DOI:
10.1039/C6QO00393A
(Research Article)
Org. Chem. Front., 2016,
3, 1443-1446
Visible-light-induced three-component 1,2-difluoroalkylarylation of styrenes with α-carbonyl difluoroalkyl bromides and indoles†
Received
26th July 2016
, Accepted 29th August 2016
First published on 30th August 2016
Abstract
A novel visible light photoredox catalysis three-component 1,2-difluoroalkylarylation of styrenes was disclosed, and two new C–C bonds were generated in a single step through regioselective incorporation of a CF2 group and a variety of indoles to C
C bonds. The well-designed photoredox system achieved the synthesis of a series of difluoro-containing indole derivatives with mild conditions and a broad substrate scope.
Introduction
Organic compounds with the difluoromethene group (CF2) usually possess unique physical and chemical advantages which lead to the broad exploitation of this motif in the field of agrochemicals and pharmaceuticals.1 Additionally, it is well established that the CF2 group can act as a bioisostere for an oxygen atom or a carbonyl group, making it an interesting group with respect to the design of bioactive molecules or CF2-containing drugs.2 Therefore, the selective introduction of the CF2 moiety into diverse skeletons has become a hot topic in synthetic chemistry.3,4
Over the past few years, visible-light photoredox catalysis has been regarded as a powerful tool for the preparation of fluorinated compounds.5 Among the available methods, versatile fluoroalkylative difunctionalization of alkenes has been well-developed quite recently.6 In 2011, seminal studies by the Stephenson group reported an elegant visible-light-catalyzed difunctionalization of alkenes with a haloalkane (BrCF2CO2Et), providing a mild way for the halodifluoroalkylation of alkenes.7a,b Later, the groups of Dolbier, Akita and Zhu developed the amino-,7d,g aryl-,7e halo-,7f and oxy-7h difluoroalkylation of alkenes (Scheme 1) via trapping the corresponding oxidized electrophilic cation (or its corresponding radical intermediate). For the past two years, our group has also extensively developed a photoredox-catalyzed difluoroalkylative difunctionalization of alkenes to construct fluorinated ketones,7i oxindoles,7j and quinolone7j and polycyclic lactone7k scaffolds. Despite the excellent studies in the aforementioned difluoroalkylated difunctionalization research, most of them are limited to construct tandem C–CF2R and C–X (X
H, NR, OR, halogen) bonds or two-component intramolecular C(sp2)–H functionalization and cyclization. To the best of our knowledge, visible-light-induced three-component difluoroalkylation of styrenes allowing the simultaneous formation of two new C–C bonds in one step remains underdeveloped. Indole, the core structure in naturally and artificially available bioactive compounds, has received particular attention in the modification of this privileged structural motif.8 Thus, given our group established interest in the photochemistry of fluorinated radicals,7h–j,9 we envisaged that the appropriate choice of indole as a nucleophile could realize a novel photoredox-catalyzed difluoroalkylation of styrenes. Herein, we firstly reported the visible-light-promoted three-component 1,2-difluoroalkylarylation of styrene with α-carbonyl difluoroalkyl bromides as the CF2 radical precursor and indoles as nucleophiles. This method enables the regioselective incorporation of a CF2 group and a variety of indoles to C
C bonds by photoredox catalysis under mild conditions (Scheme 1).
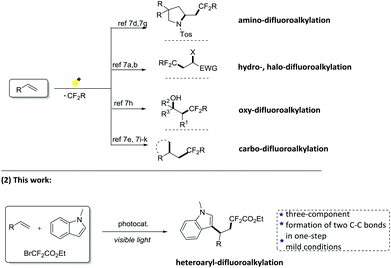 |
| Scheme 1 Photoredox-catalyzed difluoroalkylative difunctionalization of alkenes. | |
Results and discussion
In order to verify our hypothesis, we firstly commenced to use 1-methyl-1H-indole 1a with 1-methoxy-4-vinylbenzene 2a and ethyl 2-bromo-2,2-difluoroacetate 3a for model reaction to optimize the conditions (Table 1). Visible light irradiation (36 W household white LED lamp) of the reaction for 24 h led to the formation of the expected adduct 4a in 20% yield in the presence of 2 mol% of fac-Ir(ppy)3, and 2.0 equiv. of Ag2CO3 (Table 1, entry 1). To improve the yield, bases were first screened and AgOAc proved to be a more competent base (entries 2–5). Next, an evaluation of solvents revealed that DCM provided a significant improvement in yield (entry 7, 70%). The catalyst Ru(bpy)3Cl2 or Ir(ppy)2(dtbbpy)PF6 failed to improve the reaction yield (entries 11–12). Finally, the reaction could not proceed either in the dark or in the absence of fac-Ir(ppy)3 or base (entries 13–15), indicating that illumination, the photocatalyst and the base played key roles in this reaction system.
Table 1 Optimization of the reaction conditionsa
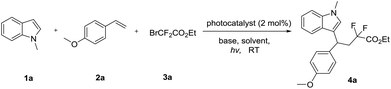
|
Entry |
Photocatalyst |
Base |
Solvent |
Yieldb [%] |
The reactions were carried out with 1a (0.2 mmol), 2a (0.4 mmol), 3a (0.4 mmol), base (0.4 mmol, 2 equiv.), photocatalyst (0.004 mmol, 2 mol%), solvent (2 mL), at room temperature, 36 W fluorescent light bulb, 36 h.
Yield of the isolated product based on the amount of 1a.
The reactions were carried out with 1a (0.2 mmol), 2a (0.3 mmol), and 3a (0.3 mmol).
The reactions were carried out with 1a (0.2 mmol), 2a (0.5 mmol), and 3a (0.5 mmol).
Without base.10
In the dark. bpy = bipyridine, ppy = phenylpyridine, dtbpy = 4,4′-di-tert-butyl-2,2′-bipyridine. DMF = N,N-dimethylformamide, DMSO = dimethylsulfoxide, DCM = dichloromethane.
|
1 |
fac-Ir(ppy)3 |
Ag2CO3 |
CH3CN |
20 |
2 |
fac-Ir(ppy)3 |
K2CO3 |
CH3CN |
53 |
3 |
fac-Ir(ppy)3 |
K2HPO4 |
CH3CN |
51 |
4 |
fac-Ir(ppy)3 |
KOAc |
CH3CN |
60 |
5 |
fac-Ir(ppy)3 |
AgOAc |
CH3CN |
66 |
6 |
fac-Ir(ppy)3 |
AgOAc |
DMF |
68 |
7 |
fac-Ir(ppy)3 |
AgOAc |
DCM |
70 |
8 |
fac-Ir(ppy)3 |
AgOAc |
DMSO |
40 |
9c |
fac-Ir(ppy)3 |
AgOAc |
DCM |
60 |
10d |
fac-Ir(ppy)3 |
AgOAc |
DCM |
65 |
11 |
Ru(bpy)3Cl2 |
AgOAc |
DCM |
Trace |
12 |
Ir(ppy)2(dtbbpy)PF6 |
AgOAc |
DCM |
56 |
13e |
fac-Ir(ppy)3 |
— |
DCM |
nd |
14 |
— |
AgOAc |
DCM |
nd |
15f |
fac-Ir(ppy)3 |
AgOAc |
DCM |
nd |
With the optimal reaction conditions in hand, the substrate scope of indole, styrene, and difluoro-reagent was investigated, and the representative examples are shown in Scheme 2. When testing the effect of the N-substituent indoles, we found that the electronic properties of the N-substituent group were crucial for this successful transformation. The indole, protected by an electron-rich group such as the benzyl, could convert to the difluoroalkylated product (4b) in moderate yield (68%), whereas N-Boc, N-Ts indoles failed to undergo the expected transformation under the standard conditions (4c–4d). It was worth mentioning that 5-methoxy-1H-indole, having a free NH group, remained viable in this protocol with 46% yield (4e). Thus the process could be applicable to the modifications of the N–H bond in the synthesis of complicated molecules. Next, a wide range of substituent indoles were amenable to this reaction. Various substituents in the indole scaffold, such as methoxy, methyl, halogen, or cyan groups, furnished the CF2-containing indoles (4f–4l) in good yields (52%–90%). Indole with a substituent at the 4-, 6- or 7-position smoothly produced the corresponding coupling products (4m–4p). Intriguingly, 2-substituted indoles also proceeded well to give the desired products (4q–4s) in 51%–68% yields, even for the bulky phenyl substituted substrate (2r). Nevertheless, no reaction occurred when a 3-substituted indole was used as the substrate, indicating that the tandem three-component reaction has excellent chemoselectivity at the C3 position of indoles. Moreover, we found the N-hetero indole 1-methyl-1H-pyrrolo[2,3-b]pyridine (1u) was compatible with this process.
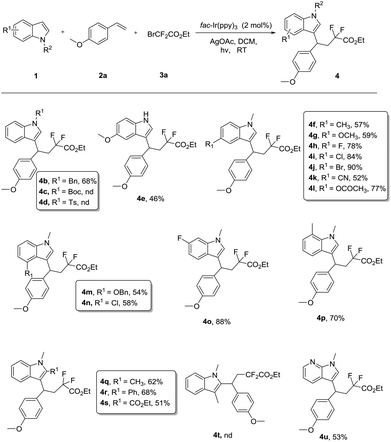 |
| Scheme 2 Scope of different substituent indoles. Reaction conditions: 1 (0.2 mmol), 2a (0.4 mmol, 2.0 equiv.), 3a (0.4 mmol, 2.0 equiv.), AgOAc (0.4 mmol, 2.0 equiv.), fac-[Ir(ppy)3] (0.004 mmol, 2 mol%), DCM (2 mL), at room temperature, 36 W LEDs, 48 h. | |
Next, we evaluated the scope of styrene, and the difluoro-reagent under the optimized reaction conditions. As illustrated in Scheme 3, different aryl alkenes were investigated. We found that the electronic nature of the aryl group had a fundamental influence on the reactivity. The electron-rich aryl alkene, bearing the o-CH3OC6H4 and p-MeC6H4 group, afforded the expected products (4v, 4w) with 75% and 58% yield, respectively. However, the alkene with an electron-withdrawing substituent group was not a suitable substrate (see the ESI†). To our delight, the internal alkenes 1,2-dihydronaphthalene provided the CF2-substituted products 4x in 65% yield. Subsequently, we set out to study difluorinated substrates. Delightfully, bromodifluoroketones and bromodifluoroacetamides as difluorinating reagents were well-tolerated, thus providing the corresponding products (4aa–4ac) with moderate to good yields (30%–80%).
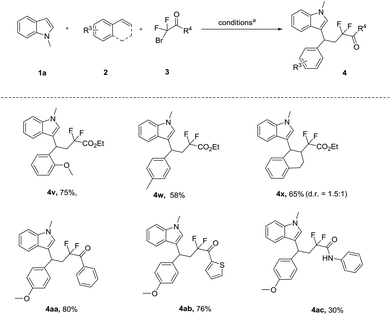 |
| Scheme 3 Variation of the scope of styrene 2 and difluoro-reagent 3. a See Table 1 and Scheme 2. | |
To gain an insight into the mechanism of this transformation, a radical-trapping experiment was carried out. Notably, the reaction was completely suppressed by the radical inhibitor TEMPO (2,2,6,6-tetramethylpiperidinooxy), thus implying that this current reaction involves a free radical process. Based on this result and previous reports,11 a plausible reaction mechanism via SET processes is shown in Scheme 4. Firstly, irradiation of iridium photocatalyst 9 with visible light will produce the long-lived excited state 10 (−1.73 V vs. SCE in CH3CN), which can undergo the SET process in the presence of 3a to generate the CF2 radical 5 and Ir4+11 (+0.77 V vs. SCE in CH3CN).12 Then, addition of ˙CF25 to alkene 2a gives the more stable benzyl radical intermediate 6, which is subsequently oxidized into the cation intermediate 7 by Ir4+11. Finally, the β-difluoroalkylated carbocation intermediate 7 is attacked by nucleophilic indoles 1a to afford cation intermediate 8, followed by base-mediated deprotonation to give the final selective difluoroalkylated product 4a.
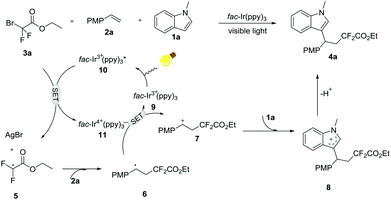 |
| Scheme 4 Plausible reaction mechanism. | |
Conclusions
In conclusion, we have successfully developed the visible-light-catalyzed three-component 1,2-difluoroalkylarylation of styrenes for the synthesis of a series of CF2-containing indole derivatives. This reaction allows a one-step regioselective construction of two C–C bonds with mild reaction conditions and a broad substrate scope. Given the prevalence of organofluorine compounds in medicinal agents, and life and materials sciences, we expect that this reaction will find immediate application in the synthesis of biologically active compounds.
Acknowledgements
We gratefully acknowledge the National Natural Science Foundation of China (21474048 and 21372114) for financial support.
Notes and references
-
(a) S. Purser, P. R. Moore, S. Swallow and V. Gouverneur, Chem. Soc. Rev., 2008, 37, 320 RSC;
(b) J. A. Erickson and J. I. McLoughlin, J. Org. Chem., 1995, 60, 1626 CrossRef CAS;
(c) R. Aráoz, E. Anhalt, L. René, M.-A. Badet-Denisot, P. Courvalin and B. Badet, Biochemistry, 2000, 39, 15971 CrossRef;
(d) V. D. Romanenko and V. P. Kukhar, Chem. Rev., 2006, 106, 3868 CrossRef CAS PubMed;
(e) K. Mîller, C. Faeh and F. Diederich, Science, 2007, 317, 1881 CrossRef PubMed;
(f)
J.-P. Begue and D. Bonnet-Delpon, Bioorganic and Medicinal Chemistry of Fluorine, Wiley, Hoboken, 2008 Search PubMed.
-
(a) C. M. Blackburn, D. A. England and F. Kolkmann, J. Chem. Soc., Chem. Commun., 1981, 930 RSC;
(b) G. M. Blackburn, D. E. Kent and F. Kolkmann, J. Chem. Soc., Perkin Trans. 1, 1984, 1119 RSC;
(c) J. A. Erickson and J. I. Mcloughlin, J. Org. Chem., 1995, 60, 1626 CrossRef CAS;
(d)
T. Kitazume and T. Kamazaki, Experimental Methods in Organic Fluorine Chemistry, Gordon and Breach Science, Tokyo, 1998 Search PubMed.
- For some reviews on difluoroalkylation, see:
(a) T. Liang, C. N. Neumann and T. Ritter, Angew. Chem., Int. Ed., 2013, 52, 8214 CrossRef CAS PubMed;
(b) C. Ni, M. Hu and J. Hu, Chem. Rev., 2015, 115, 765 CrossRef CAS PubMed;
(c) W. Zhang, F. Wang and J. Hu, Chem. Commun., 2009, 7465 Search PubMed;
(d) C. Zhang, Q. Chen, Y. Guo, J. Xiao and Y. Gu, Chem. Soc. Rev., 2012, 41, 4536 RSC;
(e) E. J. Cho, Chem. Rec., 2016, 16, 47 CrossRef CAS PubMed.
- For examples of difluoroalkylation reactions:
(a) Z. Feng, Q.-Q. Min, Y.-L. Xiao, B. Zhang and X. Zhang, Angew. Chem., Int. Ed., 2014, 53, 1669 CrossRef CAS PubMed;
(b) Y. Xiao, W. Guo, G. He, Q. Pan and X. Zhang, Angew. Chem., Int. Ed., 2014, 53, 9909 CrossRef CAS PubMed;
(c) Y. Yu, G. He and X. Zhang, Angew. Chem., Int. Ed., 2014, 53, 10457 CrossRef CAS PubMed;
(d) S.-L. Shi and S. L. Buchwald, Angew. Chem., Int. Ed., 2015, 54, 1646 CrossRef CAS PubMed;
(e) G. Li, T. Wang, F. Fei, Y.-M. Su, Y. Li, Q. Lan and X.-S. Wang, Angew. Chem., Int. Ed., 2016, 55, 1 CrossRef PubMed;
(f) A. Prieto, R. Melot, D. Bouyssi and N. Monteiro, ACS Catal., 2016, 6, 1093 CrossRef CAS;
(g) A. Prieto, R. Melot, D. Bouyssi and N. Monteiro, Angew. Chem., Int. Ed., 2016, 55, 1885 CrossRef CAS PubMed;
(h) J. Xie, T. Zhang, F. Chen, N. Mehrkens, F. Rominger, M. Rudolph and A. S. K. Hashmi, Angew. Chem., Int. Ed., 2016, 55, 2934 CrossRef CAS PubMed.
- For examples of photoredox catalysis fluoro-containing radical reactions:
(a) X.-J. Wei, D.-T. Yang, L. Wang, T. Song, L.-Z. Wu and Q. Liu, Org. Lett., 2013, 15, 6054 CrossRef CAS PubMed;
(b) X. Sun and S. Yu, Org. Lett., 2014, 16, 2938 CrossRef CAS PubMed;
(c) L. Wang, X.-J. Wei, W.-L. Jia, J.-J. Zhong, L.-Z. Wu and Q. Liu, Org. Lett., 2014, 16, 5842 CrossRef CAS PubMed;
(d) J. Jung, E. Kim, Y. You and E. J. Cho, Adv. Synth. Catal., 2014, 356, 2741 CrossRef CAS;
(e) C. Yu, N. Iqbal, S. Park and E. J. Cho, Chem. Commun., 2014, 50, 12884 RSC;
(f) Q. Wei, J.-R. Chen, X.-Q. Hu, X.-C. Yang, B. Lu and W.-J. Xiao, Org. Lett., 2015, 17, 4464 CrossRef CAS PubMed;
(g) B. Sahoo, J.-L. Li and F. Glorius, Angew. Chem., Int. Ed., 2015, 54, 11577 CrossRef CAS PubMed;
(h) J. Rong, L. Deng, P. Tan, C. Ni, Y. Gu; and J. Hu, Angew. Chem., Int. Ed., 2016, 55, 2743 CrossRef CAS PubMed;
(i) P. Gao, X.-R. Song, X.-Y. Liu and Y.-M. Liang, Chem. – Eur. J., 2015, 21, 7648 CrossRef CAS PubMed.
- For examples of the fluoroalkylative difunctionalization of alkenes:
(a) X. Pan, H. Xia and J. Wu, Org. Chem. Front., 2016, 1163 RSC;
(b) T. Koike and M. Akita, Org. Chem. Front., 2016 10.1039/C6QO00139D;
(c) T. Courant and G. Masson, J. Org. Chem., 2016, 6945 CrossRef CAS PubMed;
(d) E. J. Cho, Chem. Rec., 2016, 16, 47 CrossRef CAS PubMed;
(e) S. Barata-Vallejo, S. M. Bonesi and A. Postigo, Org. Biomol. Chem., 2015, 13, 11153 RSC;
(f) M.-Y. Cao, X. Ren and Z. Lu, Tetrahedron Lett., 2015, 56, 3732 CrossRef CAS;
(g) B. Li, D. Fan, C. Yang and W. Xia, Org. Biomol. Chem., 2016, 14, 5293 RSC;
(h) H. Egami and M. Sodeoka, Angew. Chem., Int. Ed., 2014, 53, 8294 CrossRef CAS PubMed;
(i) E. Merino and C. Nevado, Chem. Soc. Rev., 2014, 43, 6598 RSC;
(j) C. Alonso, E. M. De Marigorta, G. Rubiales and F. Palacios, Chem. Rev., 2015, 115, 1847 CrossRef CAS PubMed.
-
(a) J. D. Nguyen, J. W. Tucker, M. D. Konieczynska and C. R. J. Stephenson, J. Am. Chem. Soc., 2011, 133, 4160 CrossRef CAS PubMed;
(b) C. J. Wallentin, J. D. Nguyen, P. Finkbeiner and C. R. J. Stephenson, J. Am. Chem. Soc., 2012, 134, 8875 CrossRef CAS PubMed;
(c) Q.-Y. Lin, X.-H. Xu, K. Zhang and F.-L. Qing, Angew. Chem., Int. Ed., 2016, 55, 1479 CrossRef CAS PubMed;
(d) Z. Zhang, X. Tang, C. S. Thomoson and W. R. Dolbier Jr., Org. Lett., 2015, 17, 3528 CrossRef CAS PubMed;
(e) X.-J. Tang, C. S. Thomoson and W. R. Dolbier Jr., Org. Lett., 2014, 16, 4594 CrossRef CAS PubMed;
(f) X.-J. Tang and W. R. Dolbier Jr., Angew. Chem., Int. Ed., 2015, 54, 4246 CrossRef CAS PubMed;
(g) M. Zhang, W. Li, Y. Duan, P. Xu, S. Zhang and C. Zhu, Org. Lett., 2016, 18, 3266 CrossRef CAS PubMed;
(h) Y. Arai, R. Tomita, G. Ando, T. Koike and M. Akita, Chem. – Eur. J., 2016, 22, 1262 CrossRef CAS PubMed;
(i) P. Xu, K. Hu, Z. Gu, Y. Cheng and C. Zhu, Chem. Commun., 2015, 51, 7222 RSC;
(j) Z. Gu, H. Zhang, P. Xu, Y. Cheng and C. Zhu, Adv. Synth. Catal., 2015, 357, 3057 CrossRef CAS;
(k) C. Qu, P. Xu, W. Ma, Y. Cheng and C. Zhu, Chem. Commun., 2015, 51, 13508 RSC.
-
(a) A. T. Balaban, D. C. Oniciu and A. R. Katritzky, Chem. Rev., 2004, 104, 2777 CrossRef CAS PubMed;
(b) I. V. Seregin and V. Gevorgyan, Chem. Soc. Rev., 2007, 36, 1173 RSC;
(c) D. M. D'Souza and T. J. J. Müller, Chem. Soc. Rev., 2007, 36, 1095 RSC.
-
(a) P. Xu, J. Xie, Q. Xue, C. Pan, Y. Cheng and C. Zhu, Chem. – Eur. J., 2013, 19, 14039 CrossRef CAS PubMed;
(b) P. Xu, A. Abdukader, K. Hu, Y. Cheng and C. Zhu, Chem. Commun., 2014, 50, 2308 RSC;
(c) P. Xu, G. Wang, Y. Zhu, W. Li, Y. Cheng, S. Li and C. Zhu, Angew. Chem., Int. Ed., 2016, 55, 2939 CrossRef CAS PubMed.
- The aromatic C–H difluoroalkylation product and alkene C–H difluoroalkylation product were detected from the reaction without a base, as determined by 1H NMR analysis of the crude reaction mixture (see the ESI†).
-
(a) Y. Yasu, T. Koike and M. Akita, Angew. Chem., Int. Ed., 2012, 51, 9567 CrossRef CAS PubMed;
(b) Y. Yasu, T. Koike and M. Akita, Org. Lett., 2013, 15, 2136 CrossRef CAS PubMed;
(c) A. Carboni, G. Dagousset, E. Magnier and G. Masson, Org. Lett., 2014, 16, 1240 CrossRef CAS PubMed;
(d) A. Carboni, G. Dagousset, E. Magnierb and G. Masson, Chem. Commun., 2014, 50, 14197 RSC;
(e) X.-H. Ouyang, R.-J. Song, M. Hu, Y. Yang and J.-H. Li, Angew. Chem., Int. Ed., 2016, 55, 3187 CrossRef CAS PubMed.
- C. K. Prier, D. A. Rankic and D. W. C. MacMillan, Chem. Rev., 2013, 113, 5322 CrossRef CAS PubMed.
Footnote |
† Electronic supplementary information (ESI) available. See DOI: 10.1039/c6qo00393a |
|
This journal is © the Partner Organisations 2016 |