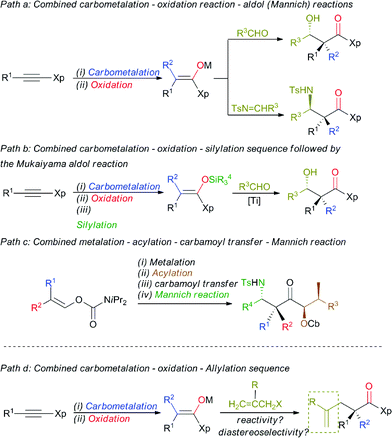 |
| Scheme 1 Formation of quaternary carbon stereocentres from alkynes and alkenes. Xp = chiral auxiliary. | |
Although these methodologies are now well established, the formation of these quaternary stereocentres is always accompanied by the creation of an additional adjacent stereocentre.
Results and discussion
The preparation of an acyclic single quaternary stereocentre devoid of any additional new ones would be highly desirable in the context of natural product synthesis.9 One potential solution to this question could emerge from the diastereoselective allylation of stereodefined polysubstituted metal enolates (Scheme 1, path d).10 It should be noted that the presence of this allylic functionality could be subsequently manipulated towards the synthesis of more complex molecular architectures. Herein, we disclose our approach for the diastereo- and enantioselective formation of quaternary stereocentres α to a carbonyl functionality via the allylation of stereodefined β,β-disubstituted amide copper enolates.
Stereodefined polysubstituted enolates could be formed through the addition of an organocuprate R22CuLi·Me2S, easily accessible by the addition of 2 equivalents of commercially available R2Li to 1 equivalent of CuBr·SMe2,11 to ynamide 1.12,13 1 Equivalent of t-BuOOH was then added to the formed vinyl cuprate 2Cuate, and the acidic hydrogen of the peroxide was deprotonated in situ by the remaining sacrificial R2 group of the vinyl copper species to produce an oxenoid as a reactive intermediate,14 which could undergo a 1,2-metalate rearrangement5,15 to give stereodefined copper enolate 3 (method A). Alternatively, the addition of an organocopper R2Cu·Me2S, now obtained by the addition of only one equivalent of the same commercially available R2Li to 1 equivalent of CuBr·SMe2,11 to ynamide 1 led to the formation of the stereodefined vinyl copper species 2Cu.
Treatment of the resulting 2Cu with freshly prepared oxenoid t-BuOOLi led to the formation of the same stereodefined copper enolate 3 after the 1,2-metalate rearrangement (method B). Then, addition at low temperature of various allyl bromide derivatives to the reaction mixture produced the expected allylated products 4 as described in Scheme 2.
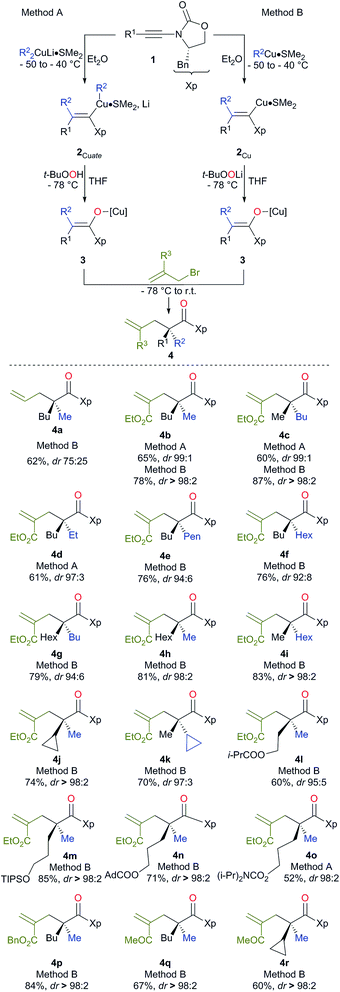 |
| Scheme 2 Formation of allylic alkylation products with quaternary carbon stereocentres. | |
Although the addition of allyl bromide did not provide the expected product, even in the presence of various polar coordinative additives such as HMPA, DMPU, TMEDA, the addition of a more reactive allyl iodide in the presence of HMPA gave the allylated product 4a, albeit in a low diastereoisomeric ratio (Scheme 2). The solution came when even more reactive electrophiles such as (bromomethyl) acrylate derivatives were used. To our delight, the reaction proceeded smoothly at low temperature either via the in situ preparation of the oxenoid (4b, 65% yield, dr > 98
:
2, method A) or via the addition of oxenoid prepared in an independent flask (4b, 78% yield, dr > 98
:
2, method B). 1H, 13C NMR and HPLC analyses of the crude reaction mixtures were used to determine the diastereoisomeric ratios of all synthesized products. Although diastereoisomeric ratios for most of the synthesized products were similarly high, yields were consistently higher when method B was used. It should be noted that lower diastereoisomeric ratios were obtained when the allylation reaction was performed at −50 °C instead of −78 °C (dr 96
:
4 and >98
:
2 respectively). By permuting the nature of the two alkyl groups (R1 on the ynamide and R2 of the organocopper derivatives), both diastereoisomers at the quaternary carbon stereocentre are easily accessible with similar diastereoisomeric ratios using the same strategy (compare 4b and 4c, Scheme 2). This strategy allows the creation of two C–C bonds and one C–O bond in a single-pot operation from a simple heterosubstituted alkyne with the creation of various acyclic quaternary carbon stereocentres (see 4d–i) in excellent diastereoisomeric ratios and yields (based on three consecutive chemical steps). Interestingly, branched alkyl groups such as cyclopropyl units and also functionalized alkyl chains could be introduced at the quaternary carbon stereocentre, expanding nicely the scope of the reaction (see 4j, k and 4l–o respectively). When benzyl-2-(bromomethyl) acrylate was used as the electrophile, similarly excellent diastereoselectivity and chemical yield were obtained (4p). When 3-bromo-but-3-en-2-one was used as the electrophile, we were delighted to observe that the reaction still proceeded with very high diastereomeric ratios even when the quaternary stereocentre possessed a cyclopropyl ring, albeit in slightly lower yields (4q and 4r respectively). It is important to note that this reaction could be scaled up to larger quantities (1.25 g of final product) without any erosion of the diastereoisomeric ratio and chemical yield (i.e. formation of 4b in 78% yield and dr > 98
:
2). The absolute configuration was determined by X-ray analysis of 4p (see ESI†)16 and assigned by analogy for all products described in Scheme 2. The stereochemistry of the major isomer could be rationalized by an anti diastereoselective allylic alkylation reaction of the stereodefined copper enolate 3. In this case, the oxazolidinone intramolecularly chelates the copper atom leading to a pseudo-metallacycle where one face is shielded by the benzyl group of the oxazolidinone. The functionalized allyl bromide approaches the enolate moiety anti to this bulky group as represented in Scheme 3. To corroborate our mechanistic hypothesis, any substituent on C1 of the electrophilic partner should induce steric interactions and therefore decrease the reactivity. Indeed, when (bromomethyl) acrylates bearing a methyl or a phenyl group at the terminal C1 position of the methylene unit were used, no allylic alkylation was observed even when the reactions were heated at higher temperature for longer periods of time.
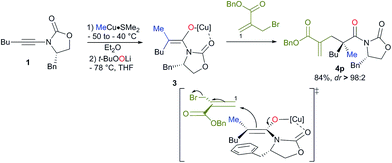 |
| Scheme 3 Proposed stereochemical model for the allylation reaction. | |
Cleavage of the oxazolidinone moiety of 4 could be performed by previously described17 high-yielding transformations with recovery of the chiral oxazolidinone as shown in Scheme 4 (see ESI†). For instance, addition of an excess of EtSLi to 4b,m in THF at −78 °C followed by oxidizing the crude reaction mixture with m-CPBA in DCM at 0 °C and finally heating in acetonitrile in the presence of DBU18 afforded the final products 5b, m in good overall yields and excellent enantioselectivities as described in Scheme 4.
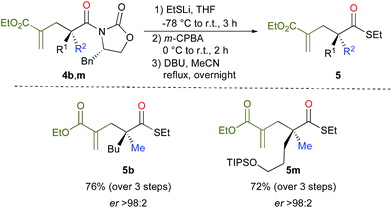 |
| Scheme 4 Cleavage of the oxazolidinone moiety of 4. | |
Conclusions
A sequence of regio- and stereoselective carbometalation followed by oxidation of ynamides leads to stereodefined fully substituted enolates that subsequently react with various functionalized allyl bromide reagents to provide the expected products possessing an enantiomerically pure quaternary carbon stereocentre in the α-position to the carbonyl group in excellent yields and enantiomeric ratios after cleavage of the oxazolidinone moiety. It should be emphasized that three new bonds are created in a single-pot operation en route to formation of the desired acyclic quaternary stereocentre.
Acknowledgements
This research was supported by the European Research Council under the European Community's Seventh Framework Program (ERC grant agreement no. 338912) and by the Israel Science Foundation administrated by the Israel Academy of Sciences and Humanities (140/12). I. M. is holder of the Sir Michael and Lady Sobell Academic Chair.
Notes and references
- For reviews, see:
(a) M. Büschleb, S. Dorich, S. Hanessian, D. Tao, K. B. Schenthal and L. E. Overman, Angew. Chem., Int. Ed., 2016, 55, 4156 CrossRef PubMed;
(b) K. W. Quasdorf and L. E. Overman, Nature, 2014, 516, 181 CrossRef CAS PubMed;
(c) A. Y. Hong and B. M. Stoltz, Eur. J. Org. Chem., 2013, 2745 CrossRef CAS PubMed;
(d) C. Hawner and A. Alexakis, Chem. Commun., 2010, 46, 7295 RSC;
(e) M. Bella and T. Casperi, Synthesis, 2009, 1583 CrossRef CAS;
(f) P. G. Cozzi, R. Hilgraf and N. Zimmermann, Eur. J. Org. Chem., 2007, 5969 CrossRef CAS;
(g) B. M. Trost and C. Jiang, Synthesis, 2006, 369 CrossRef CAS;
(h) J. Christoffers and A. Baro, Adv. Synth. Catal., 2005, 347, 1473 CrossRef CAS;
(i) I. Denissova and L. Barriault, Tetrahedron, 2003, 59, 10105 CrossRef CAS;
(j) E. J. Corey and A. Guzman-Perez, Angew. Chem., Int. Ed., 1998, 37, 388 CrossRef.
-
(a) G. Eppe, D. Didier and I. Marek, Chem. Rev., 2015, 115, 9175 CrossRef CAS PubMed;
(b) I. Marek, Y. Minko, M. Pasco, T. Mejuch, N. Gilboa, H. Chechik and J. D. Das, J. Am. Chem. Soc., 2014, 136, 2682 CrossRef CAS PubMed;
(c) K. P. McGrath and A. H. Hoveyda, Angew. Chem., Int. Ed., 2014, 53, 1910 CrossRef CAS PubMed;
(d) M. Pasco, N. Gilboa, T. Mejuch and I. Marek, Organometallics, 2013, 32, 942 CrossRef CAS.
-
(a) T. Newhouse, P. S. Baran and R. W. Hoffmann, Chem. Soc. Rev., 2009, 38, 3010 RSC;
(b) P. S. Baran, T. J. Maimone and J. M. Richter, Nature, 2007, 446, 404 CrossRef CAS PubMed;
(c) L. F. Tietze, Chem. Rev., 1996, 96, 115 CrossRef CAS PubMed;
(d) B. M. Trost, Science, 1991, 254, 1471 CAS;
(e) P. A. Wender and B. L. Miller, Nature, 2009, 460, 197 CrossRef CAS PubMed;
(f) I. Marek, Chem.–Eur. J., 2008, 14, 7460 CrossRef CAS PubMed.
-
(a) Y. Minko and I. Marek, Chem. Commun., 2014, 50, 12597 RSC;
(b) A. G. Doyle and E. N. Jacobsen, Angew. Chem., Int. Ed., 2007, 46, 3701 CrossRef CAS PubMed;
(c) S. E. Denmark, T. W. Wilson, M. T. Burke and J. R. Heemstra Jr, J. Am. Chem. Soc., 2007, 129, 14864 CrossRef CAS PubMed;
(d) E. D. Burke and J. L. Gleason, Org. Lett., 2004, 6, 405 CrossRef CAS PubMed;
(e) M. R. Morales, K. T. Mellem and A. G. Myers, Angew. Chem., Int. Ed., 2012, 51, 4568 CrossRef CAS PubMed;
(f) C. Allais, A. S. Tsai, P. Nuhant and W. R. Roush, Angew. Chem., Int. Ed., 2013, 52, 12888 CrossRef CAS PubMed;
(g) T. Abe, T. Suzuki, K. Sekiguchi, S. Hosokawa and S. Kobayashi, Tetrahedron Lett., 2003, 44, 9303 CrossRef CAS;
(h) Y.-C. Qin, C. E. Stivala and A. Zakarian, Angew. Chem., Int. Ed., 2007, 46, 7466 CrossRef CAS PubMed;
(i) Z. Gu, A. T. Herrmann, C. E. Stivala and A. Zakarian, Synlett, 2010, 11, 1717 Search PubMed.
- Y. Minko, M. Pasco, L. Lercher, M. Botoshansky and I. Marek, Nature, 2012, 490, 522 CrossRef CAS PubMed.
- Y. Minko, M. Pasco, L. Lercher and I. Marek, Nat. Protoc., 2013, 8, 749 CrossRef PubMed.
- Z. Nairoukh and I. Marek, Angew. Chem., Int. Ed., 2015, 54, 14393 CrossRef CAS PubMed.
- E. Haimov, Z. Nairoukh, A. Shterenberg, T. Berkovitz, T. F. Jamison and I. Marek, Angew. Chem., Int. Ed., 2016, 55, 5517 CrossRef CAS PubMed.
- For recent biologically active natural products possessing enantiomerically pure quaternary carbon stereocentres, see:
(a) E. A. Tiong, D. Riuvalti, B. M. Williams and J. L. Gleason, Angew. Chem., Int. Ed., 2013, 52, 3442 CrossRef CAS PubMed;
(b) G. M. Shibuya, J. A. Enquist and B. M. Stoltz, Org. Lett., 2013, 15, 3480 CrossRef CAS PubMed;
(c) F. Gao, J. L. Carr and A. H. Hoveyda, Angew. Chem., Int. Ed., 2012, 51, 6613 CrossRef CAS PubMed;
(d) A. Y. Hong and B. M. Stoltz, Angew. Chem., Int. Ed., 2012, 51, 9674 CrossRef CAS PubMed;
(e) Z.-J. Song, X.-M. Xu, W.-L. Deng, S.-L. Peng, L.-S. Ding and H.-H. Xu, Org. Lett., 2011, 13, 462 CrossRef CAS PubMed;
(f) J. A. Enquist, S. C. Virgil and B. M. Stoltz, Chem.–Eur. J., 2011, 17, 9957 CrossRef CAS PubMed;
(g) H. Mukherjee, N. T. McDougal, S. C. Virgil and B. M. Stoltz, Org. Lett., 2011, 13, 825 CrossRef CAS PubMed;
(h) I. P. Sing, J. Sidana, S. B. Bharate and W. Foley, Nat. Prod. Rep., 2010, 27, 393 RSC;
(i) E. L. Whitson, C. L. Thomas, C. J. Henrich, T. J. Sayers, J. B. MacMahon and T. C. Mackee, J. Nat. Prod., 2010, 73, 2013 CrossRef CAS PubMed.
-
(a) R. K. Boeckman, D. J. Boehmler and R. A. Musselman, Org. Lett., 2001, 3, 3777 CrossRef CAS PubMed;
(b) D. A. Kummer, W. J. Chain, M. R. Morales, O. Quiroga and A. G. Myers, J. Am. Chem. Soc., 2008, 130, 13231 CrossRef CAS PubMed;
(c) J. W. Medley and M. Movassaghi, Angew. Chem., Int. Ed., 2012, 51, 4572 CrossRef CAS PubMed;
(d) S. Hosokawa, K. Sekiguchi, M. Enemoto and S. Kobayashi, Tetrahedron Lett., 2000, 41, 6429 CrossRef CAS;
(e) B. W. H. Turnbull and P. A. Evans, J. Am. Chem. Soc., 2015, 137, 6156 CrossRef CAS PubMed.
-
(a) J.-F. Normant and A. Alexakis, Synthesis, 1981, 841 CrossRef CAS;
(b)
I. Marek and D. Tene, in Transition Metals for Fine Chemicals and Organic Synthesis, ed. C. Bolm and M. Beller, Wiley-VCH, Weinheim, 2nd edn, 2004 Search PubMed;
(c)
N. Chinkov, D. Tene and I. Marek, in Metal-Catalyzed Cross- Coupling Reactions, ed. F. Diederich and A. de Meijere, Wiley-VCH, Weinheim, 2nd edn, 2004 Search PubMed;
(d) I. Marek, J. Chem. Soc., Perkin Trans. 1, 1999, 535 RSC;
(e)
I. Marek and J.-F. Normant, in Transition Metals for Fine Chemicals and Organic Synthesis, ed. C. Bolm and M. Beller, Wiley-VCH, Weinheim, 1998, p. 514 Search PubMed;
(f)
I. Marek and Y. Minko, in Carbometalation Reactions in Metal-Catalyzed Cross-Coupling Reactions and More, ed. A. de Meijere, M. Oestreich and S. Brase, Wiley-VCH, Weinheim, 1st edn, 2014, p. 769 Search PubMed;
(g)
D. Didier and I. Marek, in Carbometalation Reactions in Copper-Catalyzed Asymmetric Synthesis, ed. A. Alexakis, N. Krause and S. Woodward, Wiley-VCH, Weinheim, 2014, p. 267 Search PubMed.
-
(a) Y. Minko, M. Pasco, H. Chechik and I. Marek, Beilstein J. Org. Chem., 2013, 9, 526 CrossRef CAS PubMed;
(b) H. Chechik-Lankin, S. Livshin and I. Marek, Synlett, 2005, 2239 Search PubMed;
(c) H. Chechik-Lankin and I. Marek, Org. Lett., 2003, 5, 5087 CrossRef CAS PubMed.
-
(a) G. Evano, A. Coste and K. Jouvin, Angew. Chem., Int. Ed., 2010, 49, 2840 CrossRef CAS PubMed;
(b) K. A. DeKorver, H. Li, A. G. Lohse, R. Hayashi, Z. Lu, Y. Zhang and R. Hsung, Chem. Rev., 2010, 110, 5064 CrossRef CAS PubMed.
-
(a) E. J. Panek, L. R. Kaiser and G. M. Whitesides, J. Am. Chem. Soc., 1977, 99, 3708 CrossRef CAS;
(b) D. Zhang and J. M. Ready, Org. Lett., 2005, 7, 5681 CrossRef CAS PubMed;
(c) J. R. DeBergh, K. M. Spivey and J. M. Ready, J. Am. Chem. Soc., 2008, 130, 7828 CrossRef CAS PubMed;
(d) Y. Minko and I. Marek, Org. Biomol. Chem., 2014, 12, 1535 RSC.
-
(a) R. P. Sonawane, V. Jheengut, C. Rabalakos, R. Larouche- Gauthier, H. K. Scott and V. K. Aggarwal, Angew. Chem., Int. Ed., 2011, 50, 3760 CrossRef CAS PubMed;
(b) H. K. Scott and V. K. Aggarwal, Chem.–Eur. J., 2011, 17, 13124 CrossRef CAS PubMed;
(c) D. S. Matteson and R. W. H. Mah, J. Am. Chem. Soc., 1963, 85, 2599 CrossRef CAS;
(d) O. Knopff, H. C. Stiasny and R. W. Hoffmann, Organometallics, 2004, 23, 705 CrossRef CAS;
(e) G. Boche and J. C. W. Lohrenz, Chem. Rev., 2001, 101, 697 CrossRef CAS PubMed.
- CCDC 1488151 contains the supplementary crystallographic data for this paper.
-
(a) T. Bixa, R. Hunter, A. Andrijevic, W. Petersen, H. Su and F. Dhoro, J. Org. Chem., 2015, 80, 762 CrossRef CAS PubMed;
(b) X. Cui, X. Xu, H. Lu, S. Zhu, L. Wojtas and X. P. Zhang, J. Am. Chem. Soc., 2011, 133, 3304 CrossRef CAS PubMed;
(c) A. L. Krasovsky, V. G. Nenajdenko and E. S. Balenkova, Tetrahedron, 2001, 57, 201 CrossRef.
- The addition of an excess of EtSLi leads to the thioester on the one hand and to the sulfa-Michael addition product on the other hand. Then, oxidation of the latter thioether with m-CPBA leads to the sulfone that is subsequently eliminated by heating in acetonitrile to regenerate the alkenyl moiety (see experimental procedure for details).
Footnote |
† Electronic supplementary information (ESI) available. CCDC 1488151. For ESI and crystallographic data in CIF or other electronic format see DOI: 10.1039/c6sc03036j |
|
This journal is © The Royal Society of Chemistry 2017 |