DOI:
10.1039/C6SC02451C
(Edge Article)
Chem. Sci., 2016,
7, 7050-7054
Intermolecular oxidative decarbonylative [2 + 2 + 2] carbocyclization of N-(2-ethynylaryl)acrylamides with tertiary and secondary alkyl aldehydes involving C(sp3)–H functionalization†
Received
3rd June 2016
, Accepted 16th July 2016
First published on 21st July 2016
Abstract
A new metal-free oxidative decarbonylative [2 + 2 + 2] carbocyclization of N-(2-ethynylaryl)acrylamides with tertiary and secondary alkyl aldehydes is described. This reaction enables the formation of three new C–C bonds in a single reaction by a sequence of oxidative decarbonylation, radical addition across C–C unsaturated bonds, C–H functionalization and annulation, and represents the first oxidative decarbonylative [2 + 2 + 2] carbocyclization approach using tertiary and secondary alkyl aldehydes as a two carbon unit for assembling six-membered carbocycle-fused polycycles.
Introduction
High-order carbocyclization reactions represent one of the most powerful methods for building complex carbocyclic frameworks. In this field, one particularly fascinating area is the intermolecular [2 + 2 + m] carbocyclization of 1,n-enynes, which continues to gain much attention due to its straightforward and highly atom-economic features.1–4 Significant achievements include the [2 + 2 + 2] carbocyclization strategy which can allow the formation of a six-membered carbocycle within a complex polycyclic system by introducing a two-carbon unit across 1,n-enynes.2,3 Despite these advances, there are still limitations with the available transformations, such as the requirement for noble metal catalysts and narrow two-carbon unit scope (e.g., alkynes,3a–e alkenes3h–m and arylsulfonyl chlorides3n). Therefore, it would be welcomed to develop more efficient methods, especially metal-free use of new two-carbon unit strategies, to achieve the [2 + 2 + 2] carbocyclization of 1,n-enynes, which unfortunately remains a great challenge.
The decarbonylation reaction has proven among the most important methods for the formation of diverse chemical bonds in synthesis, the majority of which focus on the cleavage of C–CHO bonds by extrusion of carbon monoxide (CO) gas and then transformation through radical5 and anionic intermediates.6,7 Such successful transformations include transition metal-catalyzed Pauson–Khand-type reactions of 1,n-enynes using aldehydes as the carbon monoxide (CO) source (Scheme 1a).7 To our knowledge, however, approaches for carbocyclization of 1,n-enynes with aldehydes as the “R” source have never been reported.5g Intrigued by these results, we envisioned that extension of the decarbonylation concept to the combination of N-(2-ethynylaryl)acrylamides and alkyl aldehydes would offer a novel method to assemble six-membered carbocycle-fused polycyclic architectures. Herein, we report a novel oxidative radical decarbonylative [2 + 2 + 2] carbocyclization of N-(2-ethynylaryl)acrylamides with tertiary and secondary alkyl aldehydes that can be achieved under metal-free conditions through a sequence of oxidative decarbonylation, radical addition across C–C unsaturated bonds, C–H functionalization and annulation (Scheme 1b).10 To the best of our knowledge, this method is the first metal-free radical-mediated decarbonylative [2 + 2 + 2] carbocyclization reaction of N-(2-ethynylaryl)acrylamides with tertiary alkyl aldehydes as a two carbon unit, which provides selective and straightforward access to important six-membered carbocycle-fused polycyclic skeletons, including tetrahydrophenanthridin-6(5H)-ones, 6H-indeno[1,2-c]quinolin-6-one, 6H-benzo[c]chromen-6-one and 1H-fluorene.9 Moreover, this reaction is applicable to secondary alkyl aldehydes and the selectivity is shifted towards the decarbonylative [2 + 2 + 1] carbocyclization with N-(2-ethynylaryl)acrylamides.
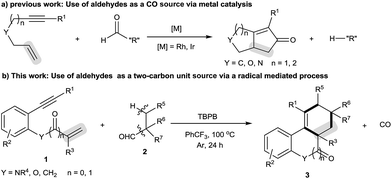 |
| Scheme 1 [2 + 2 + m] carbocyclization of 1,n-enynes with aldehydes. | |
Results and discussion
Our initial investigations focused on optimization of the decarbonylative [2 + 2 + 2] carbocyclization between N-methyl-N-(2-(phenylethynyl)phenyl)methacrylamide (1a) and pivalaldehyde (2a) (Table 1).11 When amide 1a and aldehyde 2a were subjected to tert-butyl perbenzoate (TBPB) oxidation in PhCF3 at 100 °C for 24 h, the desired decarbonylative [2 + 2 + 2] carbocyclization product 3aa was obtained in 81% yield (entry 1). Inspired by this result, a series of other oxidants, including tert-butyl hydroperoxide (TBHP), di-tert-butyl peroxide (DTBP) and K2S2O8, were examined (entries 2–4): they showed lower activity for the reaction than TBPB in terms of yields. A screening of the amount of TBPB revealed that 3 equiv. of TBPB was the best choice for further optimization (entries 5 and 6). We found that while a higher reaction temperature (110 °C) slightly affected the reaction (entry 7), a lower reaction temperature (90 °C) had an obviously negative effect (entry 8). Other solvents, including MeCN (entry 9), MeCONMe2 (entry 10) and PhCl (entry 11), were found to be less effective than PhCF3, thus giving diminished yields. Gratifyingly, the reaction could be satisfactorily performed at a 1 gram scale of amide 1a, providing 3aa in moderate yield (entry 12).
Table 1 Screening of optimal reaction conditionsa
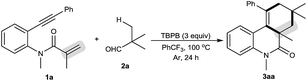
|
Entry |
Variation from the standard conditions |
Isolated yield (%) |
Reaction conditions: 1a (0.2 mmol), 2a (2 equiv.), TBPB (3 equiv.), PhCF3 (2 mL), argon, 100 °C for 24 h. TBHP (5 M in decane). Some by-products, including vinyl C–N bond-decomposition products, were observed.
1a (1 g, 3.64 mmol) and PhCF3 (5 mL) for 48 h.
|
1 |
None |
81 |
2 |
TBHP instead of TBPB |
10 |
3 |
DTBP instead of TBPB |
22 |
4 |
K2S2O8 instead of TBPB |
Trace |
5 |
TBPB (3.5 equiv.) |
80 |
6 |
TBPB (2.5 equiv.) |
71 |
7 |
At 110 °C |
80 |
8 |
At 90 °C |
62 |
9 |
MeCN instead of PhCF3 |
67 |
10 |
MeCONMe2 instead of PhCF3 |
20 |
11 |
PhCl instead of PhCF3 |
40 |
12b |
None |
73 |
The scope of this decarbonylative [2 + 2 + 2] carbocyclization protocol was probed with regard to both the N-(2-ethynylaryl)acrylamide 1 and aldehyde 2 (Table 2). Gratifyingly, a variety of N-(2-ethynylaryl)acrylamides 1b–s underwent the reaction with pivalaldehyde (2a) and TBPB to afford phenanthridin-6(5H)-ones 3 in moderate to good yields. We found that amides 1b–e bearing a wide range of N-substituents, such as N-Bn, N-allyl, N-Ts and even free N–H, were viable substrates to assemble 3ba–ea in moderate yields. With respect to the alkyne moiety in N-(2-ethynylaryl)acrylamides, the reaction was perfectly tolerant of various aryl substituents, namely 4-MeC6H4, 4-MeOC6H4, 4-BrC6H4, 4-ClC6H4, 4-FC6H4, 4-CNC6H4, 3-MeC6H4, 3-BrC6H4 and pyridin-3-yl groups, at the terminal alkyne, and the nature of the aryl group had no detrimental effect on the reaction (3fa–na).11 It was noted that in the case of the alkyl-substituted alkyne 1o a moderate yield of the product (3oa) was still achieved. It is important to emphasize that amides 1p–r with substituents, such as Me and Cl, on the 4- or 5-position of the N-aryl moiety were smoothly converted to 3pa–ra in 70–75% yields. To our surprise, amide 1s with a phenyl group on the 2-position of the acrylamide moiety shifted the selectivity towards functionalization of the phenyl C(sp2)–H bond, not the tert-butyl C(sp3)–H bond, thus furnishing 6H-indeno[1,2-c]quinolin-6-one 3sa rather than the phenanthridin-6(5H)-one.
Table 2 Carbocyclization of N-(2-ethynylaryl)acrylamides (1) with tertiary alkyl aldehydes (2)a
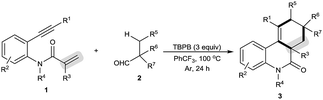
|
Reaction conditions: 1 (0.2 mmol), 2a (2 equiv.), TBPB (3 equiv.), PhCF3 (2 mL), argon, 100 °C for 24 h.
|
|
In the presence of amide 1a and TBPB, tertiary alkyl aldehydes, namely 3-ethyl-3-formylpentanenitrile (2b) and 2,2-dimethylpent-4-enal (2c), were also found to be viable substrates to produce the decarbonylative [2 + 2 + 2] carbocyclization products 3ab and 3ac. Notably, in the case of 2,2-dimethylpent-4-enal (2c) the functionalization of the more active allyl C(sp3)–H bond takes precedence over the methyl C(sp3)–H bond: 2,2-dimethylpent-4-enal (2c) successfully underwent the reaction with N-(2-ethynylaryl)acrylamides 1f, 1k and 1t, affording 3fc, 3kc and 3tc in moderate to good yields with high diastereoselectivity.
As shown in Scheme 2, the decarbonylative [2 + 2 + 2] carbocyclization protocol also allowed the formation of useful 6H-benzo[c]chromen-6-one 3ua (eqn (1)) and 1H-fluorenes 3va and 3vc (eqn (2)) from the corresponding 2-(phenylethynyl)phenyl methacrylate (1u) and methyl 2-(2-(phenylethynyl)benzyl)acrylate (1v), but a linear enyne, 4-methyl-N-(2-methylallyl)-N-(3-phenylprop-2-yn-1-yl)-benzenesulfonamide (1w), was not a suitable substrate.
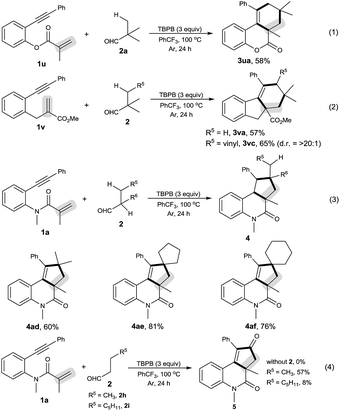 |
| Scheme 2 Variation of other 1,n-enynes and secondary alkyl aldehydes. | |
We found that secondary alkyl aldehydes 2d–f were viable substrates to perform the decarbonylative carbocyclization reaction, but the selectivity was shifted towards the functionalization of the C(sp3)–H bond adjacent to the aldehyde group, resulting in the construction of five-membered carbocycle-fused polycyclic skeletons (eqn (3)). For example, isobutyraldehyde (2d) underwent the decarbonylative [2 + 2 + 1] carbocyclization reaction to afford 4H-cyclopenta[c]quinolin-4-one 4ad in 60% yield. Using cyclopentanecarbaldehyde (2e) or cyclohexanecarbaldehyde (2f) also delivered the decarbonylative [2 + 2 + 1] carbocyclization products 4ae and 4af with a spirocyclic scaffold. Unfortunately, cyclopropane aldehyde (2g) was not a suitable aldehyde. Attempts to carbocyclize primary alkyl aldehydes, such as butyraldehyde (2h) and caprylic aldehyde (2i), failed to give the desired products. but instead provided the CO-inserted Pauson–Khand-type product, 3a,5-dimethyl-1-phenyl-3,3a-dihydro-2H-cyclopenta[c]quinoline-2,4(5H)-dione (5), in 57% and 8% yields, respectively (eqn (4)).7
To understand the mechanism, the control reaction of amide 1a with aldehyde 2a and TBPB was completely suppressed by a stoichiometric amount of radical inhibitors, including TEMPO, hydroquinone and BHT. Moreover, aldehyde 2a was converted into 1-(tert-butoxy)-2,2,6,6-tetramethylpiperidine (6) by reacting with TEMPO (eqn (5) in Scheme 3). These results implied that the decarbonylative [2 + 2 + 2] carbocyclization protocol involves a radical process. Therefore, the mechanism of this reaction is presented in Scheme 3.4,5,8,10 Aldehyde 2a is converted into alkyl radical Avia oxidative decarbonylation with the aid of TBPB.5 Addition of the alkyl radical A across the C–C double bond in amide 1a affords new alkyl radical intermediate B, followed by cyclization to produce unstable vinyl radical intermediate C.4,10 The intermediate C readily undergoes a 1,6-H shift followed by annulation to form intermediate E.8 Oxidation of intermediate E by TBPB affords the cationic intermediate F.4,5,8 Finally, deprotonation of intermediate F results in the formation of the product 3aa.
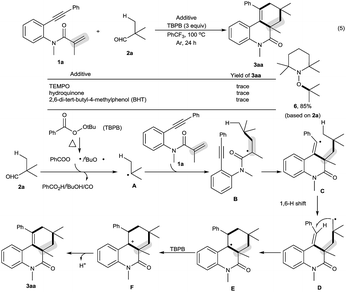 |
| Scheme 3 Control experiments and possible reaction mechanism. | |
Conclusions
In summary, we have developed the first oxidative decarbonylative [2 + 2 + m] annulation reaction of 1,n-enynes with tertiary and secondary alkyl aldehydes as a two-carbon unit source for the synthesis of diverse six-membered carbocycle-fused polycycles under metal-free conditions. This method proceeds through a sequence of oxidative decarbonylation, 1,6-H shift and annulation, and allows the one-step formation of three new C–C bonds with broad substrate scope and excellent functional group tolerance. Moreover, this method is applicable to secondary alkyl aldehydes leading to five-membered carbocyclic-ring-fused polycycles. Further studies will focus on the development of enantioselective aspects and other 1,n-enyne oxidative radical annulation reactions.
Acknowledgements
We thank the Natural Science Foundation of China (No. 21402046 and 21472039), the Specialized Research Fund for the Doctoral Program of Higher Education (No. 20120161110041), and the Hunan Provincial Natural Science Foundation of China (No. 13JJ2018) for financial support.
Notes and references
- For selected reviews, see:
(a) P. A. Wender, V. A. Verma, T. H. Paxton and T. H. Pillow, Acc. Chem. Res., 2008, 41, 40 CrossRef CAS PubMed;
(b) M. Lautens, W. Klute and W. Tam, Chem. Rev., 1996, 96, 49 CrossRef CAS PubMed;
(c) P. A. Inglesby and P. A. Evans, Chem. Soc. Rev., 2010, 39, 2791 RSC;
(d)
D. P. Curran and M. Harmata, Advances in Cycloaddition, JAI Press, Greenwich, CT, 1988–1999, vol. 1–6 Search PubMed;
(e)
W. Carruthers, Cycloaddition Reactions in Organic Synthesis, Tetrahedron Organic Chemistry Series, Pergamon, Elmsford, NY, 1990 Search PubMed;
(f) L. Yet, Chem. Rev., 2000, 100, 2963 CrossRef CAS PubMed;
(g) C. Aubert, O. Buisine and M. Malacria, Chem. Rev., 2002, 102, 813 CrossRef CAS PubMed.
- For reviews on [2 + 2 + 2] cycloaddition reactions, see:
(a) J. Varela and C. Saá, Chem. Rev., 2003, 103, 3787 CrossRef CAS PubMed;
(b) S. Kotha, E. Brahmachary and K. Lahiri, Eur. J. Org. Chem., 2005, 4741 CrossRef CAS;
(c) K. Tanaka, Chem.–Asian J., 2009, 4, 508 CrossRef CAS PubMed;
(d) B. R. Galan and T. Rovis, Angew. Chem., Int. Ed., 2009, 48, 2830 CrossRef CAS PubMed;
(e) G. Dominguez and J. Perez-Castells, Chem. Soc. Rev., 2011, 40, 3430 RSC;
(f) N. Weding and M. Hapke, Chem. Soc. Rev., 2011, 40, 4525 RSC.
-
(a) R. Grigg, R. Scott and P. J. Stevenson, J. Chem. Soc., Perkin Trans. 1, 1988, 1357 RSC;
(b) C. H. Oh, H. R. Sung, S. H. Jung and Y. M. Lim, Tetrahedron Lett., 2001, 42, 5493 CrossRef CAS;
(c) S. Kezuka, T. Okado, E. Niou and R. Takeuchi, Org. Lett., 2005, 7, 1711 CrossRef CAS PubMed;
(d) P. A. Evans, J. R. Sawyer, K. W. Lai and J. C. Huffman, Chem. Commun., 2005, 3971 RSC;
(e) C.-A. Chang, J. A. King Jr and K. P. C. Vollhardt, J. Chem. Soc., 1981, 53 Search PubMed;
(f) P. A. Evans, K. W. Lai and J. R. Sawyer, J. Am. Chem. Soc., 2005, 127, 12466 CrossRef CAS PubMed;
(g) S. García-Rubín, C. González-Rodríguez, C. García-Yebra, J. A. Varela, M. A. Esteruelas and C. Saá, Angew. Chem., Int. Ed., 2014, 53, 1841 CrossRef PubMed;
(h) B. M. Trost, K. Imi and A. F. Indolese, J. Am. Chem. Soc., 1993, 115, 8831 CrossRef CAS;
(i) J. Seo, H. M. P. Chui, M. J. Heeg and J. Montgomery, J. Am. Chem. Soc., 1999, 121, 476 CrossRef CAS;
(j) J. A. Varela, S. G. Rubín, C. González-Rodríguez, L. Castedo and C. Saá, J. Am. Chem. Soc., 2006, 128, 9262 CrossRef CAS PubMed;
(k) T. Shibata and Y. Tahara, J. Am. Chem. Soc., 2006, 128, 11766 CrossRef CAS PubMed;
(l) D. Tanaka, Y. Sato and M. Mori, J. Am. Chem. Soc., 2007, 129, 7730 CrossRef CAS PubMed;
(m) M. Schelwies, A. Farwick, F. Rominger and G. Helmchen, J. Org. Chem., 2010, 75, 7917 CrossRef CAS PubMed;
(n) G.-B. Deng, Z.-Q. Wang, J.-D. Xia, P.-C. Qian, R.-J. Song, M. Hu, L.-B. Gong and J.-H. Li, Angew. Chem., Int. Ed., 2013, 52, 1535 CrossRef CAS PubMed.
-
(a) M. Hu, J.-H. Fan, Y. Liu, X.-H. Ouyang, R.-J. Song and J.-H. Li, Angew. Chem., Int. Ed., 2015, 54, 9577 CrossRef CAS PubMed;
(b) J.-K. Qiu, B. Jiang, Y.-L. Zhu, W.-J. Hao, D.-C. Wang, J. Sun, P. Wei, S.-J. Tu and G. Li, J. Am. Chem. Soc., 2015, 137, 8928 CrossRef CAS PubMed;
(c) Z.-Z. Chen, S. Liu, W.-J. Hao, G. Xu, S. Wu, J.-N. Miao, B. Jiang, S.-L. Wang, S.-J. Tu and G. Li, Chem. Sci., 2015, 6, 6654 RSC;
(d) Y.-N. Wu, R. Fu, N.-N. Wang, W.-J. Hao, G. Li, S.-J. Tu and B. Jiang, J. Org. Chem., 2016, 81, 4762 CrossRef CAS PubMed;
(e) M. Hu, R.-J. Song, X.-H. Ouyang, F.-L. Tan, W.-T. Wei and J.-H. Li, Chem. Commun., 2016, 52, 3328 RSC;
(f) L.-Y. Lv and Z.-P. Li, Org. Lett., 2016, 18, 2264 CrossRef PubMed.
- For a review and selected papers, see:
(a)
J. W. Wilt, in Free Radicals, ed. J. K. Kochi, Wiley-interscience, New York, N.Y, 1973, vol. 1, ch. 8, pp. 346–356 Search PubMed;
(b) J. W. Wilt and H. Philip, J. Org. Chem., 1960, 25, 891 CrossRef CAS;
(c) J. W. Wilt and M. P. Stumpf, J. Org. Chem., 1965, 30, 1256 CrossRef CAS;
(d) J. W. Wilt and W. W. Pawlikowski, Jr, J. Org. Chem., 1975, 40, 3641 CrossRef CAS;
(e) J. R. Hwu and P. S. Furth, J. Am. Chem. Soc., 1989, 111, 8834 CrossRef CAS;
(f) A. Poloukhtine and V. V. Popik, J. Org. Chem., 2005, 70, 1297 CrossRef CAS PubMed; For a paper on the use of aldehydes as the “R” source for the cyclization of N-aryl acrylamides, see:
(g) L. Yang, W. Lu, W. Zhou and F. Zhang, Green Chem., 2016, 18, 2941 RSC.
- For reviews and representative papers, see:
(a) M. A. Garralda, Dalton Trans., 2009, 3635 RSC;
(b) T. Patra, S. Manna and D. Maiti, Angew. Chem., Int. Ed., 2011, 50, 12140 CrossRef CAS PubMed;
(c) K. Ohno and J. Tsuji, J. Am. Chem. Soc., 1968, 90, 99 CrossRef CAS;
(d) M. Kreis, A. Palmelund, L. Bunch and R. Madsen, Adv. Synth. Catal., 2006, 348, 2148 CrossRef CAS;
(e) T. Iwai, T. Fujihara and Y. Tsuji, Chem. Commun., 2008, 6215 RSC;
(f) P. Fristrup, M. Kreis, A. Palmelund, P. O. Norrby and R. Madsen, J. Am. Chem. Soc., 2008, 130, 5206 CrossRef CAS PubMed;
(g) K. Sen and J. C. Hackett, J. Am. Chem. Soc., 2010, 132, 10293 CrossRef CAS PubMed;
(h) A. Schirmer, M. A. Rude, X. Z. Li, E. Popova and S. B. del Cardayre, Science, 2010, 329, 559 CrossRef CAS PubMed;
(i) T. C. Fessard, S. P. Andrews, H. Motoyoshi and E. M. Carreira, Angew. Chem., Int. Ed., 2007, 46, 9331 CrossRef CAS PubMed;
(j) B. Gutmann, P. Elsner, T. Glasnov, D. M. Roberge and C. O. Kappe, Angew. Chem., Int. Ed., 2014, 53, 11557 CrossRef CAS PubMed;
(k) E. I. Gürbüz, D. D. Hibbitts and E. Iglesia, J. Am. Chem. Soc., 2015, 137, 11984 CrossRef PubMed;
(l) J. A. Varela, C. González-Rodríguez, S. G. Rubín, L. Castedo and C. Saá, J. Am. Chem. Soc., 2006, 128, 9576 CrossRef CAS PubMed;
(m) X. Guo, J. Wang and C.-J. Li, J. Am. Chem. Soc., 2009, 131, 15092 CrossRef CAS PubMed;
(n) P. Wang, H. Rao, F. Zhou, R. Hua and C.-J. Li, J. Am. Chem. Soc., 2012, 134, 16468 CrossRef CAS PubMed;
(o) Q. Shuai, L. Yang, X. Guo, O. Baslé and C.-J. Li, J. Am. Chem. Soc., 2010, 132, 12212 CrossRef CAS PubMed.
-
(a) T. Morimoto, K. Fuji and K. Tsutsumi, J. Am. Chem. Soc., 2002, 124, 3806 CrossRef CAS PubMed;
(b) T. Shibata, N. Toshidaa and K. Takagi, J. Org. Chem., 2002, 67, 7446 CrossRef CAS PubMed;
(c) K. Fuji, T. Morimoto and K. Tsutsumi, Angew. Chem., Int. Ed., 2003, 42, 2409 CrossRef CAS PubMed;
(d) J. H. Park, Y. Cho and Y. K. Chung, Angew. Chem., Int. Ed., 2010, 49, 5138 CrossRef CAS PubMed;
(e) F. Chahdoura, L. K. Fourmy, J. Durand, D. Madec and M. Gómez, Eur. J. Org. Chem., 2013, 29 Search PubMed;
(f) T. Furusawa, T. Morimoto, K. Ikeda, H. Tanimoto, Y. Nishiyama, K. Kakiuchi and N. Jeong, Tetrahedron, 2015, 71, 875 CrossRef CAS.
- For reviews, see:
(a) P. Mátyus, O. Eliás, P. Tapolcsányi, A. Polonka-Bálint and B. Halász-Dajka, Synthesis, 2006, 2625 CrossRef;
(b) M. C. Haibach and D. Seidel, Angew. Chem., Int. Ed., 2014, 53, 5010 CrossRef CAS PubMed;
(c) U. Wille, Chem. Rev., 2013, 113, 813 CrossRef CAS PubMed; For representative papers on radical hydride shift, see:
(d) E. Bosch and M. D. Bachi, J. Org. Chem., 1993, 58, 5581 CrossRef CAS;
(e) T. Hashimoto, D. Hirose and T. Taniguchi, Angew. Chem., Int. Ed., 2014, 53, 2730 CrossRef CAS PubMed;
(f) K. Wang, S. M. Villano and A. M. Dean, J. Phys. Chem. A, 2015, 119, 7205 CrossRef CAS PubMed;
(g) P. Yu, J.-S. Lin, L. Li, S.-C. Zheng, Y.-P. Xiong, L.-J. Zhao, B. Tan and X.-Y. Liu, Angew. Chem., Int. Ed., 2014, 53, 11890 CrossRef CAS PubMed;
(h) P. Yu, S.-C. Zheng, N.-Y. Yang, B. Tan and X.-Y. Liu, Angew. Chem., Int. Ed., 2015, 54, 4041 CrossRef CAS PubMed.
- For selected reviews and papers, see:
(a)
M. A. Lago, J. I. Luengo, C. E. Peishoff and J. D. Elliot, in Annual Reports in Medicinal Chemistry, ed. J. A. Bristol, Academic Press, San Diego, 1996, vol. 31, p. 81 Search PubMed;
(b)
S. F. Martin, in The Alkaloids, ed. A. R. Brossi, Academic Press, New York, 1987, vol. 30, pp. 251–376 Search PubMed;
(c) L. Ingrassia, F. Lefranc, J. Dewelle, L. Pottier, V. Mathieu, S. Spiegl-Kreinecker, S. Sauvage, M. E. Yazidi, M. Dehoux, W. Berger, E. Van Quaquebeke and R. Kiss, J. Med. Chem., 2009, 52, 1100 CrossRef CAS PubMed;
(d) J. P. Cueva, A. Gallardo-Godoy, J. I. Juncosa Jr, P. A. Vidi, M. A. Lill, V. J. Watts and D. E. Nichols, J. Med. Chem., 2011, 54, 5508 CrossRef CAS PubMed;
(e) K. C. Nicolaou, J. A. Pfefferkorn, A. J. Roecker, G.-Q. Cao, S. Barluenga and H. J. Mitchell, J. Am. Chem. Soc., 2000, 122, 9939 CrossRef CAS;
(f) C. Xue, V. R. R. Donuru and H. Liu, Macromolecules, 2006, 39, 5747 CrossRef CAS;
(g) G. Majetich and J. M. Shimkus, J. Nat. Prod., 2010, 73, 284 CrossRef CAS PubMed.
- For papers on the common radical cyclization of 1,n-enynes, see:
(a) I. Ryu, H. Miyazato, H. Kuriyama, K. Matsu, M. Tojino, T. Fukuyama, S. Minakata and M. Komatsu, J. Am. Chem. Soc., 2003, 125, 5632 CrossRef CAS PubMed;
(b) H. M. Ko, C. W. Lee, H. K. Kwon, H. S. Chung, S. Y. Choi, Y. K. Chung and E. Lee, Angew. Chem., Int. Ed., 2009, 48, 2364 CrossRef CAS PubMed;
(c) S. Mondal, B. Gold, R. K. Mohamed and I. V. Alabugin, Chem.–Eur. J., 2014, 20, 8664 CrossRef CAS PubMed;
(d) N. Fuentes, W. Kong, L. Fernández-Sánchez, E. Merino and C. Nevado, J. Am. Chem. Soc., 2015, 137, 964 CrossRef CAS PubMed;
(e) J.-Y. Luo, H.-L. Hua, Z.-S. Chen, Z.-Z. Zhou, Y.-F. Yang, P.-X. Zhou, Y.-T. He, X.-Y. Liu and Y.-M. Liang, Chem. Commun., 2014, 50, 1564 RSC.
- CCDC 1438591 (3ha) contains the supplementary crystallographic data for this paper.†.
Footnote |
† Electronic supplementary information (ESI) available. CCDC 1438591. For ESI and crystallographic data in CIF or other electronic format see DOI: 10.1039/c6sc02451c |
|
This journal is © The Royal Society of Chemistry 2016 |
Click here to see how this site uses Cookies. View our privacy policy here.